Light-dependent single-cell heterogeneity in the chloroplast redox state regulates cell fate in a marine diatom
Figures
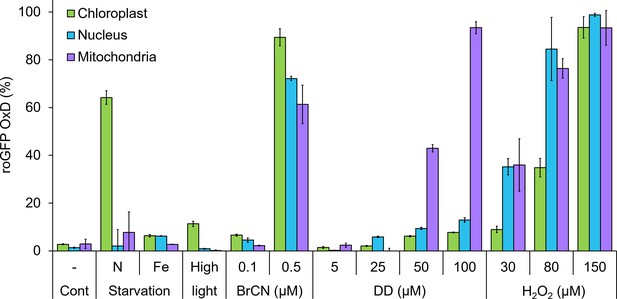
Organelle-specific oxidation in response to environmental stressors in P. tricornutum.
Maximal roGFP degree of oxidation (OxD) measured in the chloroplast, nucleus and mitochondria in response to various environmental stress conditions based on bulk measurements using flow cytometry, data from previously published works. Cont – control untreated cells. N starvation - nitrate limitation 56 hr (See Rosenwasser et al., 2014). Fe starvation - iron limitation, day 3 (see Graff van Creveld et al., 2015; Graff van Creveld et al., 2016). High light, 700 µmol photons·m−2·sec−1, 38 min (see Graff van Creveld et al., 2015). BrCN - cyanogen bromide, 0.1 and 0.5 µM, 10 min (see Graff van Creveld et al., 2015). DD - 2E,4E/Z-decadienal (diatom-derived unsaturated aldehyde), 5, 25, 50 and 100 µM, 3 hr (see Graff van Creveld et al., 2015). H2O2 – 30, 80, and 150 µM, ~30 min (see Graff van Creveld et al., 2015). Bars represent average of biological triplicates, error bars are standard error of the mean.
-
Figure 1—source data 1
Organelle-specific oxidation in response to environmental stressors in P. tricornutum.
- https://doi.org/10.7554/eLife.47732.004
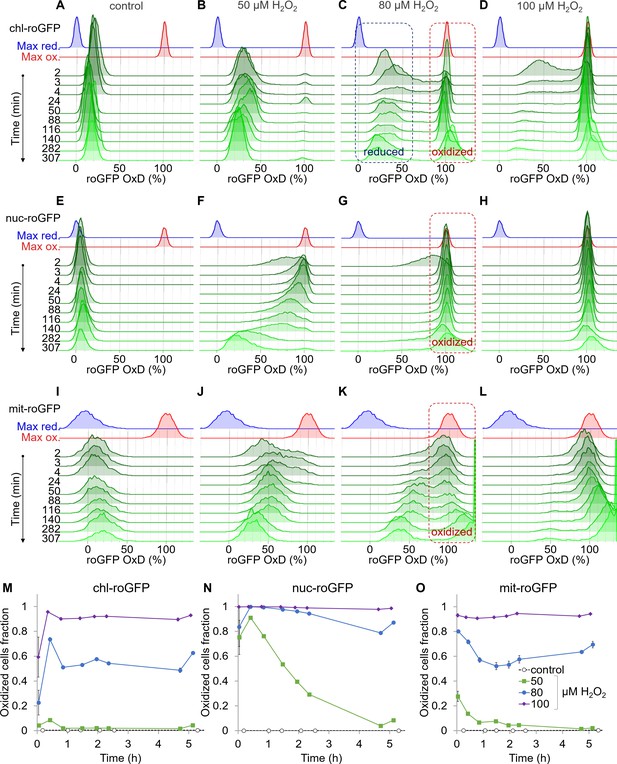
Organelle-specific oxidation of roGFP in response to H2O2 reveals heterogeneity at the single-cell level.
The distribution of roGFP OxD in the population over time was measured by flow cytometry in P. tricornutum cells expressing roGFP targeted to the chloroplast (chl-roGFP, (A–D, M)), nucleus (nuc-roGFP, (E–H, N)) and mitochondria (mit-roGFP, (I–L, O)). (A–L) Oxidation of roGFP in response to 0 µM (A,E,I), 50 µM (B,F,J), 80 µM (C,G,K), and 100 µM (D,H,L) H2O2. Maximum reduction (blue) and oxidation (red) of roGFP following additions of 2 mM Dithiothreitol (DTT) or 200 µM H2O2 respectively are shown as a reference. The ‘oxidized’ and ‘reduced’ subpopulations are marked by red and blue dashed boxes respectively (C, G, K). The experiment was done in triplicates, for visualization the first replica is shown except for the first 4 min in which all replicates are shown for higher temporal resolution. Each histogram consists of >8000 (A–D), >5900 (E–H) and >1400 (I–L) roGFP-positive cells. Measurements of >100% OxD may result from increased auto-fluorescence leakage after long exposure to stress (Figure 2—figure supplements 5–6). (M–O) The fraction of the ‘oxidized’ subpopulation over time upon exposure to 0–100 µM H2O2. Mean ± SEM, n = 3 biological repeats. SEM lower than 0.018 are not shown.
-
Figure 2—source data 1
Flow cytometry measurements of organelle-specific oxidation of roGFP in response to H2O2 over time.
- https://doi.org/10.7554/eLife.47732.012
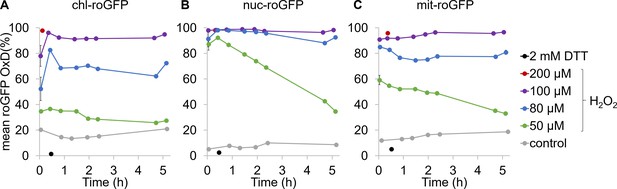
Organelle specific roGFP mean oxidation in response to H2O2 application in P. tricornutum.
Flow cytometry measurements of the mean ± SEM roGFP OxD in the population over time in response to oxidative stress in chl-roGFP (A), nuc-roGFP (B) and mit-roGFP (C) P. tricornutum strains, n = 3 biological repeats. Oxidation dynamics were measured following treatments with different H2O2 concentrations: 50 µM (green), 80 µM (blue), 100 µM (purple) and without treatment (control, gray). Maximum reduction (2 mM DTT, black) and maximum oxidation (200 µM H2O2, red) are shown for reference. Error bars below 1.5% are not shown.
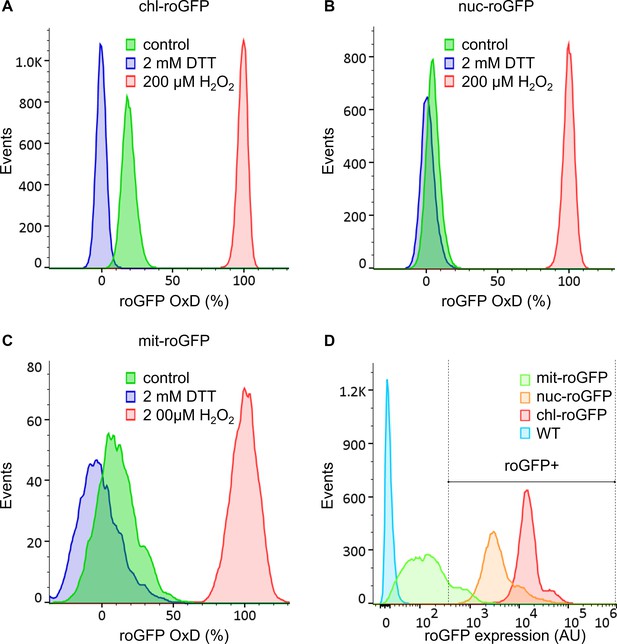
Distribution of roGFP oxidation in different subcellular compartments of P. tricornutum cells at steady state.
Flow cytometry measurements of roGFP OxD and expression levels in P. tricornutum cells expressing roGFP targeted to the chloroplast (chl-roGFP, (A)), nucleus (nuc-roGFP, (B)) and mitochondria (mit-roGFP, (C)). (A–C) The distribution of roGFP OxD in the population at steady state conditions (without treatment, green) and following treatments of maximum oxidation (200 µM H2O2, red) and maximum reduction (2 mM DTT, blue). (D) Relative roGFP expression levels (AU) based on fluorescence intensity in chl-roGFP (red), nuc-roGFP (orange), mit-roGFP (green) and WT (no roGFP, cyan) strains. Relative roGFP expression was calculated by multiplication of the i405 and i488 (see Materials and methods). A threshold was set to exclude cells with low roGFP expression from roGFP oxidation calculations, shown as a gate with the label ‘roGFP+’. Each histogram consists of >12,000 cells (A, B, D) or >2,400 cells (C, due to lower roGFP expression). The experiment was done in triplicates, for visualization purposes one representative repeat is shown.
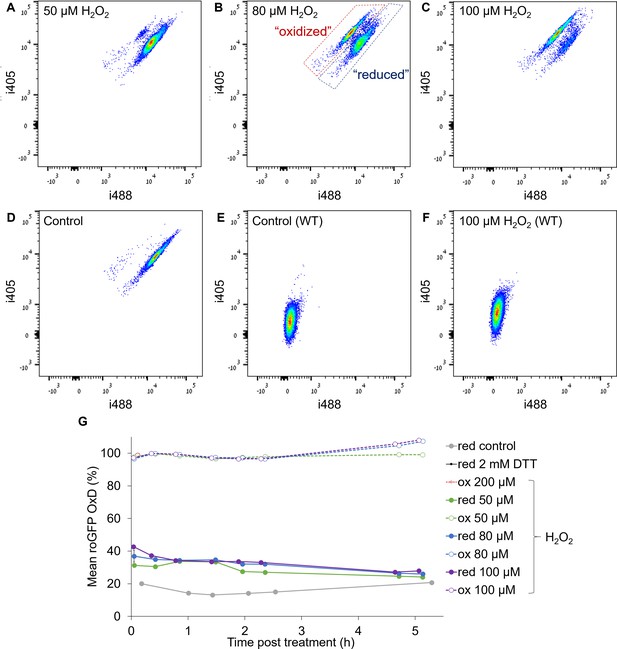
Oxidation of chl-roGFP reveals distinct subpopulations in response to H2O2 treatment.
(A–F) Density plots of flow cytometry fluorescence measurements of i405 over i488 (see Materials and methods) of chl-roGFP (A-D, roGFP positive cells) and WT cells that do not express roGFP (E-F, chlorophyll positive cells) 83–88 min post H2O2 treatments of 50 µM (A), 80 µM (B), 100 µM (C, F) and untreated control (D, E). The ratio i405/i488 increases upon roGFP oxidation and is used for calculating roGFP OxD (see Materials and methods). The ‘oxidized’ and ‘reduced’ chl-roGFP subpopulations are marked in red and blue respectively in B. (G) Flow cytometry measurements of mean roGFP OxD of the ‘oxidized’ (dashed, empty symbols) and ‘reduced’ (solid, full symbols) subpopulations over time post 50–200 µM H2O2 treatments and in untreated control (gray). Maximum reduction following 2 mM DTT (black) is shown for reference. Results are shown as mean ± SEM, n = 3 biological repeats.
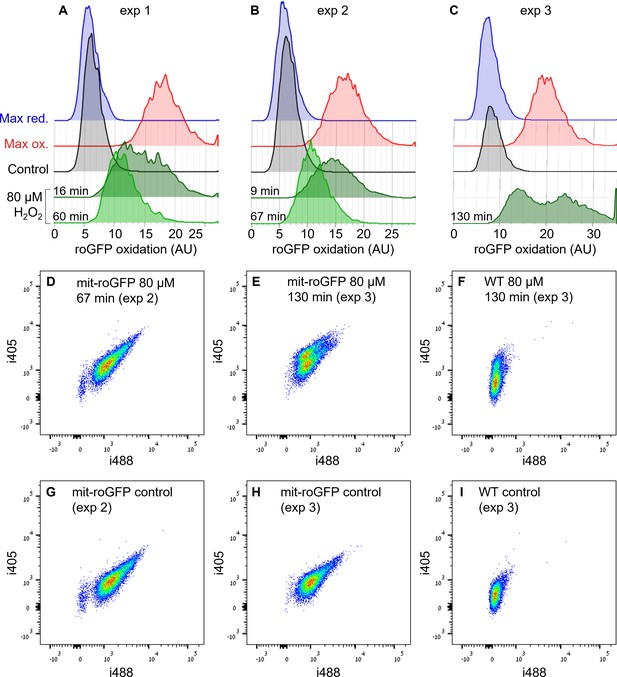
Heterogeneity in mit-roGFP response to H2O2.
Flow cytometry measurements of mit-roGFP oxidation in response to 80 µM H2O2 in three independent experiments (in addition to the experiment shown in Figure 2): exp 1 (A), exp 2 (B, D, G) and exp 3 (C, E, F, H, I). (A–C) Distribution of mit-roGFP OxD at different times post 80 µM H2O2 (green). Measurements of untreated control (black) and following maximum oxidation (red, 200 µM H2O2) and maximum reduction (blue, 2 mM DTT) are shown for reference. (D–I) Density plots of roGFP fluorescence measurements of i405 vs. i488 of mit-roGFP (D, E, G, H) and WT (F, I) strains at different times post 80 µM H2O2 treatment (D–F) or untreated control (G–I). The ratio i405/i488 represents roGFP oxidation state (see Materials and methods). WT is shown for auto-fluorescence (AF) leakage reference, demonstrating the effect of lower expression level in mit-roGFP strain. A differential response was observed in exp 3 and in the exp shown in Figure 2K,L, but in exp 1 and 2 no distinct subpopulations were observed. This differential response was clearly observed only at later time points, which were not measured in exp 1 and 2, and may result from auto-fluorescecne leakage (see Figure 2—figure supplements 5–6). Measurements were done in triplicates, one repeat is shown for visualization.
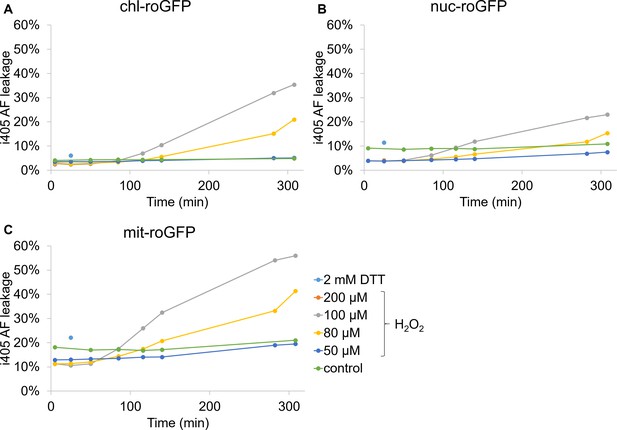
Auto-fluorescence leakage into i405 channel in roGFP strains following H2O2 treatment.
Flow cytometry measurements of i405 auto-fluorescence leakage in P. tricornutum strains expressing roGFP targeted to the chloroplast (chl-roGFP, (A)), nucleus (nuc-roGFP, (B)) and mitochondria (mit-roGFP, (C)) over time following treatments of 0–200 µM H2O2 or 2 mM DTT. Leakage into i405 channel was calculated by the mean i405 of the WT strain (n = 3 biological repeats) divided by the mean i405 of the roGFP expressing strain (n = 3 biological repeats) at the same time-point following the same treatment.
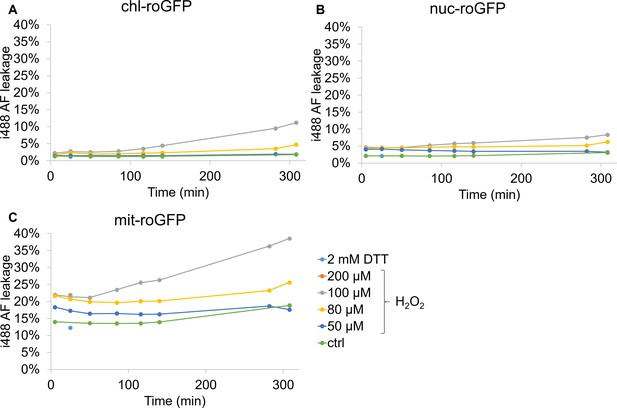
Auto-fluorescence leakage into i488 channel in roGFP strains following H2O2 treatment.
Flow cytometry measurements of i488 auto-fluorescence leakage in P. tricornutum strains expressing roGFP targeted to the chloroplast (chl-roGFP, (A)), nucleus (nuc-roGFP, (B)) and mitochondria (mit-roGFP, (C)) over time following treatments of 0–200 µM H2O2 or 2 mM DTT. Leakage into i488 channel was calculated by the mean i488 of the WT strain (n = 3 biological repeats) divided by the mean i488 of the roGFP expressing strain (n = 3 biological repeats) at the same time-point following the same treatment.
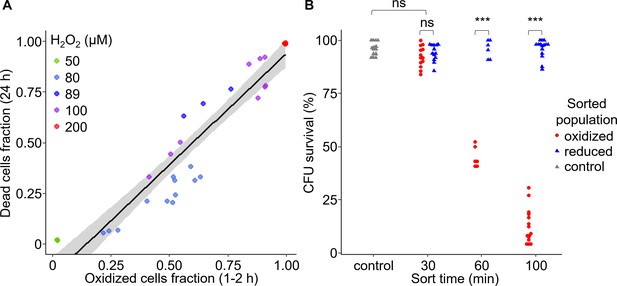
Early oxidation of chl-roGFP in a subpopulation precedes the induction of cell death.
(A) The fraction of dead cells measured by Sytox positive staining 24 hr post 50–200 H2O2 treatment as a function of the fraction of chl-roGFP ‘oxidized’ cells 1–2 hr post treatment (see Figure 2C). N ≥ 3 biological repeats per treatment, individual samples are shown, data of 5 independent experiments. Linear model is shown with 95% confidence interval in gray, formula: y = 1.09x-0.16, R2 = 0.89. (B) Colony forming units (CFU) survival of individual cells that were sorted into fresh media for regrowth based on their chl-roGFP oxidation at different times following treatment with 80 µM H2O2. Control – untreated chl-roGFP positive cells that were sorted regardless of degree of oxidation. CFU % survival was measured by the number of CFU divided by the number of sorted cells. N ≥ 6 biological repeats, individual repeats are shown, each of 24–48 separately sorted single cells. Oxidized – red circles; reduced – blue triangles; control – gray triangles. *** – p<0.001; ns – non-significant.
-
Figure 3—source data 1
Early chl-roGFP oxidation and subsequent cell death in response to H2O2.
- https://doi.org/10.7554/eLife.47732.016
-
Figure 3—source data 2
Colony forming units single-cell survival of subpopulations following H2O2 treatment and sorting.
- https://doi.org/10.7554/eLife.47732.017
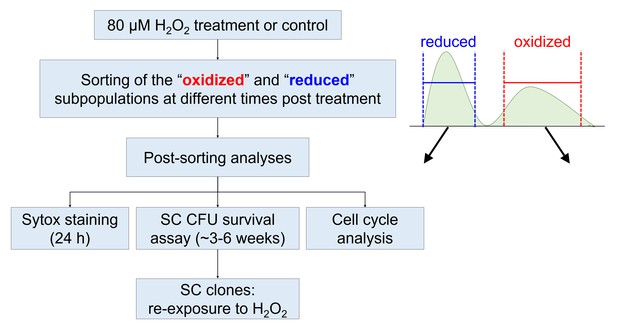
Schematic representation of sorting experiments layout and post-sorting analyses.
Cells were treated with 80 µM H2O2, and then sorted based on chl-roGFP oxidation for different post-sorting analyses. Untreated control chl-roGFP positive cells were sorted regardless of degree of oxidation. SC – single-cell. CFU – colony forming units.
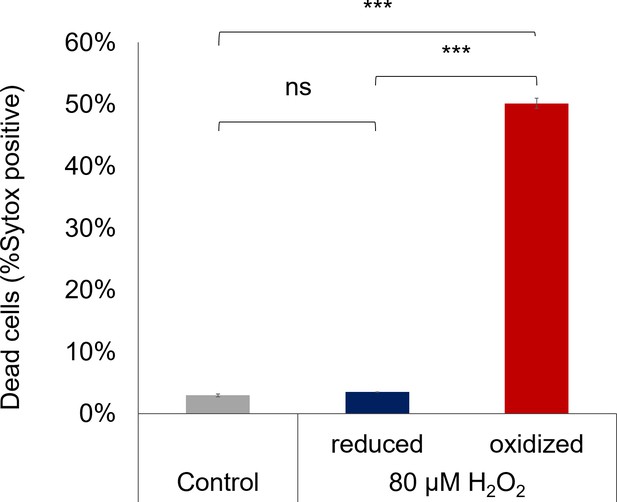
Mortality of sorted subpopulations following H2O2 treatment.
Cell death was measured using Sytox staining 24 hr post sorting of enriched ‘oxidized’ and ‘reduced’ subpopulations or untreated control (see Materials and methods). Subpopulations were sorted 112 min post 80 µM H2O2 treatment. Data is shown as mean ± SEM, n = 3 biological repeats, each consists of >3700 cells. (***) p<0.001; (ns) non-significant (p=0.73), Tukey test.
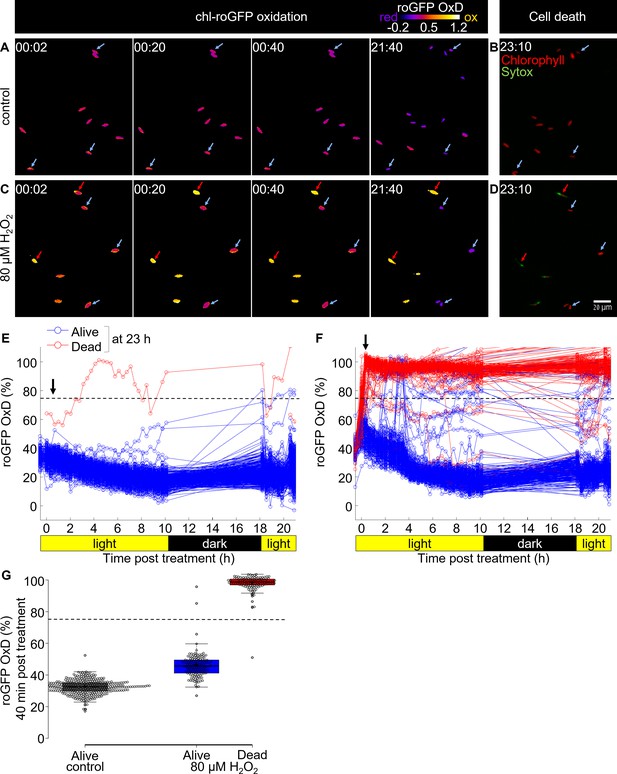
Tracking redox dynamics of individual cells using long term in vivo imaging in a microfluidics setup.
Oxidation of chl-roGFP was imaged over time using a customized microfluidic setup and epifluorescence microscopy. Cells were imaged following treatment with either fresh media (control; A, B, E) or 80 µM H2O2 (C, D, F). To quantify cell death, cells were stained with Sytox ~23 hr post treatment. (A, C) Representative frames depicted in pseudo-color of calculated roGFP OxD at different times post treatment (hh:mm). Two subpopulations of ‘oxidized’ (red arrows) and ‘reduced’ (blue arrows) cells were detected in treated cells. (B, D) Overlay of Sytox staining (green, dead cells) and chlorophyll auto-fluorescence (red) at 23:10 hr post treatment. (E, F) Quantification of chl-roGFP OxD per cell over time post treatment. Color is based on cell fate as measured ~23 hr post treatment: blue – alive (Sytox negative), red – dead (Sytox positive). OxD values > 110% likely result from auto-fluorescence leakage, and are not shown. (G) Box-plot of chl-roGFP OxD 40 min post treatment (arrow in E, F) of cells that were grouped based on their cell fate as measured ~23 hr post treatment. Gray – control alive; blue – 80 µM H2O2 alive; red – 80 µM H2O2 dead. Circles - single cells; cross – mean; colored box – 1st to 3rd quartiles; horizontal line within the box – median. Individual cells are shown. (E–G) N ≥ 250 cells per treatment from ≥3 different fields of view. Horizontal dashed line represents the chl-roGFP oxidation threshold used for discriminating cells that subsequently died or survived.
-
Figure 4—source data 1
Early chl-roGFP OxD and subsequent cell fate of single cells in response to H2O2 in a microfluidics setup.
- https://doi.org/10.7554/eLife.47732.023
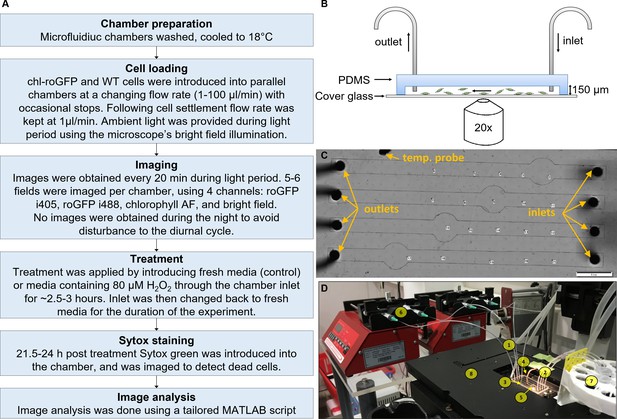
Microfluidics experimental layout.
(A) Schematic representation of microfluidics experiments. (B) Side view of the microfluidics chip design. PDMS - Polydimethylsiloxane. (C) Bright field image of the microfluidics chip (4x magnification) with markings of example imaging positions (dots), temperature probe, inlets and outlets. Scale bar = 5 mm. (D) The microfluidics experimental setup. 1: outlet tubes; 2: inlet tubes; 3: microfluidics chamber; 4: temperature electrode; 5: microscope objective; 6: syringe pumps; 7: inlet reservoirs; 8: motorized stage with controlled temperature.
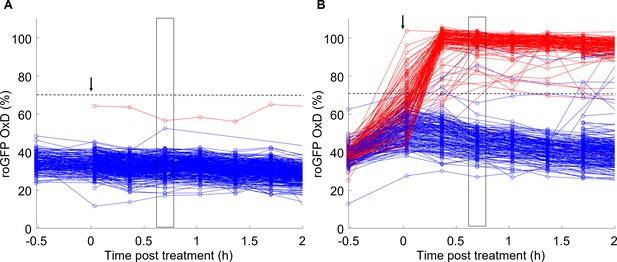
Tracking early chl-roGFP oxidation dynamics in single cells following oxidative stress using in vivo imaging in a microfluidics setup.
OxD of chl-roGFP per cell over time following treatment with either fresh media (control; A) or 80 µM H2O2 (B) of cells imaged over time using microfluidics and epifluorescence microscopy. Treatment was introduced at time 0 (arrow). Color is based on subsequent cell fate as measured by Sytox staining ~23 hr post treatment: blue – alive, red – dead. N ≥ 250 cells from at least three different fields. Horizontal dashed line represents the chl-roGFP oxidation threshold used for discriminating cells that subsequently died or survived. The black box marks the time point used for cell fate discrimination analysis in Figure 4G and Figure 4—figure supplement 3.
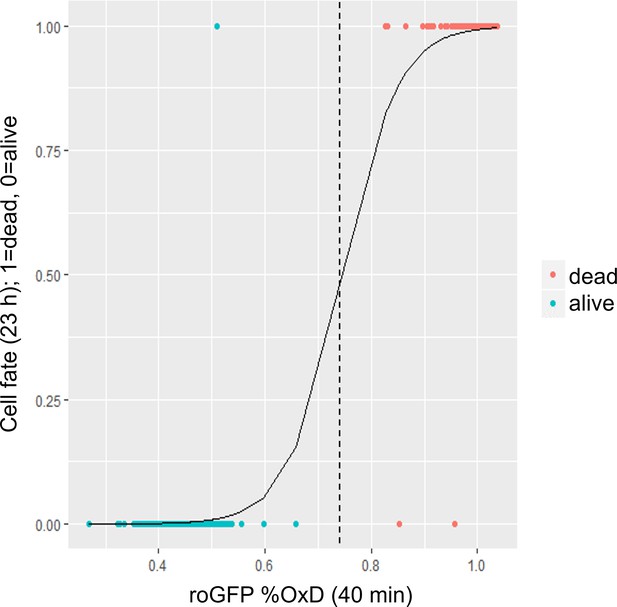
Logistic regression analysis of cell fate vs. chl-roGFP oxidation.
Cell fate (1 = dead, 0 = alive) as measured ~23 hr post 80 µM H2O2 treatment using Sytox staining vs. chl-roGFP OxD 40 min post treatment. Each dot is data from a single cell, n = 252 cells. Logistic regression is shown as a solid line. A threshold of OxD = 74% was used for discriminating cells that subsequently died (red) or survived (cyan), and is shown as a dashed line.
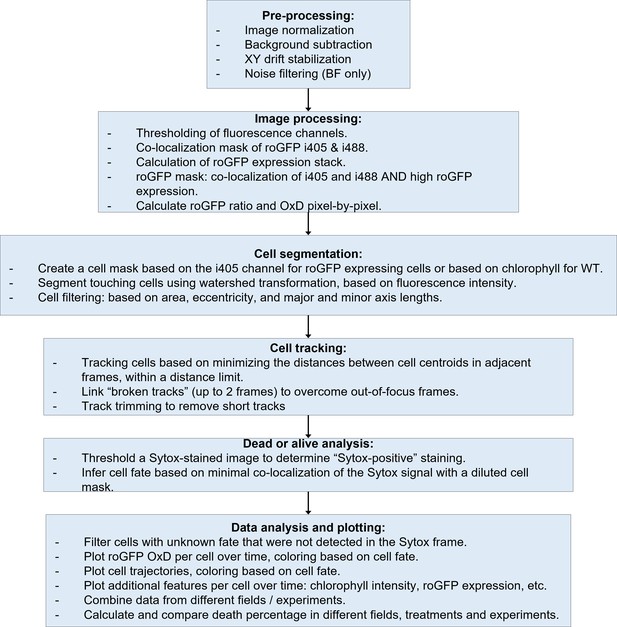
Schematic representation of the microfluidics image analysis pipeline.
https://doi.org/10.7554/eLife.47732.022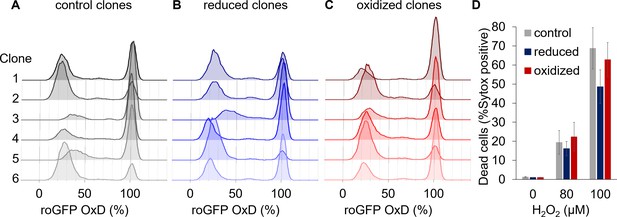
Clonal populations derived from sorted cells maintain the bi-stable phenotype of chl-roGFP response to H2O2.
(A–C) The distribution of chl-roGFP OxD (%) 40–45 min post 80 µM H2O2 treatment in clonal populations derived from sorted single cells of different origins, 3 weeks post sorting. The ‘reduced’ (B) and ‘oxidized’ (C) subpopulations were sorted 30 min post 80 µM H2O2 treatment based on chl-roGFP oxidation (Figure 3—figure supplement 1); ‘control’ (A) – clones of untreated cells that were sorted based on positive roGFP fluorescence. Each histogram is of a single clone,≥9900 cells per histogram, six representative clones per group are shown. (D) The fraction of dead cells 24 hr post H2O2 treatment of the different clones shown in (A–C) as measured by positive Sytox staining. Data is shown as mean ± SEM, n = 6 clones per group per treatment.
-
Figure 5—source data 1
The fraction of dead cells post H2O2 treatment of clones originating from different subpopulations.
- https://doi.org/10.7554/eLife.47732.028
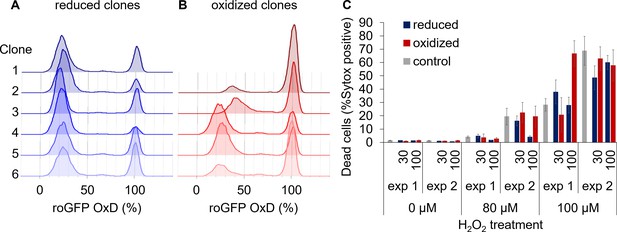
Sorted clonal populations maintain the bi-stable phenotype in chl-roGFP response to H2O2.
(A–B) The distribution of chl-roGFP OxD 40–45 min post re-exposure to 80 µM H2O2 treatment in clonal populations derived from sorted single cells of different origins, 3 weeks post sorting. The ‘reduced’ (A) and ‘oxidized’ (B) subpopulations were sorted 100 min post 80 µM H2O2 treatment based on chl-roGFP oxidation. Each histogram is of a single clone,≥9900 cells per histogram, six representative clones per group are shown. The same phenomenon was observed in two independent experiments in all examined clones (≥18 clones per group), for visualization data from one is shown. (C) The fraction of dead cells 24 hr post H2O2 treatment of clones originating from single cells sorted 30 and 100 min post H2O2 treatment or untreated control, as measured by positive Sytox staining. Data is shown as mean ± SEM, n ≥ 5 per column. Data of 2 independent experiments.
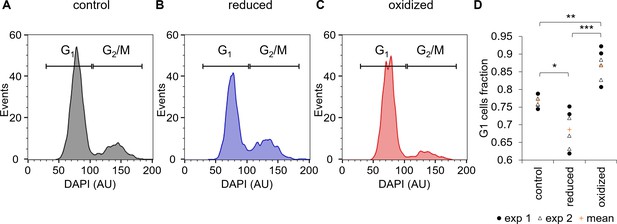
Cell cycle analysis of sorted ‘reduced’ and ‘oxidized’ subpopulations.
(A–C) Cell cycle analysis of FACS sorted control untreated cells (A) and of ‘reduced’ (B) and ‘oxidized’ (C) subpopulations that were sorted 30 min post 80 µM H2O2 treatment, based on chl-roGFP OxD. DAPI staining was used for DNA content quantification, gates used to discriminate G1 (two genome copies) and G2/M (four copies) are marked. (D) Fraction of cells within the G1 gate (marked in A-C) in sorted subpopulations. Data points of n = 6 biological repeats of 2 independent experiments (exp 1 and 2 marked with circles and triangles respectively) and the mean (orange plus sign) are shown, 1200–2000 cells analyzed per sample. P-values: *=0.011, **=0.0024, ***=0.0001. Tukey test was used for comparisons with control, paired t-test was used for comparing ‘reduced’ and ‘oxidized’ subpopulations.
-
Figure 6—source data 1
Cell cycle analysis of sorted subpopulations.
- https://doi.org/10.7554/eLife.47732.030
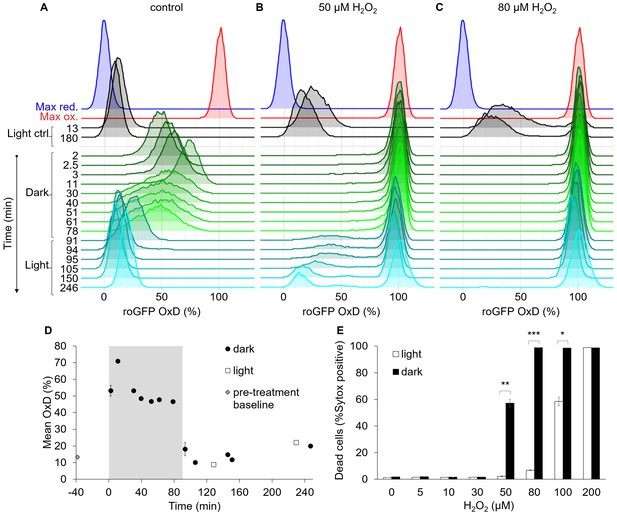
The bimodal chl-roGFP oxidation in response to H2O2 is light-dependent.
The effects of a short exposure to darkness during daytime on chl-roGFP oxidation patterns were examined. (A–C) Flow cytometry measurements of chl-roGFP OxD distribution in the population over time. Cells were treated with 0 µM (control, A), 50 µM (B), and 80 µM H2O2 (C), and were then transitioned to the dark at time 0 (within 5 min post H2O2 treatment). Cells were kept in the dark for 90 min (green) and were then transferred back to the light (cyan). The same H2O2 treatment without transition to the dark (light ctrl, black) and maximum oxidation (200 µM H2O2, red) and reduction (2 mM DTT, blue) are shown for reference. The experiment was done in triplicates that were highly similar, for visualization the first replica is shown. Each histogram consists of >8000 cells. (D) Mean ± SEM basal (control) chl-roGFP OxD over time of cells transitioned to the dark for 90 min (gray box) at time 0 (‘dark’) and cells kept in light conditions (‘light’), n = 3 biological repeats. Pre-treatment baseline under light conditions is shown. SEM lower than 0.5% are not shown. (E) The fraction of dead cells 24 hr post H2O2 treatment, with or without transition to the dark (‘dark’ and ‘light’ respectively), as measured by positive Sytox staining. Data is shown as mean ± SEM, n = 3 biological repeats. P values: *=0.0064, **=0.0026, ***=2·10−5, t-test.
-
Figure 7—source data 1
Flow cytometry measurements of chl-roGFP oxidation in response to dark and H2O2 treatments.
- https://doi.org/10.7554/eLife.47732.034
-
Figure 7—source data 2
Cell death in response to dark and H2O2 treatments.
- https://doi.org/10.7554/eLife.47732.035
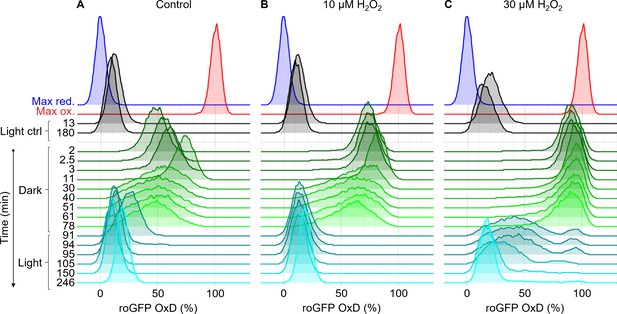
Redox response of chl-roGFP to transition to the dark.
Flow cytometry measurements of chl-roGFP oxidation in the population over time. Cells were treated with 0 µM (basal, A, same as in Figure 7A), 10 µM (B), and 30 µM H2O2 (C), and were then transitioned to the dark at time 0 (within 10 min post treatment). Cells were kept in the dark for 90 min (green) and were then transferred back to the light (cyan). For reference, chl-roGFP OxD following the same H2O2 treatment but without transition to the dark (black) and following maximum oxidation (200 µM H2O2, red) and maximum reduction (2 mM DTT, blue) are shown. The experiment was done in triplicates that were highly similar, for visualization purposes the first replica is shown. Each histogram consists of >8000 cells.
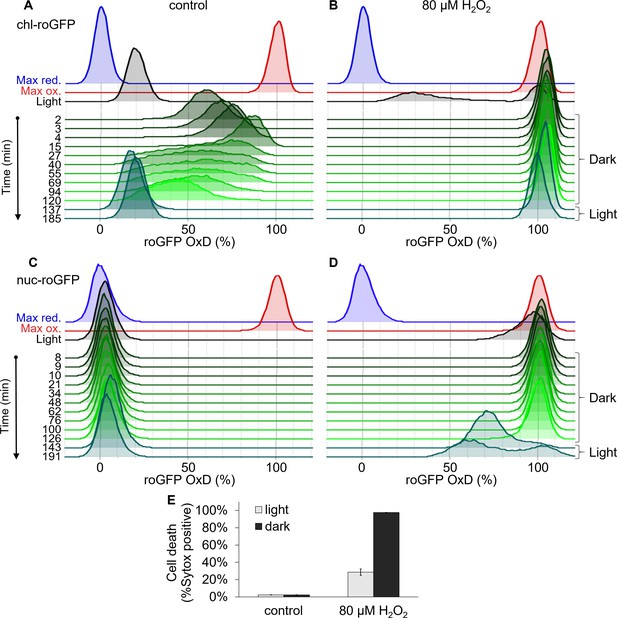
Responses of chloroplast and nucleus targeted roGFP to transition to the dark.
Measurements of roGFP oxidation response in the chloroplast and nucleus to a temporal transition to the dark. (A–D) Flow cytometry measurements of roGFP oxidation dynamics in the population over time in the chloroplast (A–B) and nucleus (C–D). Cells were treated with 80 µM H2O2 (B, D) or untreated control (A, C), and were then transitioned to the dark at time 0 (within 4 min post treatment). Cells were kept in the dark for 120 min (green) and were then transferred back to the light (dark cyan). For reference, roGFP OxD following the same H2O2 treatment but without transition to the dark (black, ~130 min post treatment) and following maximum oxidation (200 µM H2O2, red) and maximum reduction (2 mM DTT, blue) are shown. (E) The fraction of dead cells 24 hr post H2O2 treatment with or without transition to the dark (‘dark’ and ‘light’ respectively) as measured by positive Sytox staining in chl-roGFP strain. Data is shown as mean ± SEM, n = 3 biological repeats.
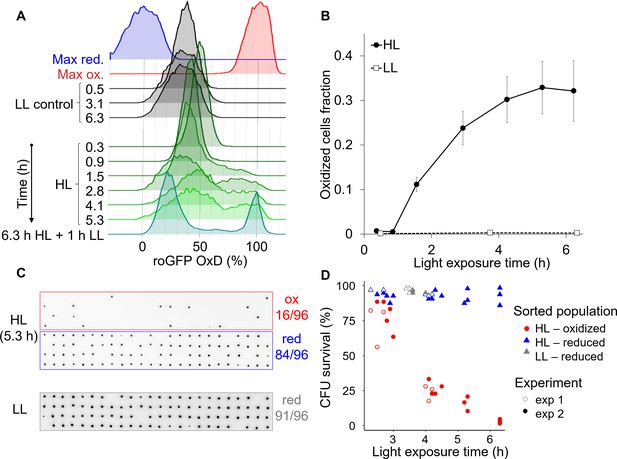
High light induces the emergence of an ‘oxidized’ subpopulation with decreased survival.
Cells expressing chl-roGFP were exposed to either high light (HL, 2000 µmol photons m−2 s−1) or low light (LL, 14 µmol photons m−2 s−1), and were FACS sorted based on their chl-roGFP oxidation at different times of light exposure to measure single-cell survival using the colony forming units (CFU) assay. (A) Flow cytometry measurements of chl-roGFP OxD distribution in the population over time of HL exposure. Cells were kept in HL for 6.3 hr (green) and were then transferred back to LL for 1 hr (cyan). LL control (black) and maximum oxidation (200 µM H2O2, red) and reduction (2 mM DTT, blue) are shown for reference. The experiment was done in triplicates, for visualization the first replica is shown. Each histogram consists of >9000 cells. (B) The fraction of oxidized chl-roGFP cells over time of exposure to HL. Mean ± SEM, N = 3. (C) Images of chlorophyll auto-fluorescence of colonies generated from single cells that were sorted based on chl-roGFP oxidation following exposure to HL or LL for the CFU assay. Panels from top to bottom: oxidized (red box) and reduced (blue box) subpopulations sorted after 5.3 hr of HL, reduced subpopulation sorted under LL control conditions (gray box). CFU survival (%) was measured by the number of colonies divided by number of sorted cells, as shown at the right of each panel. Gates used for sorting are shown in Figure 8—figure supplement 1. (D) CFU survival (%) of single cells that were sorted based on chl-roGFP oxidation at different times of light exposure onto agar plates. Data of 2 independent experiments each with three biological repeats, 64–96 sorted cells per repeat, individual repeats are shown. (A–D) Data points of 6.3 hr HL exposure were exposed to HL for 6.3 hr and then moved back to LL for 1 hr.
-
Figure 8—source data 1
The fraction of oxidized cells over time in response to high light.
- https://doi.org/10.7554/eLife.47732.039
-
Figure 8—source data 2
Colony forming units survival of single cells sorted following high light exposure based on chl-roGFP oxidation.
- https://doi.org/10.7554/eLife.47732.040
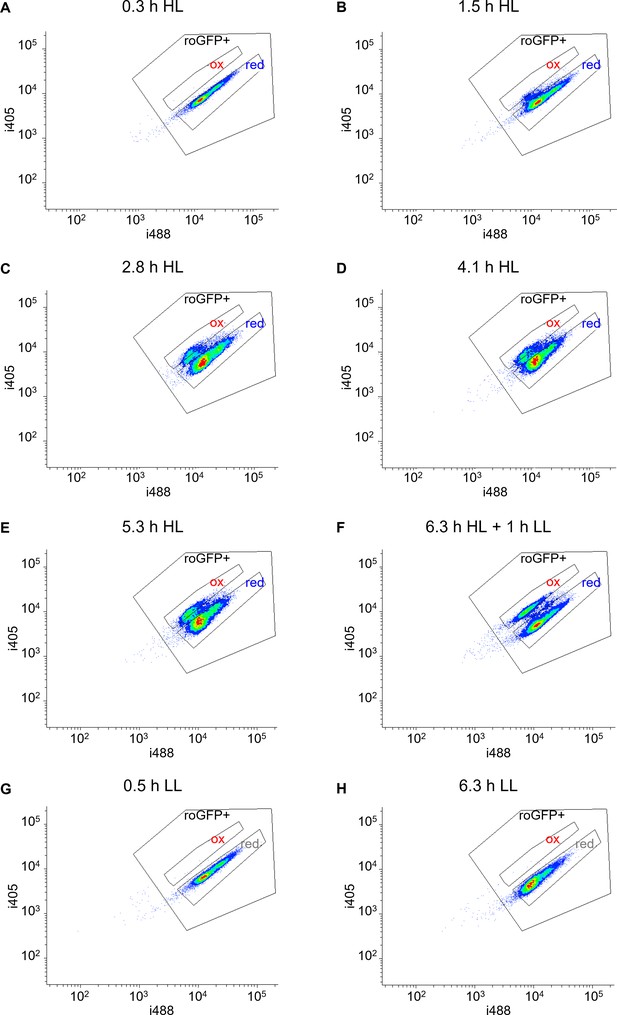
High light induces the emergence of an oxidized subpopulation.
(A–H) Density plots of chl-roGFP fluorescence measured by flow cytometry over time following exposure to high light (HL, 2000 µmol photons m−2 s−1, (A–F) or under control low light conditions (LL, 14 µmol photons m−2 s−1, (G–H). The ratio between the two excitation wavelengths (i405/i488) increases upon roGFP oxidation and is used to measure roGFP oxidation degree (see Materials and methods). Gates used for sorting the oxidized (‘ox’) and reduced (‘red’) subpopulations for the CFU assay are shown. roGFP+: positive roGFP expression gate, discriminating cells with low or absent roGFP expression. F: Cells were exposed to HL for 6.3 hr and then moved back to LL for 1 hr.
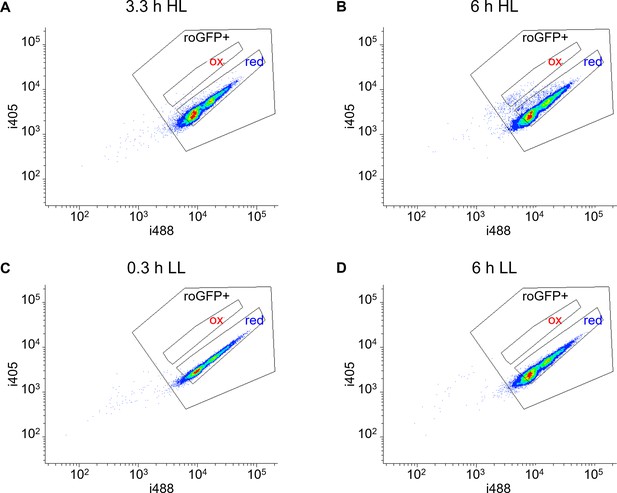
Measurements of nuclear roGFP oxidation in response to high light.
(A–D) Density plots of nuc-roGFP fluorescence measured by flow cytometry over time following exposure to high light (HL, 2000 µmol photons m−2 s−1, (A–B) or under control low light conditions (LL, 14 µmol photons m−2 s−1, (C–D). The ratio between the two excitation wavelengths (i405/i488) increases upon roGFP oxidation and is used to measure roGFP oxidation degree (see Materials and methods). Gates used for sorting the oxidized (‘ox’) and reduced (‘red’) subpopulations for the CFU assay in the chl-roGFP strain are shown for reference. roGFP+: positive roGFP expression gate, discriminating cells with low or absent roGFP expression.
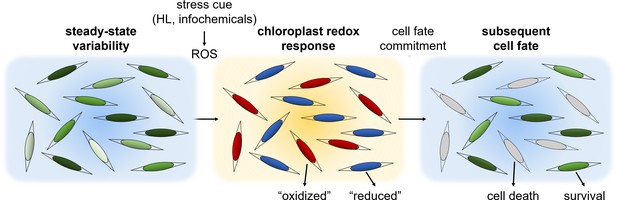
A conceptual model: phenotypic variability within diatom populations affects cell fate determination in response to stress conditions.
At steady state conditions, cells within the population have diverse metabolic states due to various factors such as local ROS levels, antioxidant capacity, metabolic activity, growth phase and cell cycle position. We propose that this variability could lead to a differential response to environmental stressors. Exposure to specific stress conditions, such as high light (HL) or toxic infochemicals, can lead to ROS accumulation at different subcellular compartments, including the chloroplast, which is used to sense the stress cue and regulate cell fate. Cells at a more susceptible metabolic state may accumulate high ROS levels and will subsequently die, as observed in the ‘oxidized’ subpopulation. More resilient cells may exhibit milder oxidation and may be able to acclimate, as observed in the ‘reduced’ subpopulation. Chloroplast EGSH oxidation is an early stage in this stress response and precedes the commitment to cell fate.
Videos
Microfluidics in vivo imaging of chl-roGFP oxidation over time following H2O2 treatment.
Oxidation of chl-roGFP cells was imaged over time using a customized microfluidic setup and epifluorescence microscopy with controlled flow, light and temperature conditions (see Materials and methods). Movie of chl-roGFP OxD in pseudo-color of cells treated with 80 µM H2O2 at time 0, the first frame is before treatment. Time stamp represents time post treatment (hh:mm). Color bar as in Figure 4.
Microfluidics in vivo imaging of basal chl-roGFP oxidation over time.
Oxidation of chl-roGFP cells was imaged over time using a customized microfluidic setup and epifluorescence microscopy with controlled flow, light and temperature conditions (see Materials and methods). Movie of chl-roGFP OxD in pseudo-color of cells treated with fresh media (control) at time 0, the first frame is before treatment. Time stamp represents time post treatment (hh:mm). Color bar as in Figure 4.
Tables
Reagent type (species) or resource | Designation | Source or reference | Identifiers | Additional information |
---|---|---|---|---|
Strain, strain background (Phaeodactylum tricornutum) | Pt1 8.6 (WT) | National Center of Marine Algae and Microbiota (NCMA) | CCMP2561 | |
Strain, strain background (Phaeodactylum tricornutum) | chl-roGFP | (Rosenwasser et al., 2014) | ||
Strain, strain background (Phaeodactylum tricornutum) | nuc-roGFP | (Rosenwasser et al., 2014) | ||
Strain, strain background (Phaeodactylum tricornutum) | mit-roGFP | (Rosenwasser et al., 2014) | ||
Commercial assay or kit | Sytox Green | Invitrogen | ||
Software, algorithm | Image analysis MATLAB script | This paper and on GitHub (Mizrachi, 2018): https://github.com/aviamiz/ITRIA |
Dynamic range of roGFP targeted to different organelles.
The dynamic range (Rox/Rred) was calculated by dividing the mean roGFP ratio under maximum oxidation conditions (200 µM H2O2) by that under maximum reduction conditions (2 mM DTT) using data from flow cytometry and microfluidics imaging experiments.
P. tricornutum strain | Flow cytometry dynamic range | Microfluidics imaging dynamic range |
---|---|---|
chl-roGFP | 5.57 | 5.42 |
nuc-roGFP | 5.94 | - |
mit-roGFP | 3.50 | - |
Additional files
-
Transparent reporting form
- https://doi.org/10.7554/eLife.47732.043