Mapping the human subcortical auditory system using histology, postmortem MRI and in vivo MRI at 7T
Figures
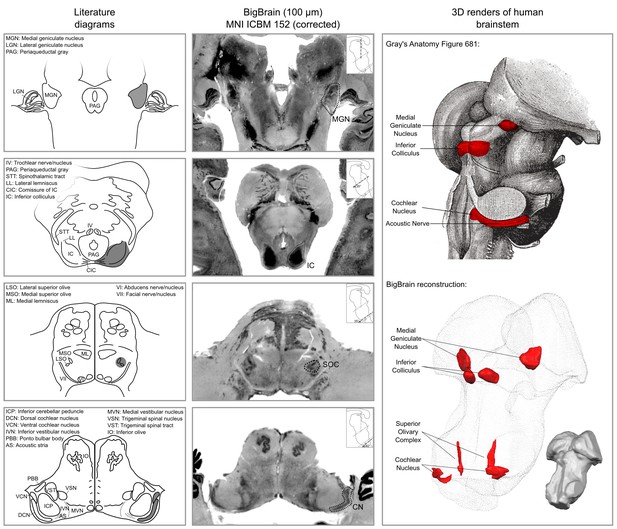
Literature diagrams (left columns) redrawn from Moore (1987) for the cochlear nucleus (CN), superior olivary complex (SOC), inferior colliculus (IC) and from the Allen Human Brain Atlas (Hawrylycz et al., 2012) for the medial geniculate body (MGB) compared to similar cuts from histology (BigBrain) in MNI (central column) and 3-D reconstructions of the segmented structures from the histology (bottom right column).
The auditory structures are highlighted in gray in the left column, by a dotted line in the central column and in red on the modified Gray’s anatomy Plate 719 (Gray and Lewis, 1918) and rendered as solid red surface meshes within the surface point cloud render of BigBrain MNI brainstem (right column). See Figure 9 for 3-D animated videos of these auditory structures.
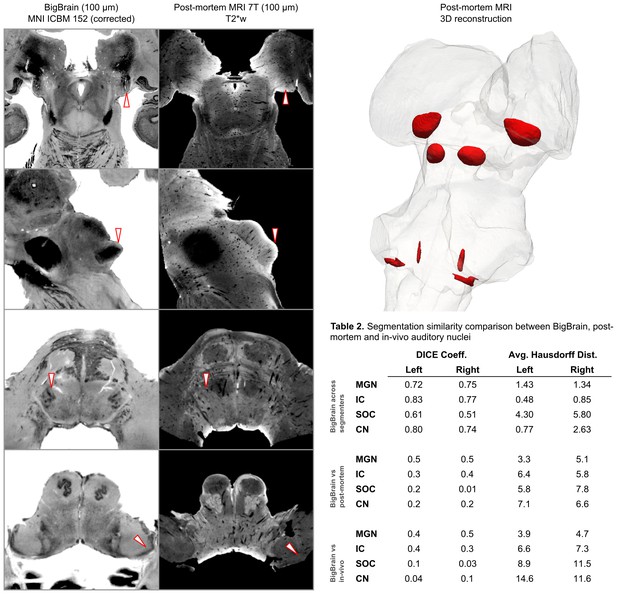
BigBrain—7T postmortem MRI image comparisons.
Histological data (BigBrain) (left column) and T2*-weighted postmortem MRI data (100 µm - central column) in MNI space. Panels from bottom to top are chosen to highlight subcortical auditory structures (CN [bottom] to MGB [top]). Arrows (white with red outline) indicate the location of the subcortical auditory nuclei. The 3-D structures resulting from the segmentation of the postmortem data is presented on the top right panel. Table 2 quantifies (using DICE coefficient and average Hausdorff distance) the agreement (in MNI space) for all subcortical structures between: (1) segmentations performed on the BigBrain dataset by the two raters (KRS and OFG) [top]; (2) segmentations obtained from the BigBrain dataset and from the post mortem MRI data [middle]; (3) segmentations obtained from the BigBrain dataset and from in vivo functional MRI data [bottom]). See Figure 9 for 3-D animated videos of these auditory structures.
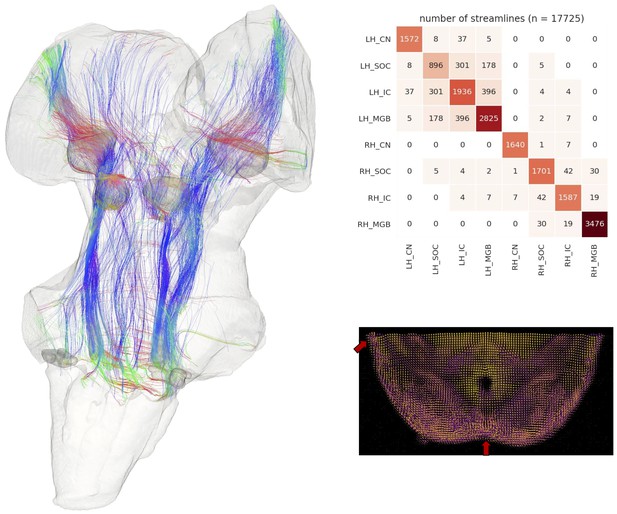
Postmortem diffusion MRI tractography.
Left: streamlines passing through subcortical auditory structures, defined from 50 µm post mortem MRI in the same specimen, warped to 200µm isotropic diffusion image space and dilated 2.5 voxels (500 µm) to include neighboring white matter. Colors represent the local orientation at each specific point along the streamline: blue is inferior-superior, red green is anterior-posterior, and red is left-right. Ten percent of streamlines are represented in this image. A rotating animation is available in the online resources. Top right: Connectivity heatmap of subcortical auditory structures. Bottom right: Diffusion orientation distribution functions (ODFs) for each voxel; axial slice at the level of the rostral inferior colliculus (IC), including the commissure of the IC (bottom center arrow) and brachium of the IC (top left arrow). A video of the streamlines is available online: https://osf.io/kmbp8/.
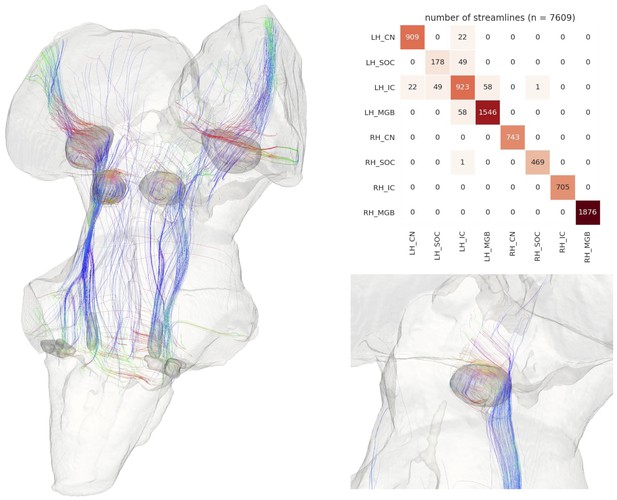
Postmortem tractography with undilated ROIs.
Postmortem human diffusion-weighted MRI tractography (from 200 µm isotropic voxels) with anatomically defined subcortical auditory seeds, downsampled to 200 µm but undilated. Streamlines that passed through manual segmentations of the medulla and optic tracts were excluded. 10% of streamlines are visualized for clarity. Top right: connectivity heatmap of subcortical auditory structures. Bottom right: Streamlines that pass through the right inferior colliculus.
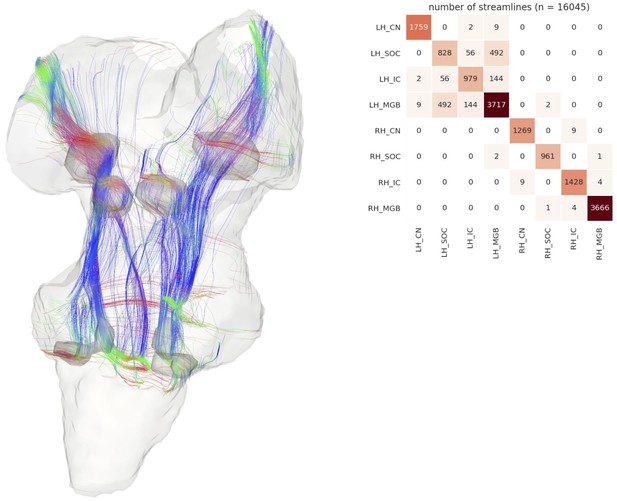
Postmortem tractography using data downsampled to in vivo resolution (1.05 mm).
Postmortem human diffusion-weighted MRI tractography with anatomically defined subcortical auditory seeds. MRI data were downsampled from 200 µm to 1050 µm to match in vivo data acquisition and then processed in the same manner as other diffusion tractography analyses. Streamlines that passed through manual segmentations of the medulla and optic tracts were excluded. 10% of streamlines are visualized for clarity. Top right: Connectivity heatmap of subcortical auditory structures.
360° rotation video of postmortem streamlines.
https://doi.org/10.7554/eLife.48932.008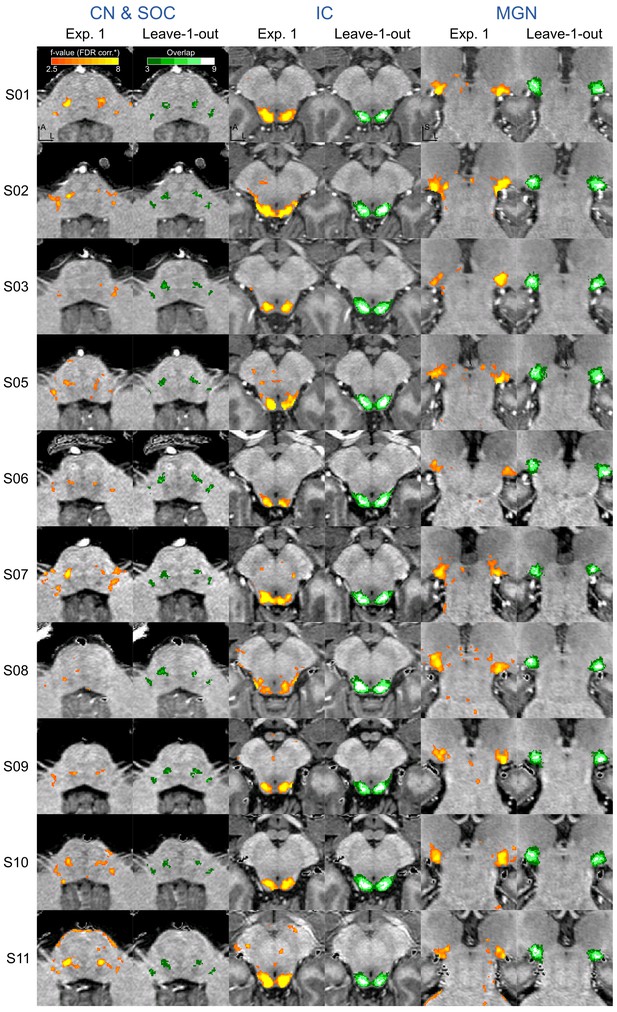
Single subject functional activation maps obtained from Experiment one thresholded for significance (FDR-q = 0.05 and p<0.001; see Materials and methods for details) and leave-one-out probabilistic functional maps highlighting voxels that are significant in at least three of the other nine subjects.
For each participant, CN/SOC and IC are shown in transversal cuts, MGB is shown in a coronal cut. See single subject videos for 3-D view of these maps in Figure 10 supplements. Unthresholded maps can be found in our online resources (see Data Availability section).
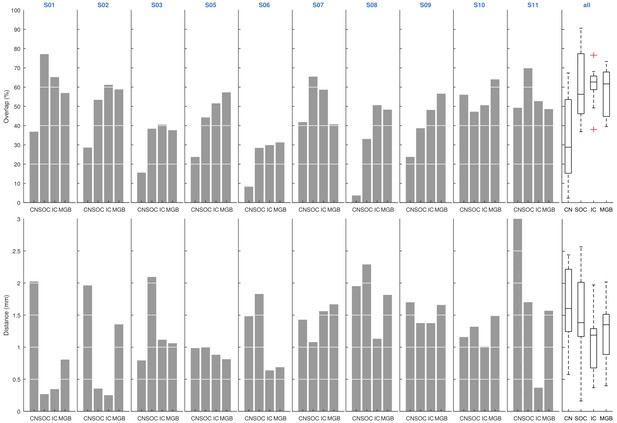
Correspondence between single subject activation maps and leave-one-out probabilistic maps.
Correspondence between single subject activation maps and leave-one-out functional probabilistic maps. Leave-one-out probabilistic functional maps are thresholded to identify voxels that are significantly responding to sounds in at least three of nine participants. The overlap represents (per region of interest) the percentage of the voxels on the leave-one-out probabilistic maps that is significantly responding to sounds in the left out subject. For each region of interest, we also report the distance in mm between the centroids of the leave-one-out probabilistic maps and the centroids of the regions significantly responding to sounds in the left out subject. The last column represents the average overlap and distance across participants per region and error bars represent the standard error across the participants.
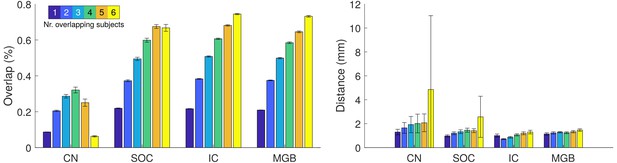
Effect of threshold on leave-one-out probabilistic maps on correspondence with single subject activations].
Correspondence between single subject activation maps and leave-one-out functional probabilistic maps at different thresholds. Leave-one-out probabilistic functional maps are thresholded to identify voxels that are significantly responding to sounds by varying thresholds from at least one of nine participants to at least six of nine participants. The overlap represents (per region of interest) the percentage of the voxels on the leave-one-out probabilistic maps that is significantly responding to sounds in the left out subject. For each region of interest, we also report the distance in mm between the centroids of the leave-one-out probabilistic maps and the centroids of the regions significantly responding to sounds in the left out subject. Boxplots represent the average overlap and distance across participants per region and error bars represent the standard error across the participants.
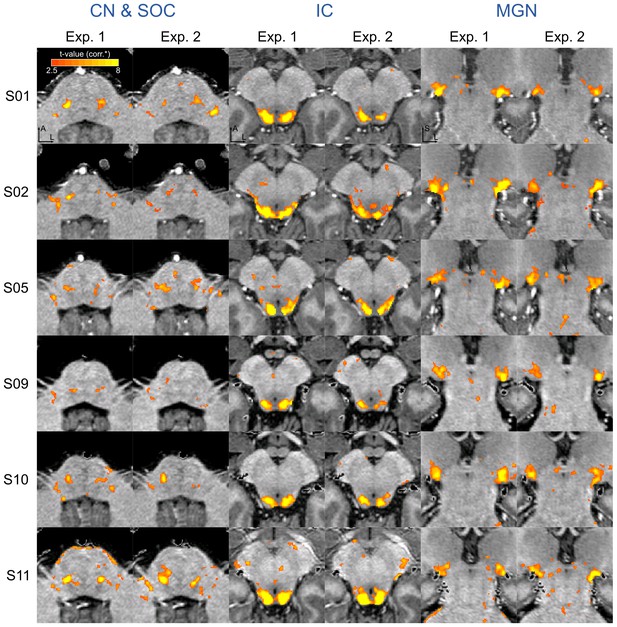
Reproducibility across experiments of the functional activation maps in six participants (also see Figure 11).
Reproducibility of functional activation maps. Functional activation maps obtained from Experiment one and Experiment 2 (six participants) thresholded for significance (FDR-q = 0.05 and p<0.001; see Materials and methods for details). For each participant, CN/SOC and IC are shown in transversal cuts, MGB is shown in a coronal cut.
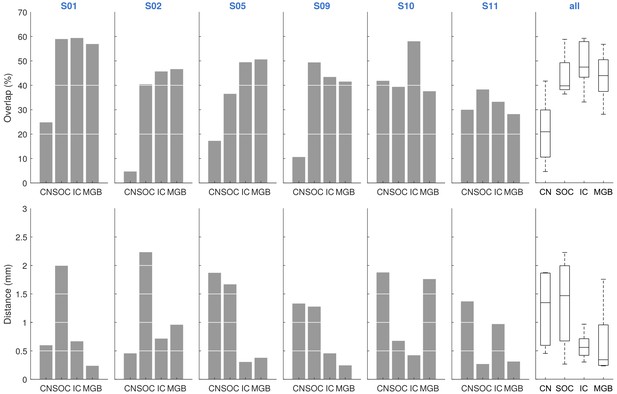
Correspondence between single subject activation maps across experiments.
Correspondence between single subject activation maps Experiment one and Experiment 2. All maps are thresholded for significance (FDR-q = 0.05 and p<0.001; see Materials and methods for details). The overlap represents (per region of interest) the percentage of the voxels significantly active in Experiment 1 that is significantly responding to sounds in Experiment 2. For each region of interest, we also report the distance in mm between the centroids of the regions significantly responding to sounds in both experiments. Videos are provided in the appendix that visualize thresholded and unthresholded maps for each of the individual participants. The last column represents the average overlap and distance across participants per region and error bars represent the standard error across the participants.
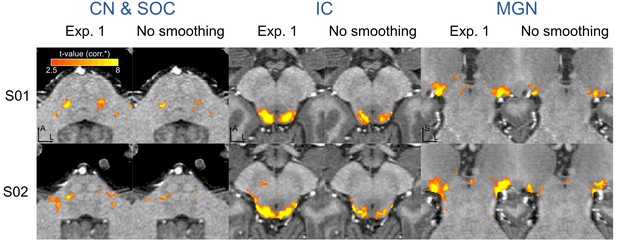
Effect of spatial smoothing in the analysis of the data collected from two of the participants.
Effect of spatial smoothing on functional activation maps. Functional activation maps obtained from Experiment one in two participants with and without applying spatial smoothing (1.5 mm FWHM Gaussian smoothing) prior to the statistical analysis. Maps are thresholded for statistical significance (FDR-q = 0.05 and p<0.001; see Materials and methods for details)). For each participant, CN/SOC and IC are shown in transversal cuts, MGB is shown in a coronal cut.
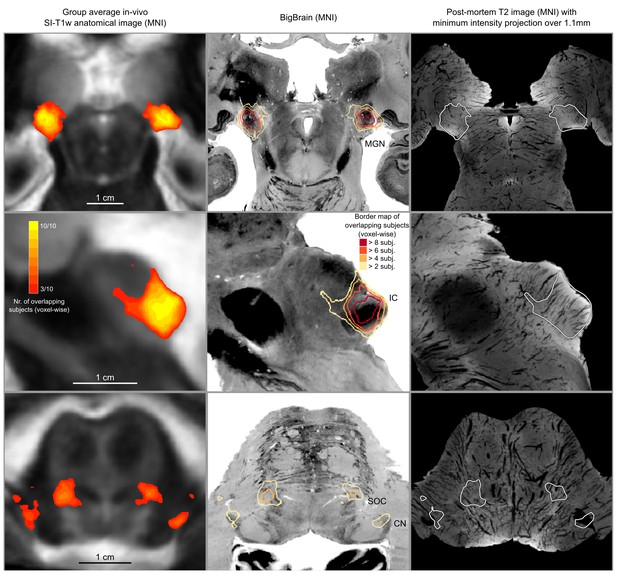
In vivo functional MRI responses to auditory stimuli, combined across 10 participants.
Left column: Conjunction of participants plotted on top of one participant’s short inversion T1-weighted anatomical MRI. Center column: Conjunction of participants’ fMRI responses warped to MNI space and plotted on top of BigBrain MNI (corrected) image. Right column: Conjunction of fMRI responses plotted on top of post mortem MRI vasculature images (1.1 mm minimum intensity projection).
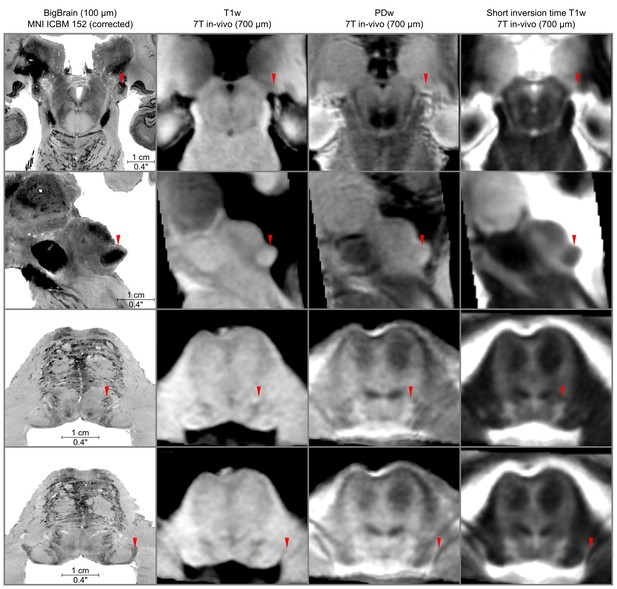
In vivo anatomical group average images in MNI space.
In vivo anatomical group average images in MNI space.
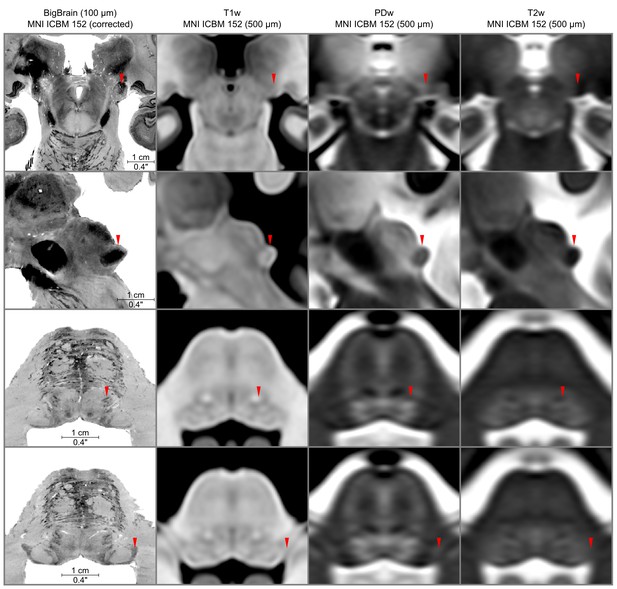
Anatomical images from MNI ICBM 152 compared to BigBrain in MNI space Anatomical images from MNI ICBM 152 2009b dataset compared to BigBrain histology in MNIspace (left column).
https://doi.org/10.7554/eLife.48932.017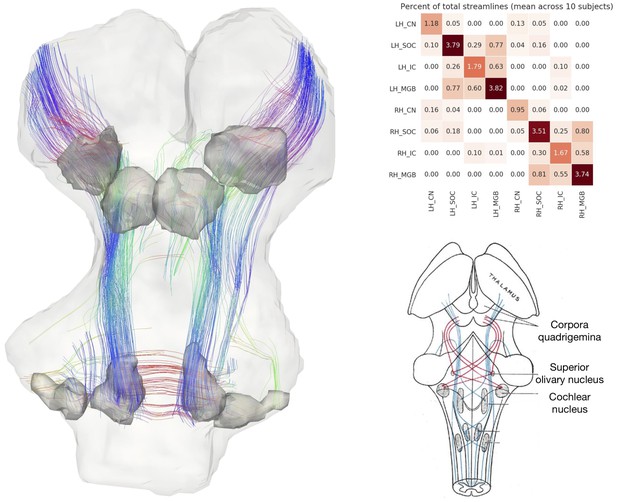
In vivo tractography of the subcortical auditory system from 7T diffusion-weighted MRI.
Left: 3-D images from one participant. Fiber orientation distribution functions were estimated from diffusion-weighted MRI images of the brainstem and were used for deterministic tractography. Streamlines that passed through functionally defined auditory ROIs (dark grey) are shown here (excluding streamlines through the medulla). Colors represent the local orientation at each specific point along the streamline: blue is inferior-superior, red green is anterior-posterior, and red is left-right. A rotating animation is available in the online resources. Top right: connectivity between subcortical auditory ROIs as a percentage of total brainstem streamlines, averaged over 10 participants. Bottom right: schematic of auditory brainstem connectivity from Gray’s Anatomy of the Human Body. A video of the streamlines is available online: https://osf.io/ykd24/.
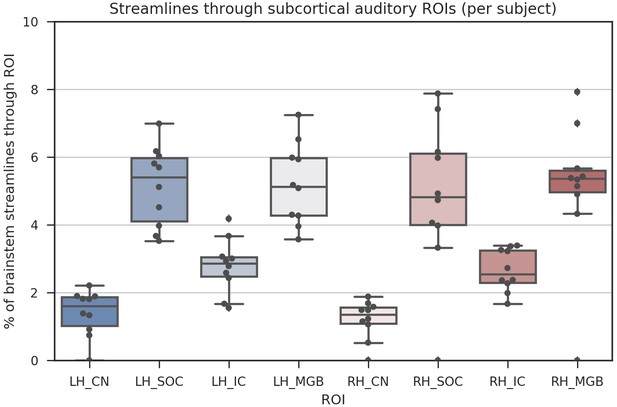
Bar plot of streamline counts through each ROI.
Diffusion-weighted MRI tractography streamlines passing through each subcortical auditory region of interest for the ten in vivo participants. Bars represent 95% confidence intervals.
360° rotation video of in vivo streamlines.
https://doi.org/10.7554/eLife.48932.020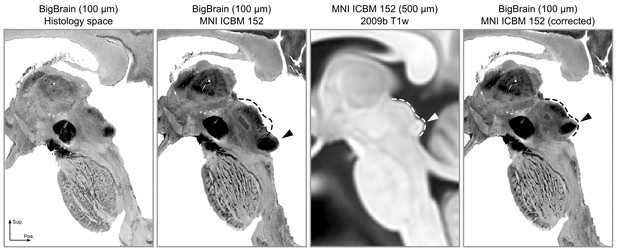
The registration error around the inferior colliculus is visible bilaterally when comparing Panel 2 and Panel 3.
The dashed lines indicate the correct shape (and location) of the colliculi in MNI space. The arrows point to the inferior colliculus (IC). The last panel shows the corrected BigBrain MNI dataset.
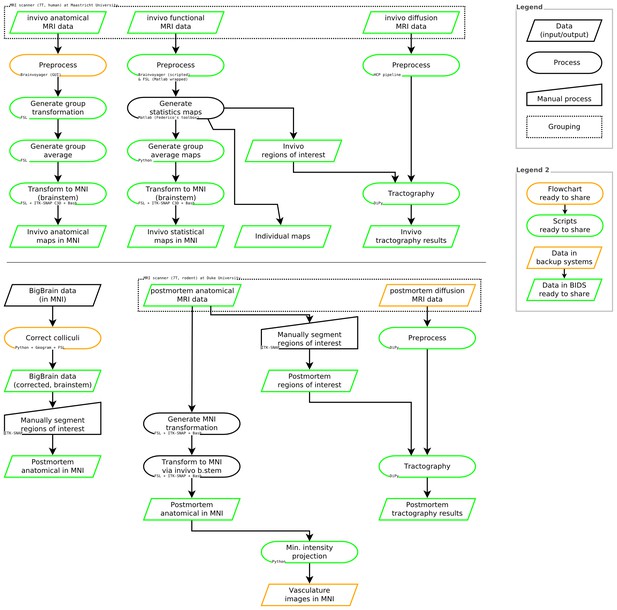
Summary of data processing steps, including availability of data and code.
https://doi.org/10.7554/eLife.48932.022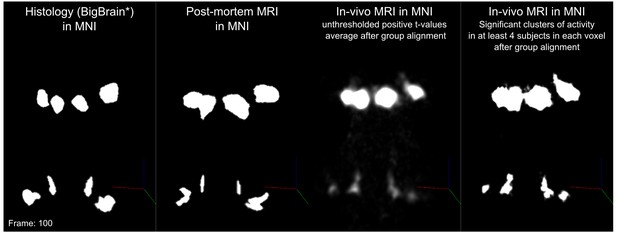
One frame of volume rendered animations for comparing histology (BigBrain), post-mortem MRI, in-vivo MRI unthresholded positive t-values group average and in-vivo MRI clusters of significant activity overlapping in at least four subjects in each voxel.
https://doi.org/10.7554/eLife.48932.0233D volume rendered comparisons in MNI space.
https://doi.org/10.7554/eLife.48932.024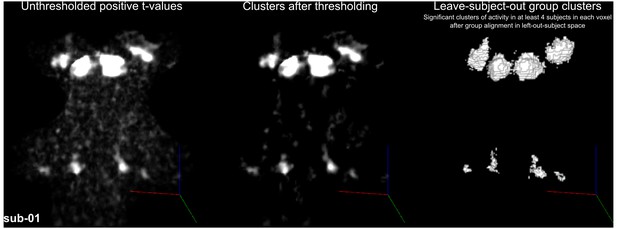
One frame of volume rendered animations for single subject statistical maps.
(Left)positive t-values (middle) after thresholding (right) leave-one-out probabilistic map ()). Viewing angle here is similar to Figure 1.
Subject 01.
https://doi.org/10.7554/eLife.48932.026Subject 02.
https://doi.org/10.7554/eLife.48932.027Subject 03.
https://doi.org/10.7554/eLife.48932.028Subject 05.
https://doi.org/10.7554/eLife.48932.029Subject 06.
https://doi.org/10.7554/eLife.48932.030Subject 07.
https://doi.org/10.7554/eLife.48932.031Subject 08.
https://doi.org/10.7554/eLife.48932.032Subject 09.
https://doi.org/10.7554/eLife.48932.033Subject 10.
https://doi.org/10.7554/eLife.48932.034Subject 11.
https://doi.org/10.7554/eLife.48932.035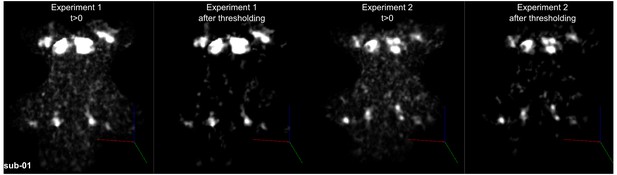
One frame of volume rendered animations for Subject 01 statistical maps (experiment 1 positive t-values and thresholded (col 1–2) and experiment 2 positive t-values and thresholded (col 3–4)).
Viewing angle here is similar to Figure 1.
Subject 01 experiment 1 vs experiment 2.
https://doi.org/10.7554/eLife.48932.037Subject 02 experiment 1 vs experiment 2.
https://doi.org/10.7554/eLife.48932.038Subject 05 experiment 1 vs experiment 2.
https://doi.org/10.7554/eLife.48932.039Subject 09 experiment 1 vs experiment 2.
https://doi.org/10.7554/eLife.48932.040Subject 10 experiment 1 vs experiment 2.
https://doi.org/10.7554/eLife.48932.041Subject 11 experiment 1 vs experiment 2.
https://doi.org/10.7554/eLife.48932.042Group average (N=6) unthresholded positive t-values for experiment 1 vs experiment 2.
https://doi.org/10.7554/eLife.48932.043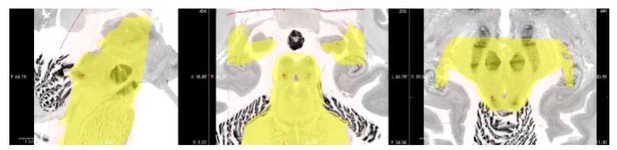
Underlay image: BigBrain histology in MNI152 space.
Yellow overlay: mask of post mortem anatomical MRI in MNI152 space.
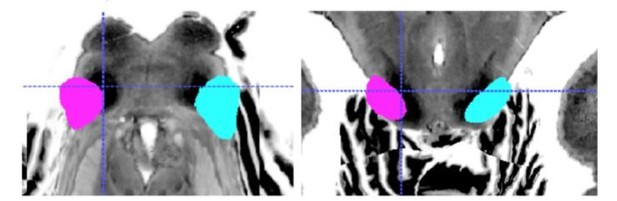
Underlay image: BigBrain histology in MNI152 space.
Segmentation overlay: conjunction of two raters’ IC segmentations based on post mortem anatomical MRI (conducted in native space) in MNI152 space.
Videos
Group average fMRI results from two separate experiments.
https://doi.org/10.7554/eLife.48932.047In vivo probabilistic tractography of the subcortical auditory system in one individual.
https://doi.org/10.7554/eLife.48932.050Tables
Comparisons between the volume (mm3) of auditory subcortical structures reported in the literature (Glendenning and Masterton, 1998) and the volume obtained in our BigBrain segmentation (in MNI space), post mortem MRI data segmentation and in vivo functional clusters (defined based on voxels that are significant in at least three, four, or five participants out of the 10 included in Experiment 1).
https://doi.org/10.7554/eLife.48932.004Literature | BigBrain | Post mortem | In vivo (thr=3) | In vivo (thr=4) | In vivo (thr=5) | |
---|---|---|---|---|---|---|
CN | 46 | 32 | 11 | 54 | 24 | 11 |
SOC | 7 | 6 | 4 | 124 | 63 | 29 |
IC | 65 | 63 | 73 | 263 | 189 | 146 |
MGN | 58 | 75 | 134 | 304 | 207 | 152 |
Additional files
-
Transparent reporting form
- https://doi.org/10.7554/eLife.48932.044