Kairomones: Finding the fish factor
In a very simplified version of the food chain found in lakes, microalgae are eaten by water fleas called Daphnia, which are in turn eaten by fish. But things get complicated very quickly if observed in more detail. Algae release toxins to defend themselves, and form long chains to evade predators (Van Donk et al., 2011), while Daphnia can change shape or move to avoid being eaten by fish.
One way that Daphnia and other members of the zooplankton avoid predators is by moving to different depths of the lake depending on the time of day, a strategy known as diel vertical migration. If the surrounding water contains fish, Daphnia move to darker, deeper regions during the day, so that the fish cannot see them (Figure 1), and move to the upper layers of the water column – where the microalgae live – at night. If there are not many fish in the vicinity, Daphnia stay near the surface during the day as well (Lampert, 1989).
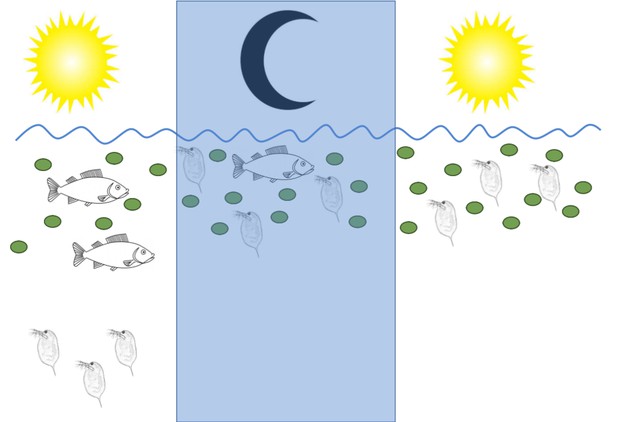
Daphnia water fleas change their behavior if fish are present.
Left: During the day Daphnia migrate to deeper, darker regions of the lake if they detect chemical signals called kairomones (not shown) that are released by fish. Middle: At night, when fish cannot see them, Daphnia move up to the water near the surface to eat the microalgae (green circles) that are plentiful there. Right: When no fish are present, there are no kairomones to detect, and Daphnia stay near the surface day and night.
Prey species must balance their resources carefully. Unnecessarily avoiding predators costs energy and can restrict access to food – the microalgae eaten by Daphnia do not live in the dark depths of the lake – but accidentally encountering a predator can be fatal. As a result, some species have adapted to detect chemicals released by predators. The identification of several of these chemicals, called kairomones, has opened up new areas of research in aquatic ecology, conservation and aquaculture (Yasumoto et al., 2005; Selander et al., 2015; Weiss et al., 2018).
The search for the kairomone that induces diel vertical migration, also known as the ‘fish factor’, has been ongoing for decades, with spectacular failures and misinterpretations on the way (see Pohnert and von Elert, 2000 for a discussion). Numerous obstacles have complicated the search: the fish factor occurs in low concentrations in lake water, and bioassay experiments that could identify it are problematic because it is difficult to monitor the vertical movement of Daphnia in a laboratory setting. Now, in eLife, Meike Hahn, Christoph Effertz, Laurent Bigler and Eric von Elert report the identity of this kairomone (Hahn et al., 2019).
Hahn et al. – who are based at the University of Cologne and the University of Zurich – used a bioassay-guided fractionation method to identify the fish factor. A technique called High Performance Liquid Chromatography allowed water in which fish had previously been incubated to be separated into ‘fractions’ that each contained a subset of chemicals. Examining the effect of each fraction on the migration behavior of Daphnia revealed one that induced diel vertical migration even though fish were not present. Hahn et al. identified the active chemical as 5α-cyprinol sulfate. Only picomolar concentrations of this compound are found in water inhabited by fish, but even these low concentrations are sufficient to change the migration behavior of Daphnia.
Since the release of kairomones places predator species at a disadvantage, a prey species can only rely on them if the predator cannot shut down the production of the molecule. This is the case for 5α-cyprinol sulfate, which is a bile acid that plays an essential role in digesting dietary fats (Hofmann et al., 2010). The fish release 5α-cyprinol sulfate from their intestine, gills, and the urinary tract. As this molecule is also stable in water, it reliably indicates the presence of fish to Daphnia.
Besides the many implications for basic research, the finding that only picomolar amounts of a compound can trigger widespread behavioral responses in a lake also raises ecotoxicological concerns. While we survey our waters for metabolites that cause immediate toxicity, we completely ignore the fact that non-toxic doses of such highly potent signaling chemicals can also have a substantial effect on an ecosystem. This calls for a new evaluation of the routine procedures used in environmental monitoring.
Kairomones are not the only chemical signals used by the species that inhabit lakes. Pheromones (Frenkel et al., 2014), defense metabolites and molecules that help species to outcompete each other also contribute to the intricate signaling mechanisms in aquatic ecosystems (Berry et al., 2008). We can conclude that these environments are really shaped by a diverse chemical landscape, a language of life that we are only just beginning to understand.
References
-
Pheromone signaling during sexual reproduction in algaeThe Plant Journal 79:632–644.https://doi.org/10.1111/tpj.12496
-
Bile salts of vertebrates: structural variation and possible evolutionary significanceJournal of Lipid Research 51:226–246.https://doi.org/10.1194/jlr.R000042
-
The adaptive significance of diel vertical migration of zooplanktonFunctional Ecology 3:21–27.https://doi.org/10.2307/2389671
-
No ecological relevance of trimethylamine in fish–Daphnia interactionsLimnology and Oceanography 45:1153–1156.https://doi.org/10.4319/lo.2000.45.5.1153
-
Identification of Chaoborus kairomone chemicals that induce defences in DaphniaNature Chemical Biology 14:1133–1139.https://doi.org/10.1038/s41589-018-0164-7
Article and author information
Author details
Publication history
Copyright
© 2019, Pohnert
This article is distributed under the terms of the Creative Commons Attribution License, which permits unrestricted use and redistribution provided that the original author and source are credited.
Metrics
-
- 1,876
- views
-
- 127
- downloads
-
- 89
- citations
Views, downloads and citations are aggregated across all versions of this paper published by eLife.
Download links
Downloads (link to download the article as PDF)
Open citations (links to open the citations from this article in various online reference manager services)
Cite this article (links to download the citations from this article in formats compatible with various reference manager tools)
Further reading
-
- Ecology
- Microbiology and Infectious Disease
Interspecies interactions involving direct competition via bacteriocin production play a vital role in shaping ecological dynamics within microbial ecosystems. For instance, the ribosomally produced siderophore bacteriocins, known as class IIb microcins, affect the colonization of host-associated pathogenic Enterobacteriaceae species. Notably, to date, only five of these antimicrobials have been identified, all derived from specific Escherichia coli and Klebsiella pneumoniae strains. We hypothesized that class IIb microcin production extends beyond these specific compounds and organisms. With a customized informatics-driven approach, screening bacterial genomes in public databases with BLAST and manual curation, we have discovered 12 previously unknown class IIb microcins in seven additional Enterobacteriaceae species, encompassing phytopathogens and environmental isolates. We introduce three novel clades of microcins (MccW, MccX, and MccZ), while also identifying eight new variants of the five known class IIb microcins. To validate their antimicrobial potential, we heterologously expressed these microcins in E. coli and demonstrated efficacy against a variety of bacterial isolates, including plant pathogens from the genera Brenneria, Gibbsiella, and Rahnella. Two newly discovered microcins exhibit activity against Gram-negative ESKAPE pathogens, i.e., Acinetobacter baumannii or Pseudomonas aeruginosa, providing the first evidence that class IIb microcins can target bacteria outside of the Enterobacteriaceae family. This study underscores that class IIb microcin genes are more prevalent in the microbial world than previously recognized and that synthetic hybrid microcins can be a viable tool to target clinically relevant drug-resistant pathogens. Our findings hold significant promise for the development of innovative engineered live biotherapeutic products tailored to combat these resilient bacteria.
-
- Ecology
For the first time in any animal, we show that nocturnal bull ants use the exceedingly dim polarisation pattern produced by the moon for overnight navigation. The sun or moon can provide directional information via their position; however, they can often be obstructed by clouds, canopy, or the horizon. Despite being hidden, these bodies can still provide compass information through the polarised light pattern they produce/reflect. Sunlight produces polarised light patterns across the overhead sky as it enters the atmosphere, and solar polarised light is a well-known compass cue for navigating animals. Moonlight produces an analogous pattern, albeit a million times dimmer than sunlight. Here, we show evidence that polarised moonlight forms part of the celestial compass of navigating nocturnal ants. Nocturnal bull ants leave their nest at twilight and rely heavily on the overhead solar polarisation pattern to navigate. Yet many foragers return home overnight when the sun cannot guide them. We demonstrate that these bull ants use polarised moonlight to navigate home during the night, by rotating the overhead polarisation pattern above homing ants, who alter their headings in response. Furthermore, these ants can detect this cue throughout the lunar month, even under crescent moons, when polarised light levels are at their lowest. Finally, we show the long-term incorporation of this moonlight pattern into the ants’ path integration system throughout the night for homing, as polarised sunlight is incorporated throughout the day.