Symbiosis: Algae and fungi move from the past to the future
Ask a biology student to describe an association between algae and fungi and they will surely explain to you how fungal structures called hyphae can surround algal cells to form a completely new organism with its own metabolism called a lichen (Honegger, 1991; Figure 1A). Thanks to nutritional exchanges between the alga and the fungus, and adaptive mechanisms that date back some 415 million years, lichens can survive in the most extreme environments.
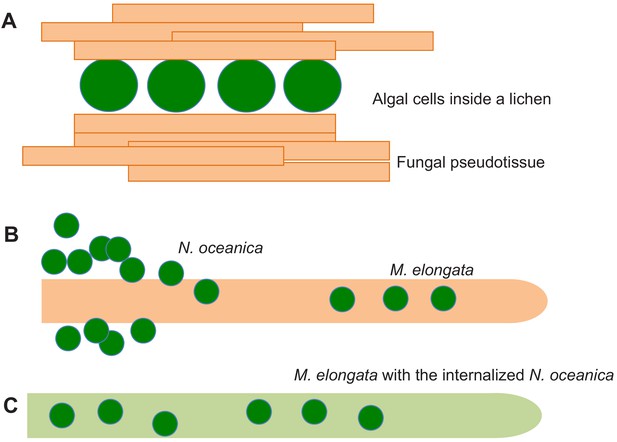
Fungal-algal symbiosis.
(A) In lichens the algal cells (green) are surrounded by fungal hyphae (orange) to form a new organism with its own metabolism and properties. Fungal hyphae aggregate to produce fungal pseudotissues. When the alga N. oceanica grows in the presence of a soil fungus called M. elongata, the algae first aggregate and make contact with the surface of a hypha (B). Eventually the algae enter the hypha, which changes color to green due to the presence of the algae, which are photosynthetically active, inside it (C).
In all known interactions between algae and fungi, the algal cells remain outside the hyphae of the fungus. Now, in eLife, Christoph Benning, Gregory Bonito and co-workers – including Zhi-Yan Du of Michigan State University as first author – report how, under certain conditions, algal cells can enter the fungus (Du et al., 2019). The experiments were performed with Nannochloropsis oceanica, an algal species that lives in marine and fresh water, and Mortierella elongata, a fungus that lives in soil. Isotope tracer experiments revealed the exchange of nutrients, including carbon and nitrogen, between the two partners. Moreover, both remained physiologically active over two months of co-cultivation, with the algal cells continuing to grow, divide and remain photosynthetically active within the hyphae (Figure 1B,C).
Symbioses between microbes and plants or animals are often used as examples of trans-kingdom co-evolution: fossils provide direct evidence of symbiosis happening in the past, and phylogenetic analyses can reveal when the symbiotic partners appeared (Lutzoni et al., 2018; Kohler et al., 2015). For example, fossils that have been dated to the Devonian era (about 450 million years ago) reveal fungal colonization patterns that are very similar to those produced by Glomeromycotina today (Remy et al., 1994): these obligate symbionts (that is, symbionts that rely on a host to survive) enter the root cells of plants to form structures called arbuscules in a widespread form of symbiosis that is now called arbuscular mycorrhiza.
However, fossils and phylogenetics cannot tell us how the various forms of symbiosis that we see today were actually formed. Phylogenetic analyses support the idea that Glomeromycotina are members of the Mucoromycota, an early diverging fungal phylum (Spatafora et al., 2016), as is Mortierella. However, it is not clear if the fungi that are responsible for arbuscular mycorrhiza today evolved from saprotrophic fungi (which feed on dead or decaying matter), since there is no evidence for such fungi evolving to become obligate symbionts. By contrast, elegant experiments on the evolution of nitrogen-fixing bacteria are available: the transfer of the sym plasmid to a Ralstonia strain clearly demonstrates that a pathogen can evolve into a symbiotic bacterium (Clerissi et al., 2018).
The study of Du et al. builds on previous work which showed that the single-cell green alga Chlamydomonas reinhardtii and yeasts can interact under specific physiological conditions (Hom and Murray, 2014). However, the fact that N. oceanica and M. elongata mainly maintain their phenotype when they are co-cultivated is rather surprising. By contrast, the fungi in lichen aggregate and give rise to pseudotissues in which algal cells (and also bacteria) become embedded (Cardinale et al., 2008; Figure 1A).
The phenomenon observed by Du et al. started with the N. oceanica flocculating (that is, clumping together) around the fungus. An obvious question is: what signal causes the process of flocculation to begin? In particular, does N. oceanica detect and react to molecules released by M. elongata? Since the genomes of both partners have been sequenced, various transcriptomic and metabolomics approaches should help researchers to answer these questions.
It will also be interesting to explore if MEP α, a gene that codes for a protein that transports ammonia (NH3) in plants and bacteria, has a role in the exchange of nitrogen between N. oceanica and M. elongata. It is known that the MEP α gene was transferred from prokaryotes to the Leotiomyceta, which are ancestors of the fungi found in lichens, and also to the green plants (Lutzoni et al., 2018), and it is still found in all such fungi and plants. Mortierella and Glomeromycotina are both very rich in lipids (which contain lots of carbon), but the latter cannot synthesize lipids, relying instead on their plant host to supply them. The type of lipid exchange observed in N. oceanica and M. elongata could have applications in biotechnology. Indeed, Du et al. have shown previously that using genetic techniques to overexpress a gene called DGTT5 leads to increased lipid accumulation in M. elongata, which could increase the output of microalgal biofuels from this system (Du et al., 2018).
Finally, it is known that M. elongata is a host for various types of endobacteria (Uehling et al., 2017; Desirò et al., 2018). Since these bacteria are distinctive evolutionary markers of the ancient fungi Mucoromycota (Bonfante and Desirò, 2017), learning more about M. elongata may also help us to understand the biological properties of Mucoromycota that make them prone to invasion by both prokaryotes and eukaryotes.
References
-
Functional aspects of the lichen symbiosisAnnual Review of Plant Physiology and Plant Molecular Biology 42:553–578.https://doi.org/10.1146/annurev.pp.42.060191.003005
-
Contemporaneous radiations of fungi and plants linked to symbiosisNature Communications 9:5451.https://doi.org/10.1038/s41467-018-07849-9
-
Comparative genomics of Mortierella elongata and its bacterial endosymbiont Mycoavidus cysteinexigensEnvironmental Microbiology 19:2964–2983.https://doi.org/10.1111/1462-2920.13669
Article and author information
Author details
Publication history
Copyright
© 2019, Bonfante
This article is distributed under the terms of the Creative Commons Attribution License, which permits unrestricted use and redistribution provided that the original author and source are credited.
Metrics
-
- 6,580
- views
-
- 430
- downloads
-
- 10
- citations
Views, downloads and citations are aggregated across all versions of this paper published by eLife.
Download links
Downloads (link to download the article as PDF)
Open citations (links to open the citations from this article in various online reference manager services)
Cite this article (links to download the citations from this article in formats compatible with various reference manager tools)
Further reading
-
- Ecology
- Microbiology and Infectious Disease
Interspecies interactions involving direct competition via bacteriocin production play a vital role in shaping ecological dynamics within microbial ecosystems. For instance, the ribosomally produced siderophore bacteriocins, known as class IIb microcins, affect the colonization of host-associated pathogenic Enterobacteriaceae species. Notably, to date, only five of these antimicrobials have been identified, all derived from specific Escherichia coli and Klebsiella pneumoniae strains. We hypothesized that class IIb microcin production extends beyond these specific compounds and organisms. With a customized informatics-driven approach, screening bacterial genomes in public databases with BLAST and manual curation, we have discovered 12 previously unknown class IIb microcins in seven additional Enterobacteriaceae species, encompassing phytopathogens and environmental isolates. We introduce three novel clades of microcins (MccW, MccX, and MccZ), while also identifying eight new variants of the five known class IIb microcins. To validate their antimicrobial potential, we heterologously expressed these microcins in E. coli and demonstrated efficacy against a variety of bacterial isolates, including plant pathogens from the genera Brenneria, Gibbsiella, and Rahnella. Two newly discovered microcins exhibit activity against Gram-negative ESKAPE pathogens, i.e., Acinetobacter baumannii or Pseudomonas aeruginosa, providing the first evidence that class IIb microcins can target bacteria outside of the Enterobacteriaceae family. This study underscores that class IIb microcin genes are more prevalent in the microbial world than previously recognized and that synthetic hybrid microcins can be a viable tool to target clinically relevant drug-resistant pathogens. Our findings hold significant promise for the development of innovative engineered live biotherapeutic products tailored to combat these resilient bacteria.
-
- Ecology
For the first time in any animal, we show that nocturnal bull ants use the exceedingly dim polarisation pattern produced by the moon for overnight navigation. The sun or moon can provide directional information via their position; however, they can often be obstructed by clouds, canopy, or the horizon. Despite being hidden, these bodies can still provide compass information through the polarised light pattern they produce/reflect. Sunlight produces polarised light patterns across the overhead sky as it enters the atmosphere, and solar polarised light is a well-known compass cue for navigating animals. Moonlight produces an analogous pattern, albeit a million times dimmer than sunlight. Here, we show evidence that polarised moonlight forms part of the celestial compass of navigating nocturnal ants. Nocturnal bull ants leave their nest at twilight and rely heavily on the overhead solar polarisation pattern to navigate. Yet many foragers return home overnight when the sun cannot guide them. We demonstrate that these bull ants use polarised moonlight to navigate home during the night, by rotating the overhead polarisation pattern above homing ants, who alter their headings in response. Furthermore, these ants can detect this cue throughout the lunar month, even under crescent moons, when polarised light levels are at their lowest. Finally, we show the long-term incorporation of this moonlight pattern into the ants’ path integration system throughout the night for homing, as polarised sunlight is incorporated throughout the day.