mPFC spindle cycles organize sparse thalamic activation and recently active CA1 cells during non-REM sleep
Figures
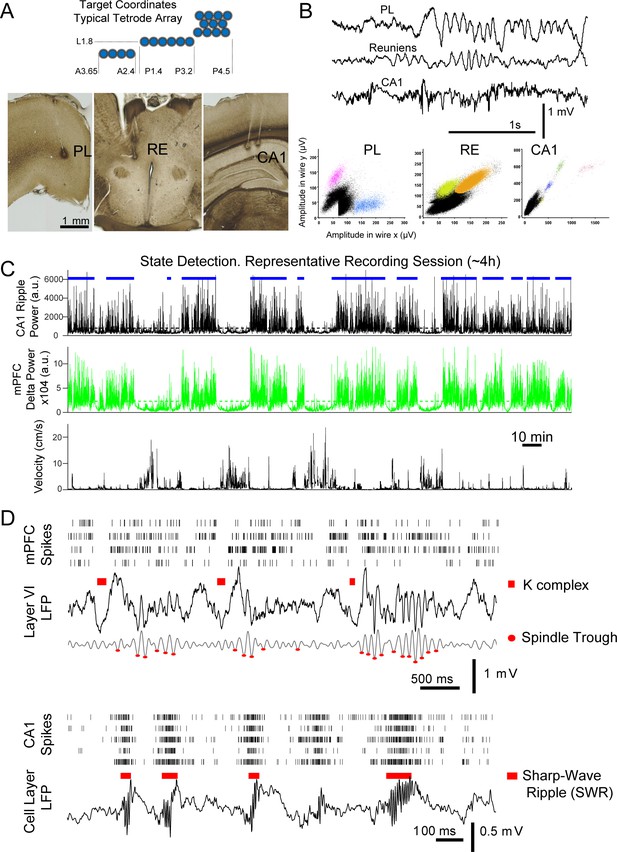
Identification of non-REM sleep oscillatory events in mPFC and CA1.
(A) Diagram of the cross-section of a typical multi-tetrode array and target coordinates. (B) Sample LFP traces and units simultaneously recorded in mPFC (prelimbic region, PL), reuniens (RE), and CA1. (C) Resting states (blue horizontal bars) were detected from the moving average of the squared filtered LFP, SWR-filtered from CA1 -top panel-, and delta-filtered from mPFC -middle panel-; selected periods used for analyses were confirmed by visual inspection and observation of the corresponding animal velocity -bottom panel-. (D) Multi-unit spike rasters (each row shows all spikes from one tetrode) and LFPs, illustrate the detection of sleep events used for analyses; top: detected K-complexes (marked above raw LFP trace) and spindle troughs (marked on the 6–14 Hz filtered LFP) in mPFC; bottom: examples of CA1 sharp-wave ripple detection.
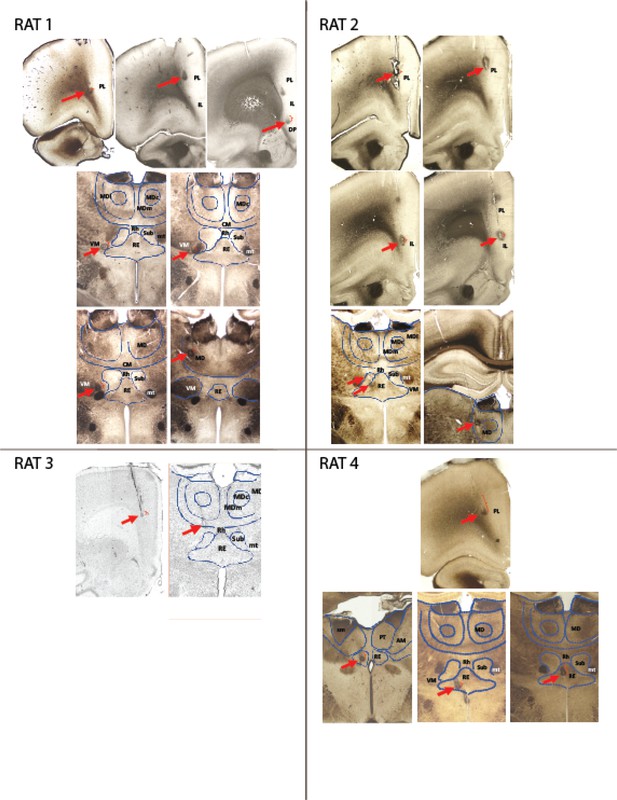
Lesions and electrode trajectory for tetrodes included in analyses.
Each picture displays a coronal section through the rat brain at the anterioposterior level where one or more lesions were identified; red arrows point to lesions; red brackets mark the tetrode trajectory (based on annotated movement) traversed by the tetrode during the recording sessions used for analyses. Blue lines outline some of the midline nuclei for reference. Abbreviations: PL = Prelimbic; IL = infralimbic; DP = dorsopeduncular cortex; MD = Mediodorsal lateral, central and medial subdivisions-; Rh = rhomboid; RE = Reuniens; CM = centromedial; VM = ventromedial; Sub = Submedius; mt = mamillothalamic tract; PT = paratenial; AM = anteromedial; sm = stria medullaris. mt = mamillothalamic tract.
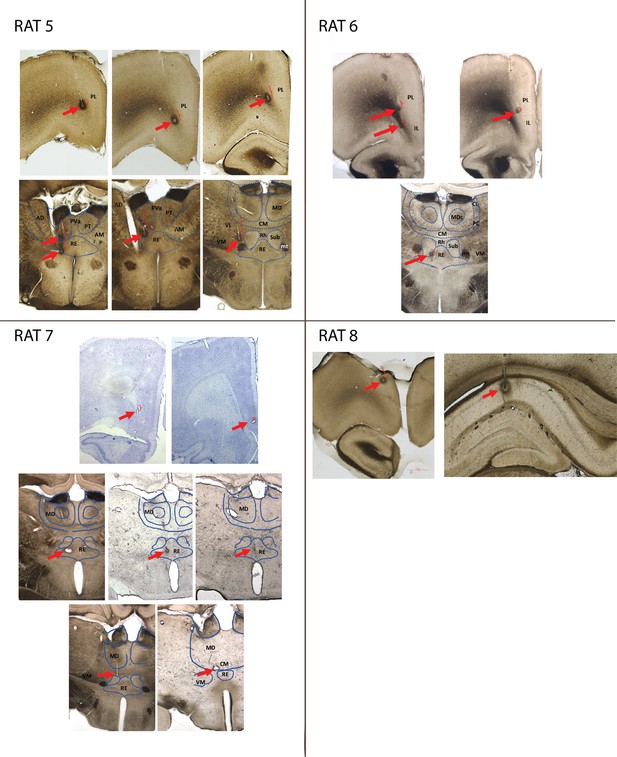
Lesions and electrode trajectory for tetrodes included in analyses (continued).
Abbreviations: AD = anteriodorsal; PVa = paraventricular, anterior subdivision; other abbreviations as in Part 1.
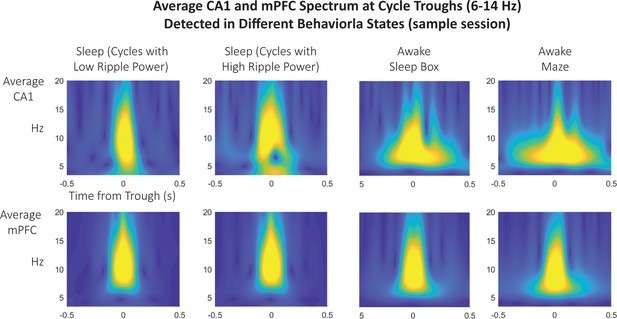
Spindle trough-triggered wavelet scalogram of CA1 and mPFC LFP in sleep compared to quiet and active wakefulness.
Spindle cycles detected during sleep are associated with spectral power concentrated in the 10–15 Hz band, suggestive of spindle (and not theta) detection. Only spindle troughs detected during sleep were used for analyses. Instead, running the algorithm for spindle trough detection during either quiet wakefulness (when the rat is in the sleep box) or active wakefulness (during exploration of the radial maze) have different profiles indicative of theta, notably, the CA1 scalogram displays lower frequency and is more sustained in time.
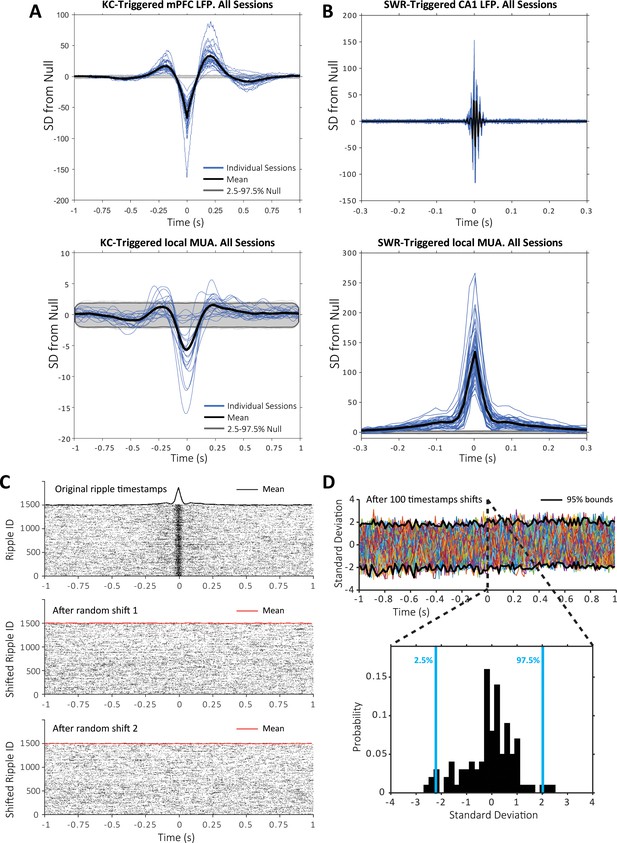
Event-triggered LFP and spike averages across sessions.
(A) K-complex (KC) triggered average of the LFP and multi-unit spikes recorded by the same tetrode used for KC detection; top plot: average KC triggered LFP for individual sessions (blue), across sessions (black) and null distribution (generated by shuffling KC timestamps); bottom plot: average KC-triggered multi-unit spikes for individual sessions (blue), across sessions (black) and null distribution (generated by shuffling the KC timestamps). (B) Same for SWRs. (C) Example of null shuffle distribution calculation for one of the sessions; top raster plot: spikes at the timestamps of SWRs detected for this session during sleep (mean peri-SWR histogram in black at the top of the raster); middle plot: example of the raster of spikes obtained after shifting all SWRs by a random delay between 1.5–2.5 s (mean peri-SWR histogram in red at the top of the raster); bottom plot: peri-SWRs raster of spikes for a second random shift. (D) Example of the distribution of mean peri-SWRs histograms after 100 SWR shifts for the session in (C), that is, null distribution for probabilistic inference per time bin; inset shows the probability density function and bounds containing the central 95% of the distribution at representative time bin 0 s.
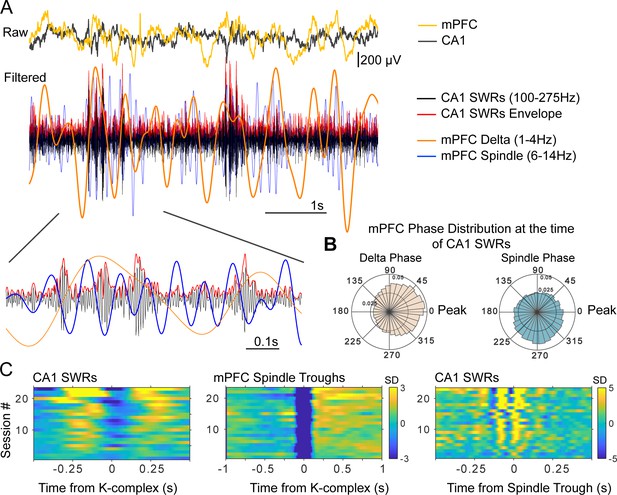
Time and phase coordination between non-REM sleep events.
(A) Overlaid raw and filtered mPFC and CA1 LFPs illustrate the time relations of the relevant oscillatory events (filtered traces have been scaled in amplitude for display purposes). (B) Average (across sessions and rats) distribution of delta and spindle phase at the time of SWRs, showing a preferential occurrence of SWRs near the peak of delta and the ascending phase of spindles (inner and outer circle values indicate probabilities). (C) Peri-event histograms of detected non-REM sleep events show that SWR probability increases in the ~250 ms windows near KCs (left panel), and are nested in the negative half-cycle of spindles (right), spindles are largest in amplitude following KCs (middle) (color scale bars indicate standard deviations (SD) with respect to a null, shuffled, distribution; scale bar in the middle panel applies also to the left panel).
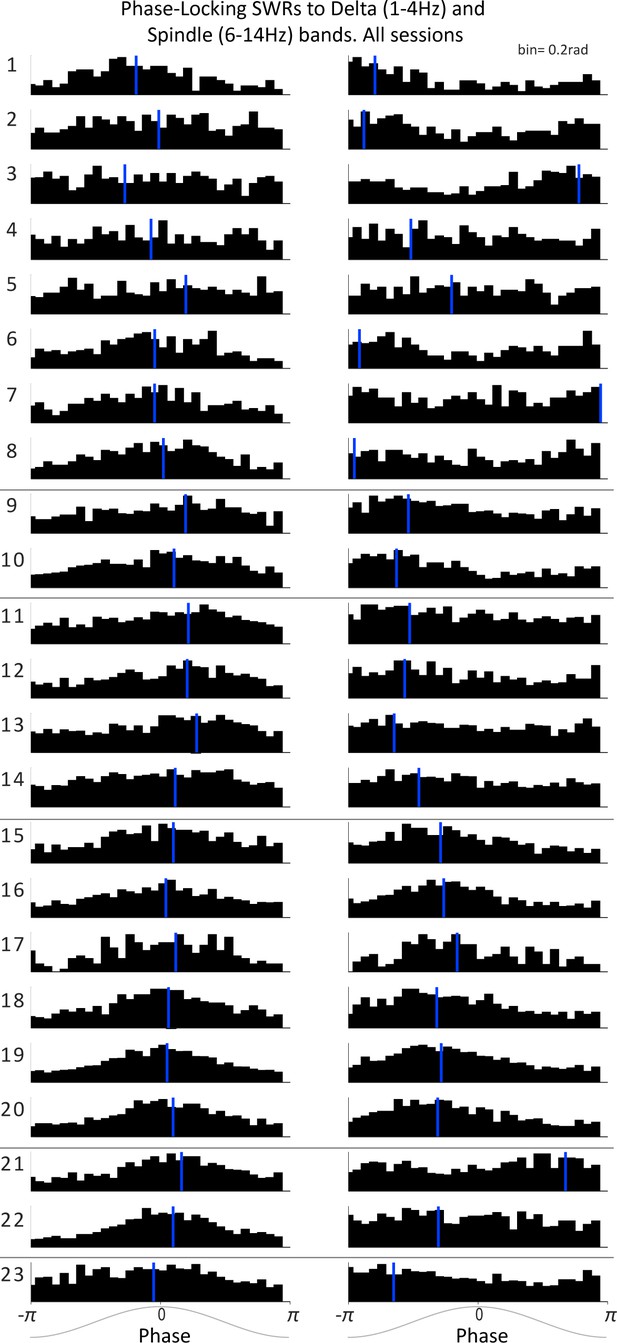
Phase-Locking of SWRs to mPFC delta and spindle frequency bands.
Distributions of mPFC LFP phase at CA1 SWRs times for the delta-filtered (left) and spindle-filtered (right) mPFC LFP for all sessions (in rows; horizontal black lines separate sessions from different rats); vertical blue lines mark the circular mean of each distribution.
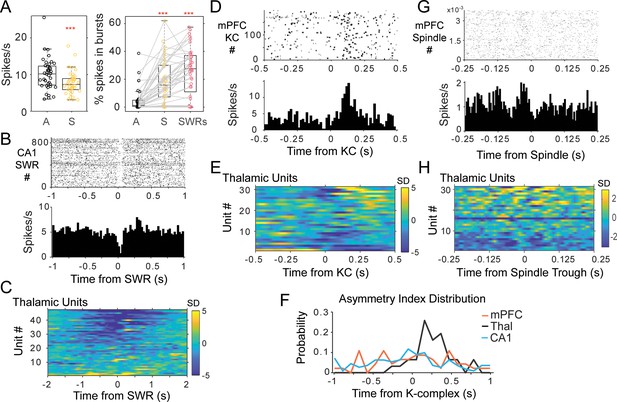
Thalamic single unit firing with non-REM sleep oscillations.
(A) Left, distributions of average firing rate for all units in the awake, A-black, compared to sleep periods, S-yellow. Right, distributions of the percent of spikes in bursts for the same units during wakefulness, sleep, and in a window of 2 s around the occurrence of CA1 SWRs. (B) Example of peri-SWR-triggered histogram for a unit recorded in the nucleus reuniens, showing a decrease in firing tightly locked to SWR occurrence (top plot is the raster of unit spikes for all detected SWRs). (C) Population results of peri-SWR triggered histogram of spikes for all thalamic units; scale bars units are in standard deviations (SD) from the null, shuffled, distribution. (D) peri-KC histogram shows a rebound of firing in a unit recorded in MD thalamus; (E) peri-KC histograms for all thalamic units. (F) Distribution of the asymmetry index in the three cell populations highlights the asymmetric response in the thalamic population compared to the other two groups. (G) Spindle-trough triggered histograms for a unit from MD, (H) same for all the thalamic units.
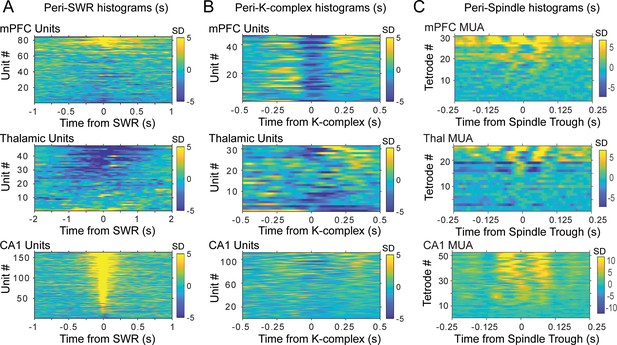
Peri-event histograms of spikes in the three brain regions for all rats and sessions.
X axis indicates time from reference event, that is , SWR in (A), K-complex in (B) and spindle trough in (C). Each row (Y axis) is the peri-event histogram for one unit or all the spikes recorded by one tetrode (in C); color bars (Z axis) indicate standard deviations from the shuffled distribution.
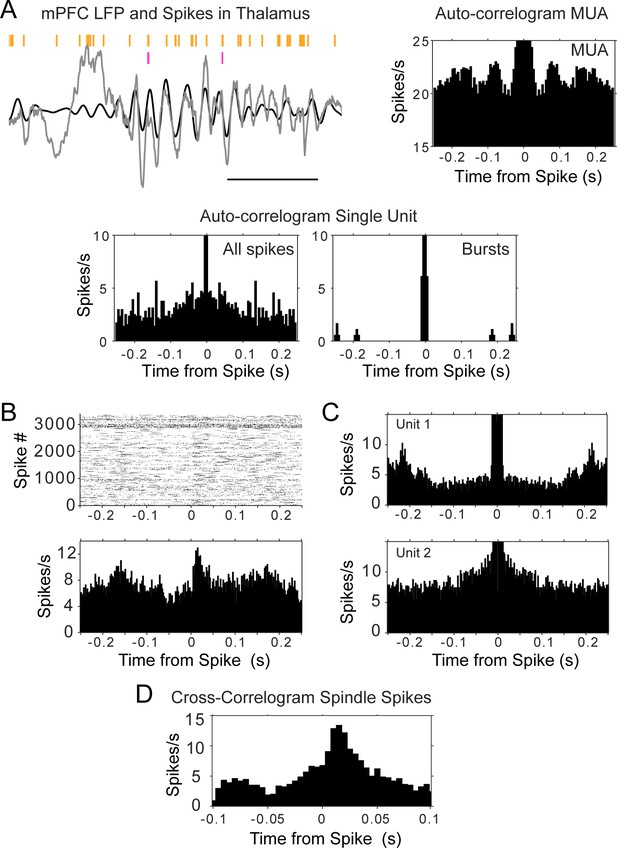
Auto- and cross-correlograms of thalamic spikes show lack of single unit rhythmicity but consistent activation of MUA and unit pairs.
(A) Top left, raw trace of mPFC LFP in gray, with overlaid filtered trace (6–14 Hz, black); spike raster in orange corresponds to all spikes (multi-unit activity, MUA) recorded by one thalamic tetrode, raster in magenta is from a single unit recorded by the same tetrode; scale bar 500 ms. Bottom, auto-correlograms of thalamic spikes during sleep for the single unit (all spikes were used for the plot on the left, burst spikes only for the plot on the right); top right, only the auto-correlogram for the MUA recorded by the same electrode displays rhythmic firing at spindle frequency. (B) Cross-correlogram between the sleep spikes of two thalamic units, showing increased modulation within about 50 ms, even when the auto-correlograms of the spikes from each individual unit (C) does not show rhythmic firing. (D) Cross-correlogram of the same thalamic pair, calculated only with spikes within spindle cycles, suggests spindle-related co-activation.
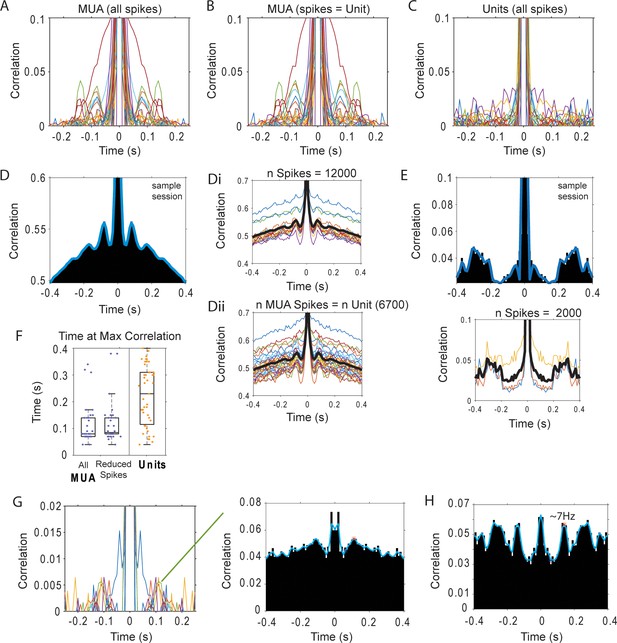
Autocorrelation of thalamic MUA and unit spikes during sleep.
(A) Autocorrelation of MUA spikes (n = 30 sessions; each color one session). (B) Average of autocorrelations calculated in blocks of spikes with the same number as the sparsest unit in the session. (C) Autocorrelation of unit spikes recorded in the same sessions (each color one unit; n = 47). (D) Left, autocorrelation of the MUA spikes for one sample session; Di, autocorrelations for each block of 12,000 spikes through the recording; Dii, autocorrelations in blocks of 6700 spikes –the number of spikes produced during sleep by a thalamic unit recorded by the same electrode-. (E) Autocorrelation of the thalamic unit (recorded in the session shown in D) for all the spikes, and calculated in blocks of 2000 spikes (bottom plot). (F) Distribution of times at autocorrelation maximum. (G) Autocorrelation of units in which the maximum value falls within spindle band lags (6–14 Hz). (H) Single unit with the strongest indication of rhythmic firing.
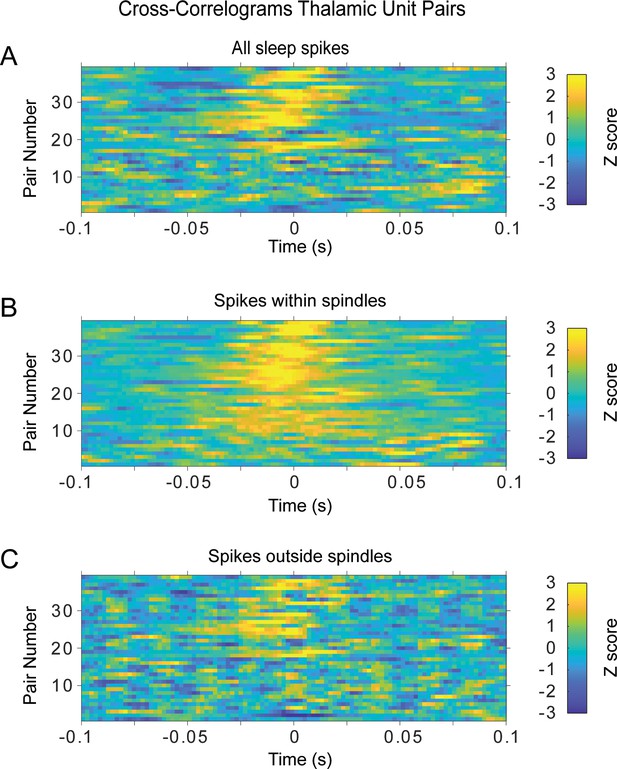
Cross-correlograms of sleep spikes for simultaneously recorded thalamic units.
Cross-correlations of spikes from simultaneously recorded thalamic pairs (n = 39; each row one pair) show a peak correlation within tenths of milliseconds, consistent with correlations within the duration of spindle cycles. (A) All sleep spikes. (B) Spikes within spindle cycles for the same pair.(C) Spikes within sleep periods between detected spindles (no spindles for at least 5 s). Rows are in the same order in all panels, based on the peak z-score in (B), which has the pair with highest z-score on the top row.
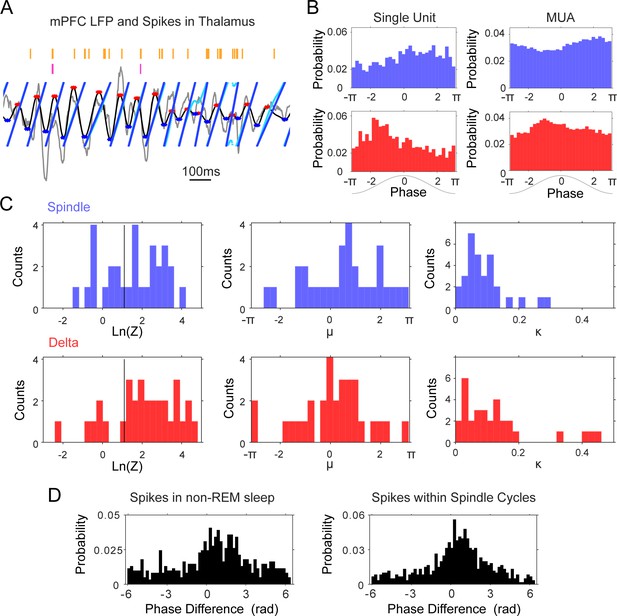
Phase-locking of thalamic units to mPFC delta (1–4 Hz) and spindle (6–14 Hz) frequencies.
(A) Instantaneous phase estimate for a filtered mPFC LFP trace using the Hilbert transform (cyan) or peak-trough interpolation (blue). (B) Spindle (blue) and delta (red) phase-distribution at the time of single unit and multi-unit spikes recorded during sleep in one tetrode in thalamus (sample rasters in Figure 7a); the non-uniform distributions suggest phase-locking of these spikes to the descending phase of the spindle oscillation and the ascending phase of delta. (C) Left, distribution of log-transformed Rayleigh’s Z for all thalamic units, used to evaluate the significance of phase-locking to the two frequency bands; values to the right of the black vertical line are significant at the p=0.05 level. Panels on the right side show the distribution of the μ (mean direction, middle panel) and κ (concentration, right panel) of the phase-distribution for all units, which suggest preferential firing at the peak of delta and descending phase of spindle oscillations. (D) Phase lag distributions for the thalamic pair in Figure 4B–D; spindle phase-difference between the closest spikes from the two units was calculated for all spikes in non-REM sleep (Left) and for all spikes within spindle cycles (Right), and suggests that the two units are most likely to fire in sequence within about a quarter of a spindle cycle from each other.
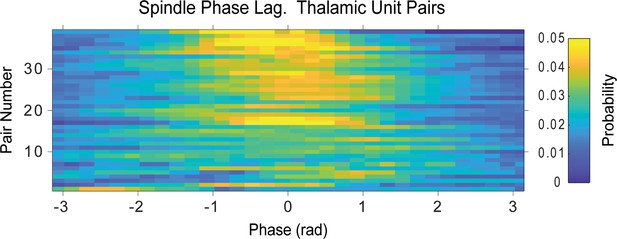
Distribution of phase lags between the spikes of simultaneously recorded thalamic unit pairs suggest their consistent activation within spindle cycles.
Phase difference calculated from the closest spikes during spindle cycles. Rows correspond to the same unit pairs as in Figure 4—figure supplement 2.
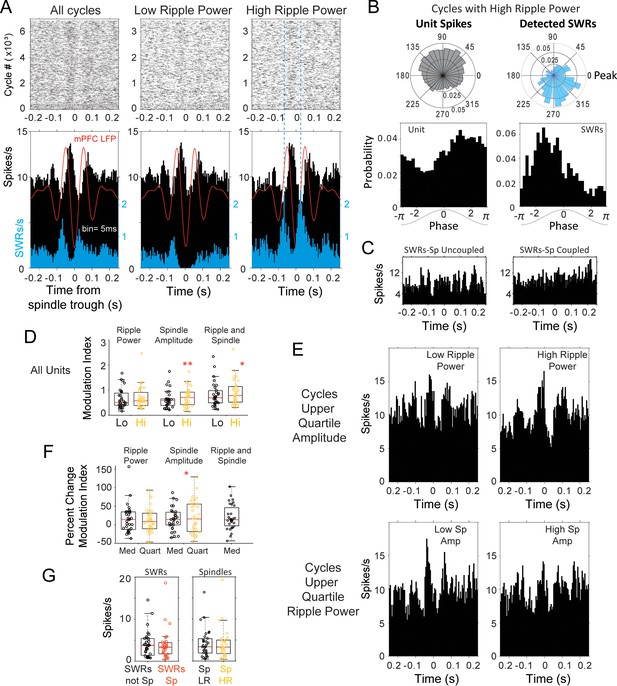
Modulation of spindle-related thalamic activity with SWRs.
(A) Spike rasters and peri-spindle-trough histograms of thalamic spikes from one unit and for SWRs recorded in the same session during sleep. Spindle cycles were classified into low and high ripple power, according to the median power in the CA1 ripple band during the cycle. In this unit there was an increased modulation of firing in spindles with high ripple power (right plot), compared to those with low power (middle); the spindle-triggered histogram of SWRs (blue) confirms the increased probability of SWR events in high-ripple power spindles. The average spindle-triggered mPFC LFP is overlaid in red. (B) Example of phase-locking for the unit and SWRs; spikes from the thalamic unit in (A) occurred preferentially in the descending phase of spindles with high ripple power, in contrast to the SWR preference for ascending phases. (C) Peri-SWR histograms of the same unit in A-B for SWRs coupled and uncoupled to spindles showed no clear correlation with SWRs. (D) Distributions of spike modulation index and the percent of change in modulation index. (E) Increased peri-spindle modulation with high ripple power still observed in spindles that are not significantly different in amplitude (upper amplitude quartile; top row); peri-spindle modulation decreases with high spindle amplitude in this unit (in cycles that are not significantly different in ripple power; bottom row). (F) For the thalamic population; changes in spike modulation were significant at the population level with spindle amplitude (each circle one unit; Hi = cycles with high ripple power, Lo = low ripple power; Med = spindles classified based on median ripple power or amplitude, Quart = spindles classified based on upper quartile of ripple power or amplitude). (G) Left, average thalamic firing in peri-SWRs histograms with SWRs nested in spindles (SWRs Sp, red) compared to those outside spindles (SWRs not Sp). Right, average firing in spindles with low (Sp LR, yellow) and high ripple power (Sp HR).
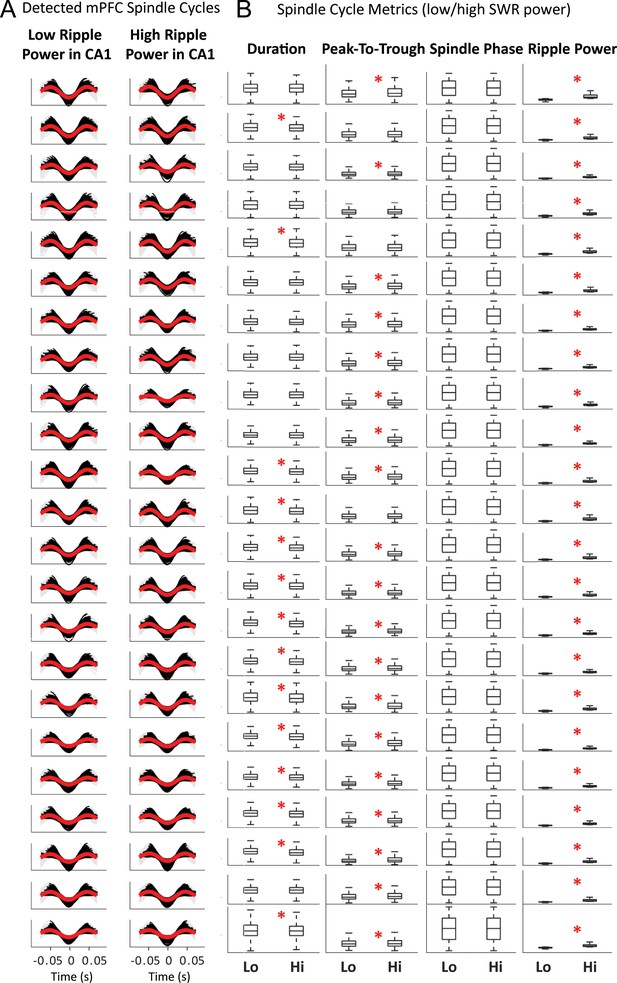
Detected spindle cycles for all sessions, classified by mean CA1 ripple power within the duration of the cycle.
After detection of spindle troughs in the filtered mPFC LFP, individual spindle cycles were identified by detecting the peak before and after the trough, then cycles were classified based on the CA1 SWR power being above (high) or below (low) the median for all cycles. (A) Each plot shows the LFP spindle-filtered trace of all cycles identified in one session in black, and their average in red, gray traces complete the wave to facilitate plotting for display purposes only; cycles with low SWR power on the left, high-ripple power cycles on the right. (B) Whisker-plot distributions of feature metrics for low- and high-SWR spindle cycles (Lo, Hi) in the corresponding sessions: cycle time duration, peak-to-trough amplitude, distribution of phases in all cycles, and average SWR power in CA1 during the cycle. Sessions with significant differences between the distributions for low and high-SWR spindles are marked with a red asterisk; y-axis removed for display purposes, see text for details.
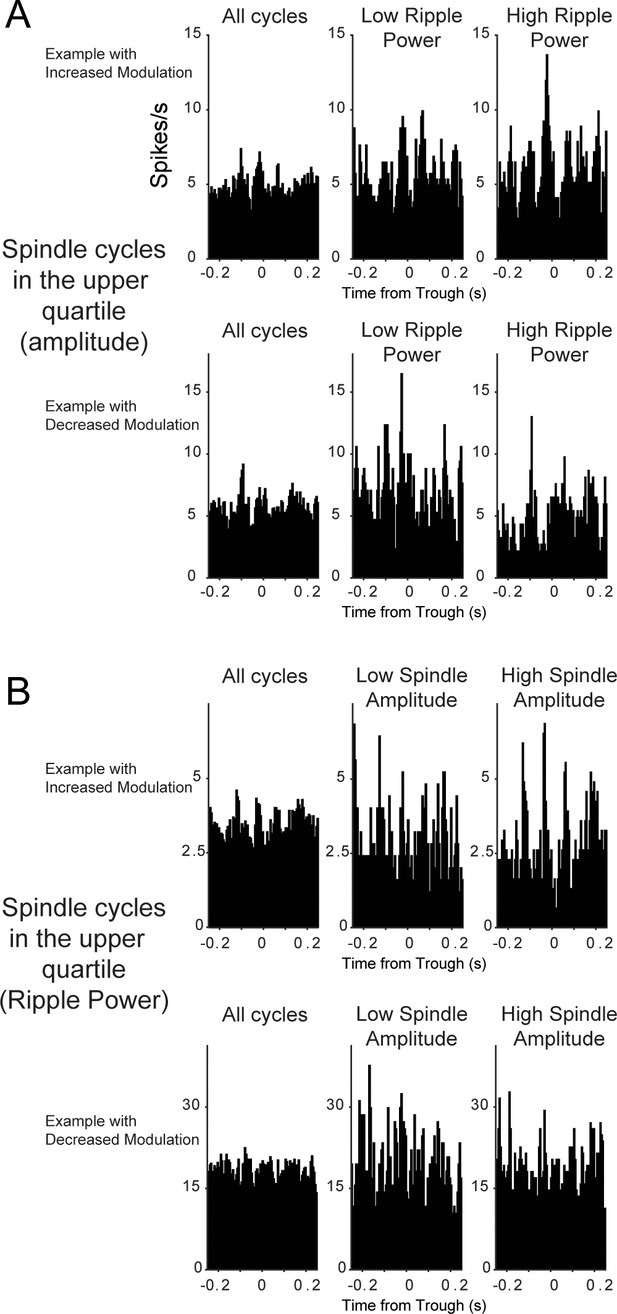
Additional examples of thalamic units with increased and decreased firing modulation with CA1 ripple power and spindle amplitude.
(A) Top row, unit with increased modulation in high ripple power spindles; bottom, unit with decreased modulation in high ripple power spindles. (B) Same for spindle amplitude: top plots, unit with increased modulation in high amplitude spindles; bottom, unit with reduced modulation with high amplitude spindles. Units in panel A and top panel B were recorded in the ventromedial nucleus, unit in B bottom, is from the paratenial-anterior medial border.
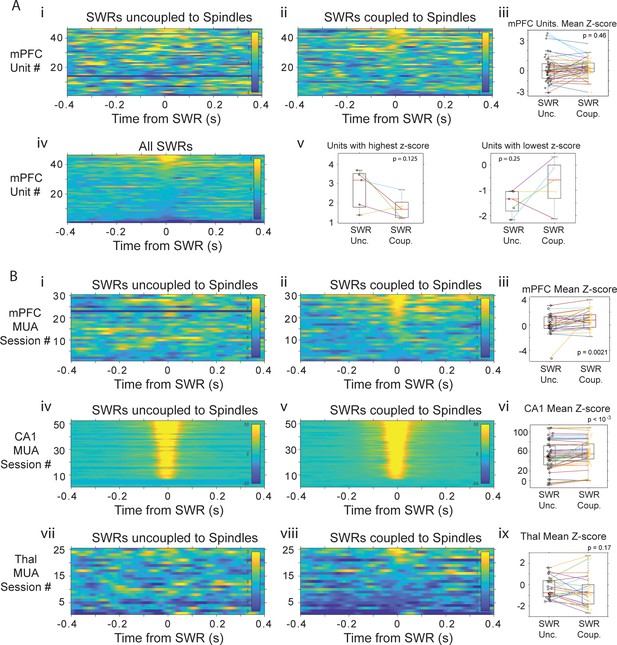
Histograms of mPFC spike activity in 800 ms windows around SWRs coupled and uncoupled to spindles.
(A) i-ii, Histograms of unit spikes for SWRs-uncoupled and coupled to spindles, each row is one unit, rows are in the same order in both panels, color code is z-score in all panels; iii, mean z-score in the −40 to 40 ms around SWRs; lines connect values for the same unit. iv, histograms for all SWRs histogram; v, units with the highest and lowest z-scores tend to have more extreme values with uncoupled SWRs. (B) Panels are organized as in A and show the multi-unit activity around SWRs uncoupled and coupled to spindles for mPFC (i-iii), CA1 (iv-vi) and thalamus (vii-ix).
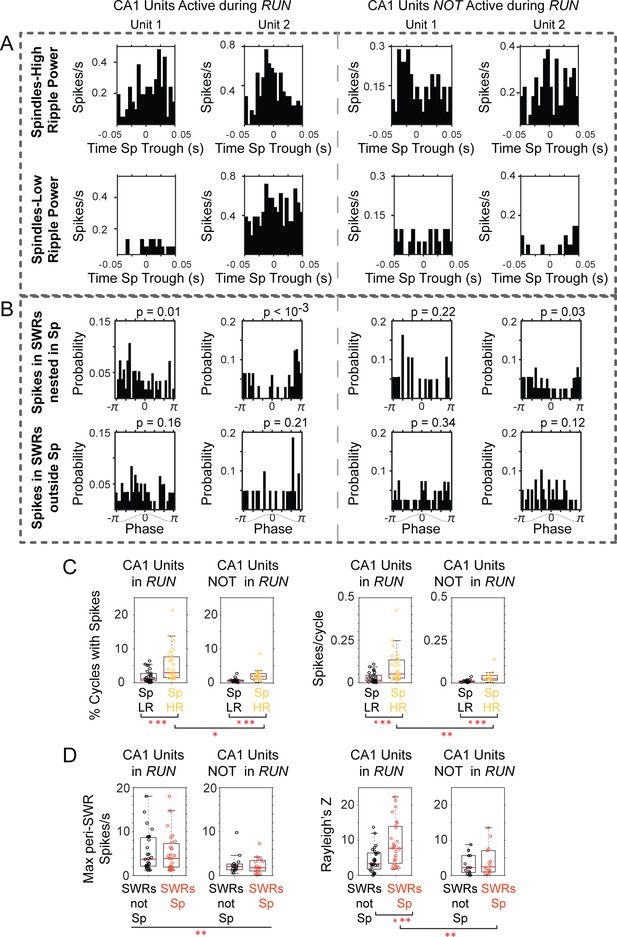
CA1 units active during ‘run’ show stronger activation in spindles with high ripple power, and firing at preferred spindle phases.
(A) Peri-spindle trough histograms of the spikes of 2 CA1 units active during exploratory behavior (left) and 2 CA1 units recorded by the same tetrode that were only active during the sleep after maze exploration (right); top plots, spindles with high ripple power; bottom plots, spindles with low ripple power (sorted clusters for these units in Figure 7—figure supplement 1). (B) Distribution of mPFC spindle phases at the time of unit spikes in SWRs nested in spindles (top) or outside (bottom); p values on top of the plots from Rayleigh test. (C) Population distributions of the percent of spindle cycles in which the CA1 units fired spikes (left panels; Sp LR = spindles with low ripple power in CA1, Sp HR = spindles with high ripple power in CA1), and the mean firing for CA1 units active or not in run (right). (D) Left, distribution of peak firing rate in the peri-SWR histograms for CA1 units active or not during run, separated by SWRs nested or not within spindle cycles (SWRs Sp vs. SWRs not Sp respectively); right, distribution of Rayleigh’s Z value suggests preferential spindle phase of CA1 units active in run when firing in SWRs nested in spindles. One asterisk indicates p<0.05, two p<0.01, three p<0.001 (non-parametric tests).
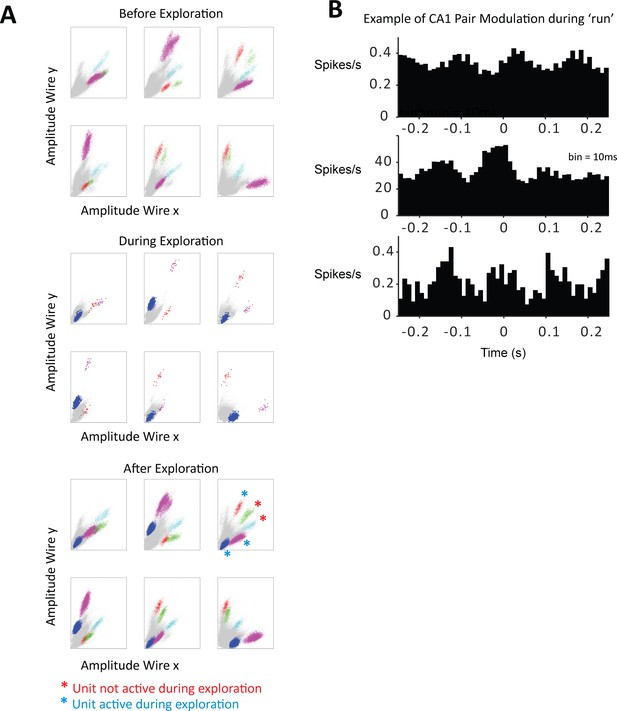
Clusters of sorted CA1 units from one tetrode and correlations during maze exploration.
(A) Three sets of six-panel plots display the peak-amplitude projections for all recorded spikes (six combinations of peak projections possible with the four wires in the tetrode). The top set of panels are the six projections obtained before exploration in the maze, the middle are the panels obtained with the data during exploration, and the bottom after exploration. Clusters of unit spikes were manually sorted, five units were identified in this session and color coded, two of them were classified as being only active after exploration on the maze (red asterisks), and three as being active both during the maze and after (blue asterisks). (B) Cross-correlograms between the spikes of three sample pairs of CA1 units while the animal explores the maze; the plots show co-modulated firing of the pairs during maze exploration in a timescale that suggests theta-related spiking.
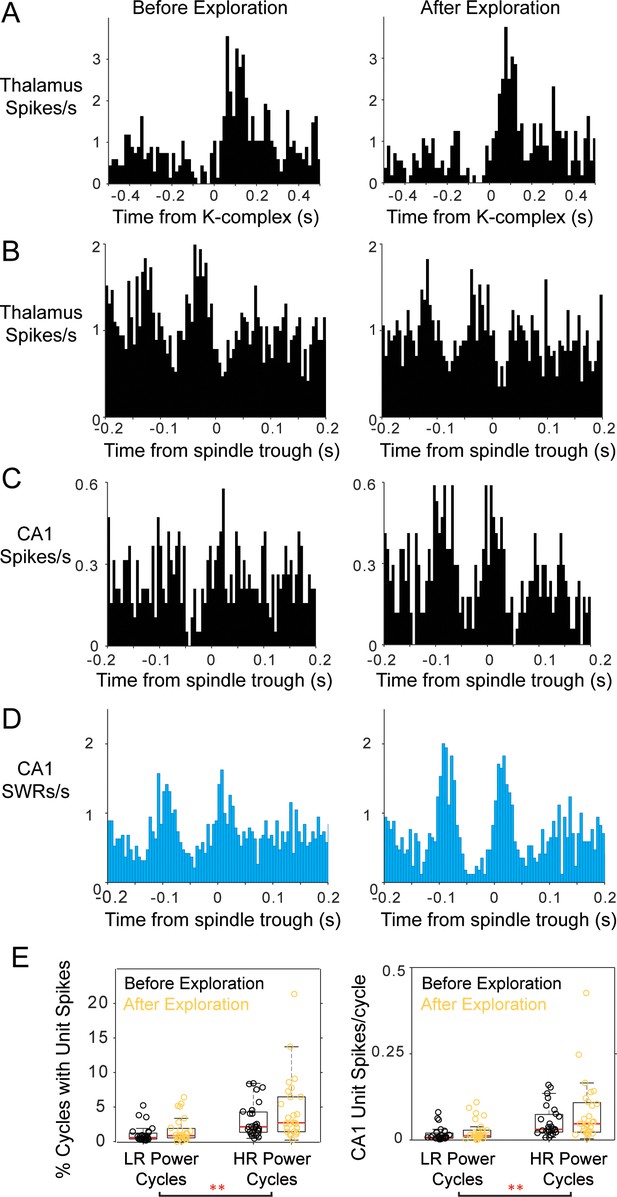
Modulation of thalamic and CA1 activity before and after free exploration on an 8-arm radial maze.
Peri-event histograms display fairly stable modulation of spiking activity in the thalamus with K-complexes before and after spatial exploration; KC-triggered histograms of thalamic spikes are shown in (A) and peri-spindle histograms in (B); instead, a CA1 unit recorded in the same session increases its modulation with spindles (C) in the sleep following behavior. (D) Spindle-triggered SWR histograms show an increase in SWR modulation following behavior. (E) Distributions of the percent of spindle cycles with CA1 unit spikes and the average number of CA1 spikes/spindle cycle before (black) and after exploration (yellow); each circle is one CA1 unit; most CA1 units increased their contribution to spindles post-behavior.
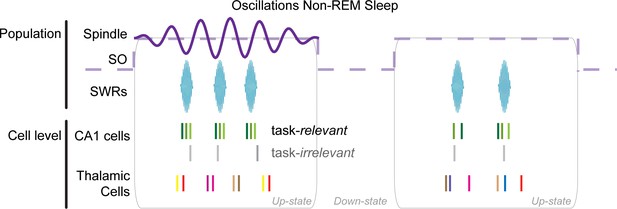
Model of spindle organization of thalamic and CA1 spike dynamics.
Task-relevant CA1 cells are preferentially engaged in SWRs and phase-locked to the spindle oscillation, coinciding with sparse co-activation of thalamic cells. The activation of different combinations of thalamic cells in subsequent spindle cycles could favor a broader range of synaptic associations at the neocortical level, while the sparsity of firing could ensure incremental synaptic changes and promote continuous learning. Each color in the spike rasters represents spikes from a different cell. SO = slow oscillation.
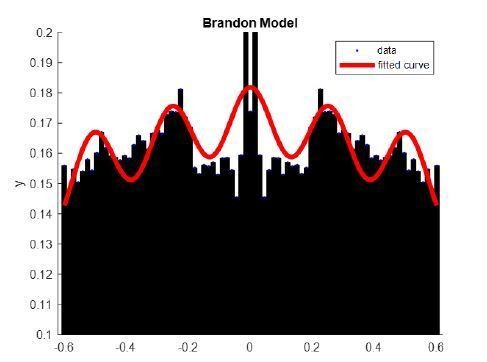
Example of cosine fit (as in Brandon et al., 2013) to the autocorrelation of a thalamic unit. X axis, time (s); y axis, correlation coefficient.

Example of peri-event histogram smoothing based on moving average (red, “new” curve), and on Gaussian smoothing (blue, “old”), used prior to calculating unit modulation index.
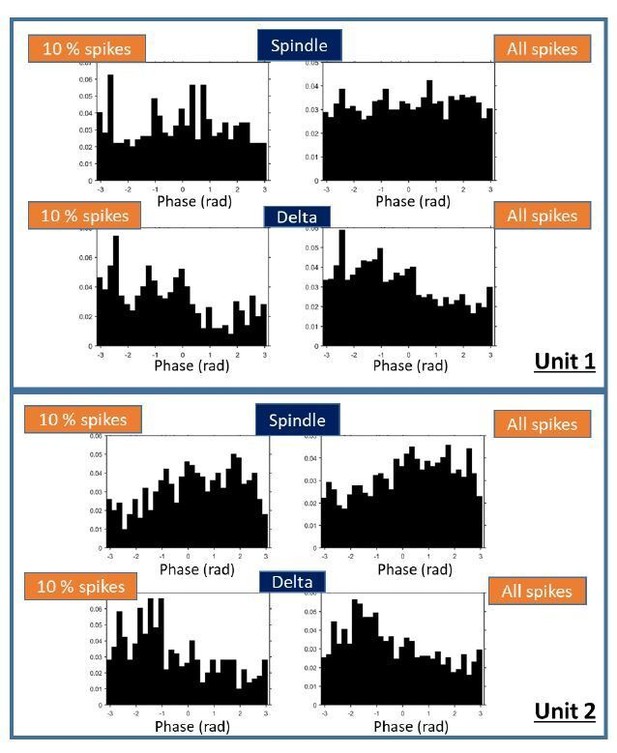
Examples of phase distributions for two thalamic units.
Distributions were calculated with 10% of the spikes (left plots) or with all spikes in sleep, as done for the results reported in the manuscript (right plots).

Cross-correlograms between spindle spikes of simultaneously recorded units in thalamus.
Y-axis indicates pair ID (each row one pair). Z-axis in spikes/s (after subtracting the mean for each pair).

Example of GLM fits for the spikes of one thalamic unit as a function of ripple (left) and spindle power (right; black dots, data, jittered on the x-axis for display purposes; red, model fit).
The fit is biased by the large number of data points with low or no spikes, which may be related to down-states and to pauses preceding burst firing (not included in this model).
Tables
Units recorded in each thalamic nucleus.
Nucleus | RE | VM | MD | Rh/CM | PT/AM | No histology | Total |
---|---|---|---|---|---|---|---|
# of units | 25 | 18 | 7 | 4 | 7 | 2 | 63 |
-
RE = reuniens; VM = ventromedial; MD = mediodorsal; Rh/CM = rhomboid –centromedian region; PT/AM = paratenial -anterior medial region.