Sperm chemotaxis is driven by the slope of the chemoattractant concentration field
Figures
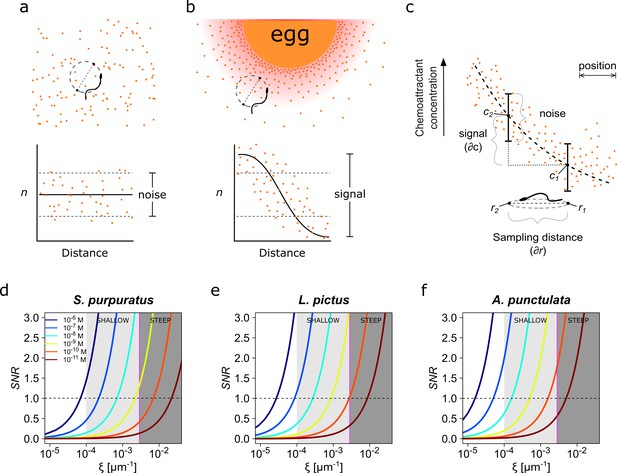
Physics of chemoreception.
(a) A spermatozoon swimming in an isotropic chemoattractant concentration field, where the number of molecules detected (n) is within the noise of detection. (b) A spermatozoon swimming near to an egg, while chemoattractant molecules are diffusing from its surrounding jelly layer creating a chemoattractant gradient. Note that the signal detected in this case is larger than the detection noise. (c) The assessment of a chemoattractant concentration gradient requires that the signal difference between two sampled positions must be greater than the noise. (d–f) The signal-to-noise ratio in the determination of the chemoattractant gradient SNR plotted against the relative slope of the chemoattractant concentration gradient in log scale, , for different chemoattractant concentrations of speract for either S. purpuratus (d), or L. pictus (e) spermatozoa, and of resact for A. punctulata (f) spermatozoa (see Supplementary file 1 for the list of parameter values taken in consideration for panels d–f). S. purpuratus spermatozoa have lower capacity of detection for the same chemoattractant concentrations at a given than L. pictus and A. punctulata. The tone of the shaded areas indicates shallow or steep gradient conditions. The horizontal dotted line represents SNR = 1; the vertical magenta line represents = 2.6 x 10−3 µm−1. Colors of the line traces (from blue to brown) indicate distinct chemoattractant concentrations in the range [10−6 – 10−11 M].
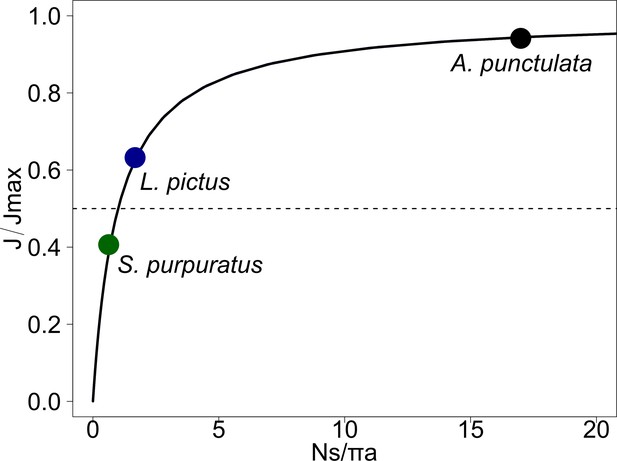
Chemoattractant diffusive currents have a non-linear relationship to receptor coverage.
For a spherical cell of radius a, with N disk-like receptors of effective radius s, the diffusive current saturates for N >> N1/2 = πa⁄s (N1/2 is highlighted for S. purpuratus, L. pictus and A. punctulata with a green, blue and black dots, respectively). For S. purpuratus, however, the number of receptors is smaller than N1/2 and the diffusive influx falls into an almost linear regime.
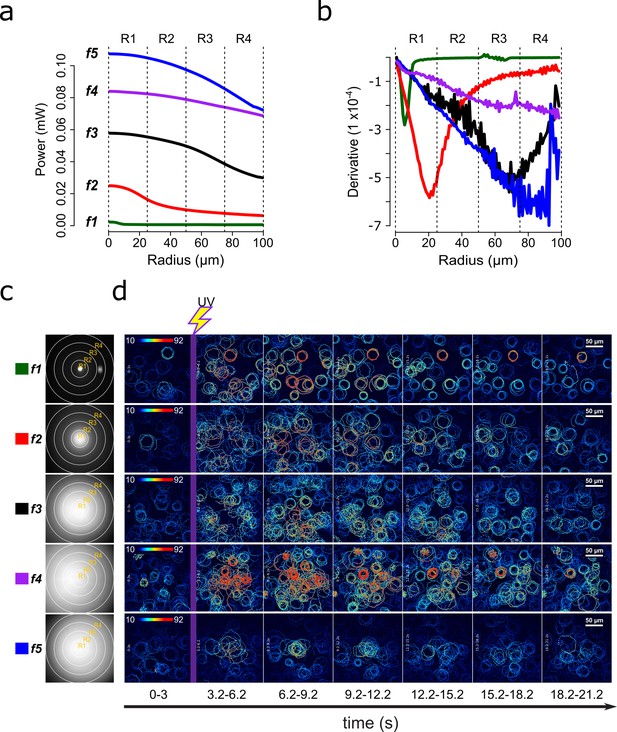
Screening of speract concentration gradients.
(a) Radial profile and its derivative (b) of the UV light scattered at the glass-liquid interface for each optical fiber (f1–f5). (c) Spatial distribution of the UV flash energy for each fiber. (d) Representative motility and [Ca2+]i responses of S. purpuratus spermatozoa exposed to different concentration gradients of speract. F-F0 time projections, showing spermatozoa head fluorescence at 3 s intervals before and after photoactivation of 10 nM caged speract in artificial seawater with 200 ms UV flash. The pseudo-color scale represents the relative fluorescence of fluo-4, a [Ca2+]i indicator, showing maximum (red) and minimum (blue) relative [Ca2+]i. Scale bars of 50 µm.
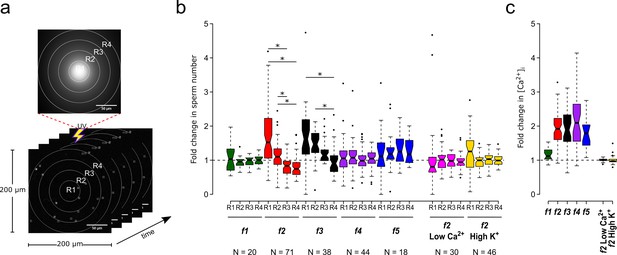
Motility and [Ca2+]i responses of S. purpuratus spermatozoa exposed to specific concentration gradients of speract.
(a) The positions of the sperm heads within the imaging field are automatically assigned to either R1, R2, R3 or R4 concentric regions around the centroid of the UV flash intensity distribution. Each ROI was also used to obtain the sperm head fluorescence from the raw video microscopy images (as the mean value of the ROI) (see Figure 3—figure supplement 1). Scale bar of 50 µm. (b) Fold change in sperm number, defined as the number of spermatozoa at the peak of the response (6 s) relative to the mean number before speract stimulation (0–3 s) (see Figure 3—figure supplement 2). (c) Relative changes in [Ca2+]i experienced by spermatozoa at the peak response (6 s) after speract stimulation. Negative controls for spermatozoa chemotaxis are artificial seawater with nominal Ca2+ (Low Ca2+); and artificial seawater with 40 mM of K+ (High K+). Both experimental conditions prevent chemotactic responses by inhibiting the Ca2+ membrane permeability alterations triggered by speract; the former disrupts the Ca2+ electrochemical gradient, and the later disrupt the K+ electrochemical gradient required as electromotive force needed to elevate pHi, and to open Ca2+ channels. The central line in each box plot represents the median value, the box denotes the data spread from 25% to 75%, and the whiskers reflect 10–90%. The number of experiments is indicated on the bottom of each experimental condition. We used the same number of experiments for the relative change in [Ca2+]i (right panel). *Statistical significance, p<0.05; multiple comparison test after Kruskal-Wallis.
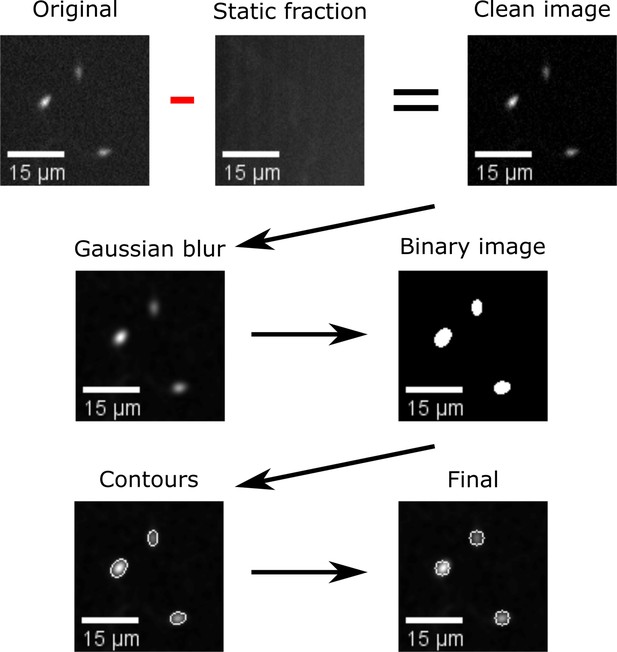
Automatic segmentation of swimming spermatozoa.
Work-flow of the segmentation algorithm: Video microscopy images were background subtracted by removing the temporal average intensity projection (static fraction) of the un-stimulated frames (93 frames = 3 s), from the whole video (25 s). The resulting images were convolved with a low-pass spatial frequency filter to reduce noise (detector, electronic, shot). The resulting images were thresholded to generate arrays of regions of interest (ROIs), a heuristic search for connected components was then applied to label single ROIs and to assign the corresponding pixels to unique spermatozoa. Scale bar of 15 µm.
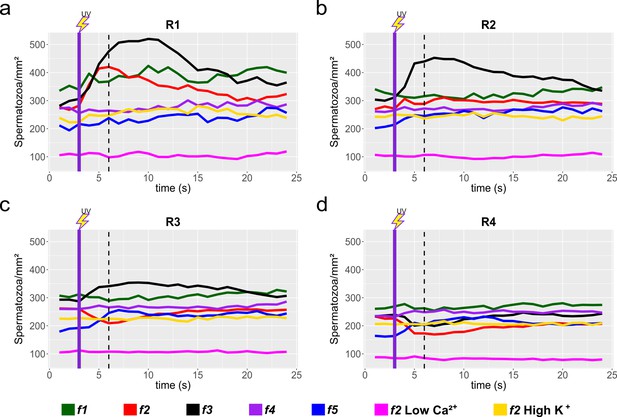
Sperm response to speract photo-release, collated data from individual experiments.
Sperm motility responses to different speract concentration gradients (f1, f2, f3, f4, f5) at R1 (a), R2 (b), R3 (c) and R4 (d) concentric regions (see Figure 3a). Negative controls for sperm chemotaxis are artificial sea water with nominal 0 Ca2+ (Low Ca2+); and artificial sea water with 40 mM of K+ (High K+). Each time trace represents the mean sperm density from up to 20 video microscopy experiments. Note that peak responses occurred around 6 s (shown by the vertical dashed lines), some 3 s after speract exposure (indicated as vertical dotted lines). Purple vertical line indicates the UV flash (200 ms).
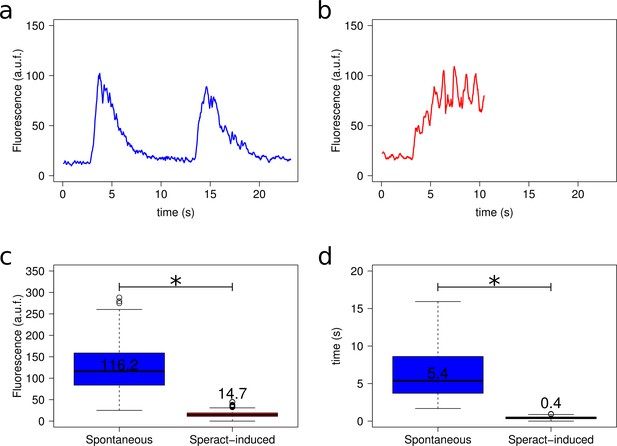
Spontaneous vs. speract-induced [Ca2+]i oscillations.
(a) Example of a spontaneous Ca2+ oscillation (two oscillations). Only 20% of sperm experiencing spontaneous oscillations suffer more than one oscillation, most of them experience only one [Ca2+]i increase. (b) Representative trace of speract-induced oscillations. Caged-speract was released after third second. (c) Comparison of Ca2+ oscillation amplitude between spontaneous (blue) vs. speract-induced (red) oscillations. n = 75 for spontaneous and n = 56 for speract-induced oscillations. (d) Comparison of Ca2+ oscillation period between spontaneous (blue) vs. speract-induced (red) oscillations. n = 16 for spontaneous and n = 56 for speract-induced oscillations. *Statistical significance, p<0.01, Mann-Whitney U test. [Ca2+]i oscillation traces (panels a and b) were smoothed using an average filter with a four frame window in a 30.8 fps setting. For further information see Extended Materials and methods, section 2.8. Spontaneous vs. speract-induced [Ca2+]i oscillations.
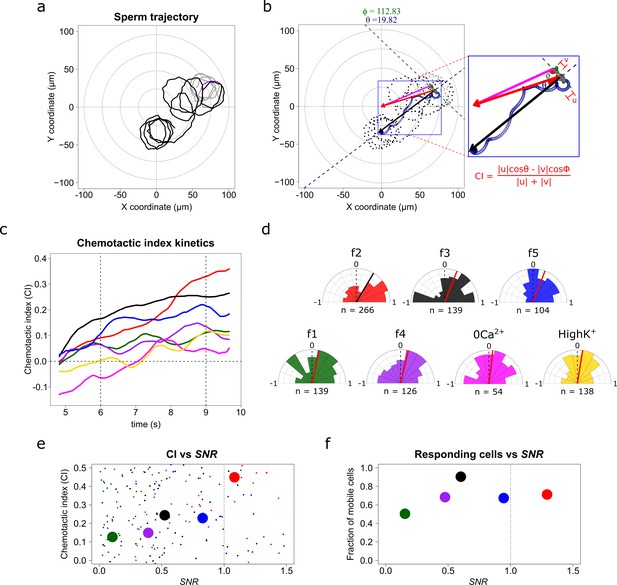
S. purpuratus spermatozoa selectively experience chemotaxis toward specific speract gradients.
(a) Sperm trajectory before (gray) and after (black) the UV irradiation (purple). (b) Definition of a chemotactic index to score chemotactic responses. Dots represent sperm trajectory before (gray) and after (black) UV irradiation. Green and blue empty spirals indicate the smoothed trajectory before and after UV irradiation. Gray and black vectors are the progressive sperm displacement before and after stimulation, respectively; and the v and u vectors are the linear speed before and after stimulation; and ϕ and θ are the angles to their corresponding reference vectors to the center of the imaging field – the highest UV irradiated area, (magenta and red, respectively). Chemotactic index (CI) is defined as in the inset (see also Video 2). (c) Temporal evolution of the chemotactic index. Functions were calculated from the median obtained from sperm trajectories of each of f1, f2, f3, f4, f5, f2-ZeroCa2+, and f2-HighK+ experimental conditions (Appendix 1—video 7). (d) Radial histograms of CI computed at second 9 (vertical dotted line at panel c). Significant differences (Binomial test, p-value<0.05) were observed only for f2, f3 and f5 fibers, compared to controls. n represents the number of individual sperm trajectories analyzed. (e) CI as a function of the signal-to-noise ratio (SNR). Each parameter was calculated for single cells. Large filled points represent the median for each gradient condition distribution. (f) Fraction of responding cells as a function of the SNR (spermatozoa whose effective displacement was above the unstimulated cells). The apparent diffusion of the swimming drifting circle of unstimulated S. purpuratus spermatozoa is Dapp = 9 ± 3 µm2 s−1 (Friedrich, 2008; Friedrich and Jülicher, 2008; Riedel et al., 2005), here responsive cells were considered by showing a Dapp = 9 µm2 s−1, and were evaluated at second 9.
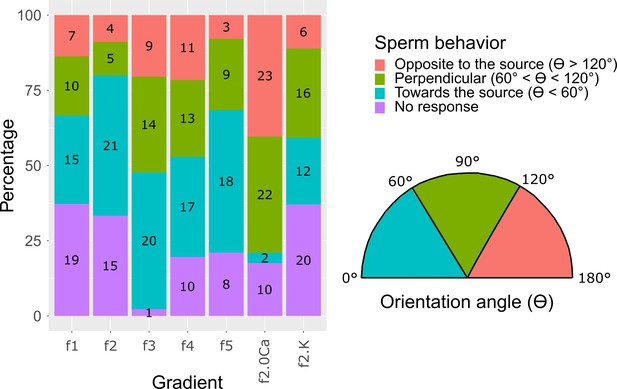
Sperm swimming behavior in response to different chemoattractant gradients.
S. purpuratus sperm behavior classified in four different classes: i) No response (purple), when spermatozoa keep swimming in concentric circles and do not move more than 15 µm of progressive swimming; ii) Toward the chemoattractant source (cyan), when spermatozoa respond drifting in swimming circles with an orientation angle (θ) smaller than 60° (θ < 60°); iii) Opposite to the chemoattractant source (pink), when spermatozoa respond drifting in swimming circles with an orientation angle (θ) bigger than 120° (θ > 120°); and iv) Perpendicular to the chemoattractant source (green), when spermatozoa respond drifting in swimming circles with an orientation angle (θ) smaller than 120° but bigger than 60° (60° ≤ θ ≤ 120°). The orientation angle θ is defined as the swimming angle of the drifting circles after speract uncaging (see Figure 4b). This analysis was made at second six after speract uncaging. Numbers in each bar represent the number of spermatozoa in each condition. Inset at the right panel shows the orientation angles (θ). For further information see Extended Materials and methods, section 2.7. Sperm swimming behavior in different chemoattractant gradients.
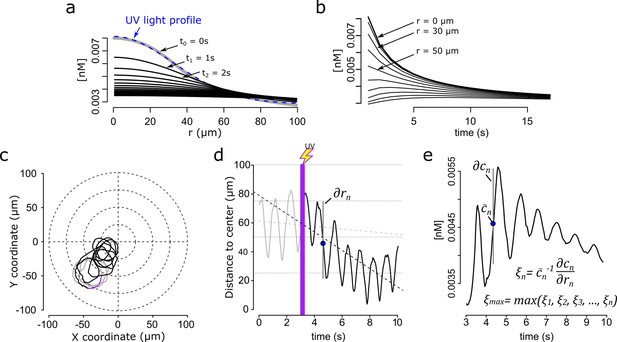
Steep speract gradients provoke chemotaxis in S. purpuratus spermatozoa. a.
Dynamics of the f2 speract gradient. The blue dashed line (t0 = 0 s) corresponds to a Gaussian distribution fitted to the UV light profile and illustrates the putative shape of the instantaneously-generated speract concentration gradient. Solid black lines illustrate the temporal evolution of the speract concentration field after t = 1, 2, 3, …, 20 s. (b) Temporal changes in the f2 speract field computed radially (each 10 µm) from the center of the gradient. (c) Characteristic motility changes of a S. purpuratus spermatozoon exposed to the f2 speract gradient. Solid lines illustrate its swimming trajectory 3 s before (gray), during UV flash (purple) and 6 s after (black) speract exposure. (d) Spermatozoa head distance to the source of the speract gradient versus time, calculated from sperm trajectory in (c). (e). Stimulus function computed from the swimming behavior of the spermatozoon in (c), considering the dynamics of (a and b).
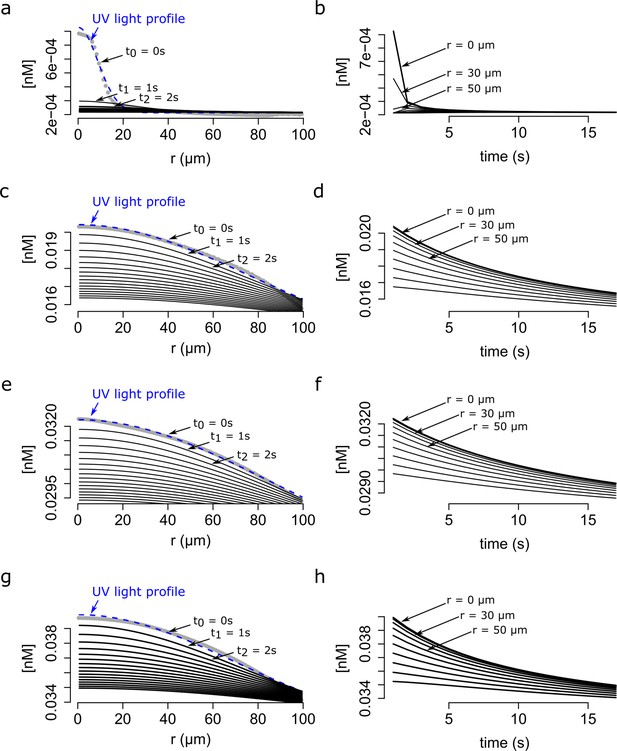
Modeling of the dynamics of speract gradient based on the UV light profile of distinct optical fibers.
The radial profiles of the UV light scattered at the glass-liquid interface of f1, f3, f4, f5 optical fibers are shown in gray. The speract gradient was generated as in Figure 5, but with the corresponding f1 (a and b), f3 (c and d), f4 (e and f) and f5 (g and h) optical fibers. Left panels - The dynamics of the speract gradient computed as is in Figure 5. The blue dashed line (t0 = 0) corresponds to a Gaussian distribution fitted to the UV light profile, and illustrates the putative shape of the instantaneously generated speract gradient. Solid black lines illustrate the shape of the speract gradient after t = 1, 2, 3, …, 20 s. Right panels - Simulated temporal changes in speract concentration gradients of f1 (a), f3 (c), f4 (e) and f5 (g) at each 10 µm radial point from the center of the concentration gradient.
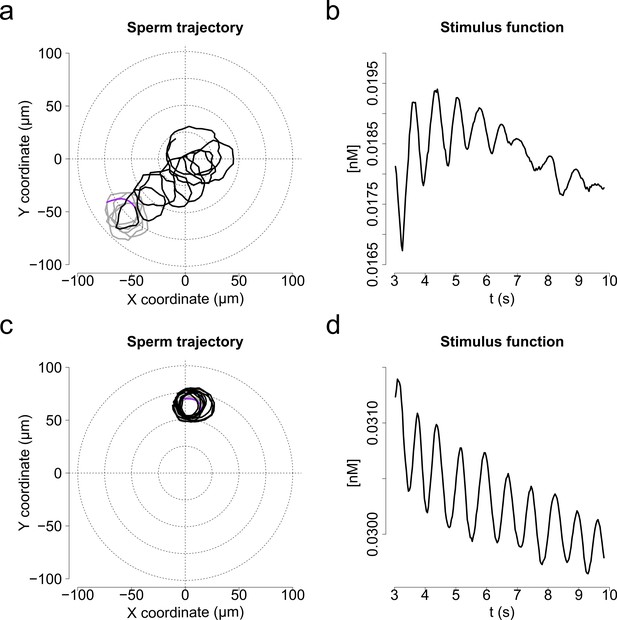
Characteristic motility changes of a S. purpuratus spermatozoon exposed to f3 and f4 speract gradients (chemotactic vs. non-chemotactic response).
Panels (a and b) show single cell responses to the f3 speract gradient (chemotactic); and panels c) and d) to the f4 speract gradient (non-chemotactic). (a, c) Solid lines illustrate the spermatozoon swimming trajectory 3 s before (gray) and 6 s after (black) speract gradient exposure. (b, d). Stimulus function computed from (a and c), considering the spatio-temporal dynamics of speract computed for the f3 and f4 gradients, respectively.
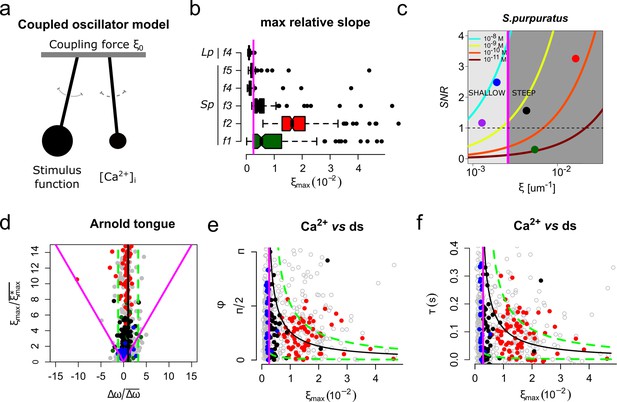
The slope of the speract concentration gradient generates a frequency-locking phenomenon between the stimulus function and the internal Ca2+ oscillator triggered by speract.
(a) Coupled oscillator model. Each sperm has two independent oscillators: i) stimulus function and ii) [Ca2+]i, which can be coupled through a forcing term that connects them, in our case the slope of the chemoattractant concentration gradient (ξ0). (b) Maximum relative slopes (ξmax) of the chemoattractant concentration gradient experienced by S. purpuratus (Sp) spermatozoa when exposed to f1, f2, f3, f4, f5 speract gradients. The maximum relative slopes of the chemoattractant concentration gradient experienced by L. pictus spermatozoa (Lp) toward f4 experimental regime are also shown. Note that ξmax for f2, f3, and f5, are up to 2–3 times greater than in f4, regardless of the species. (c) Experimental signal-to-noise ratios (SNR) regimes experienced by spermatozoa swimming in different gradient conditions. Note that only f2, f3 and f5 have higher SNR, compared to other gradient conditions, for which stochastic fluctuations mask the signal. This SNR calculation assumes a 10% of speract uncaging. The maximum relative slopes (ξ) are shown in log scale (d) Arnold’s tongue indicating the difference in intrinsic frequency of the internal Ca2+ oscillator of S. purpuratus spermatozoa, just before and after the speract gradient exposure. (e). Phase difference between the time derivative of the stimulus function and the internal Ca2+ oscillator of S. purpuratus spermatozoa, obtained by computing the cross-correlation function between both time series (Figure 6—figure supplement 2). (f). Phase difference between the time derivative of the stimulus function and the internal Ca2+ oscillator of S. purpuratus spermatozoa expressed in temporal delays. (d-f) Gray points represent the collated data of all f1, f2, f3, f4, f5 experimental regimes. Red, black and blue points indicate chemotactic spermatozoa (CI > 0 at second three after UV flash), located in R3, and R4 regions just before the speract gradient is established under f2, f3 and f5 experimental regimes, respectively. Magenta lines represent the transition boundary (γmin = ~ 2.6×10−3 µm−1, see also Figure 1d–f) below which no synchrony is observed, obtained from the theoretical estimates (black curves, mean of Δω) of panels (e) and (f). Green dashed lines indicate confidence intervals (mean ± standard deviation).
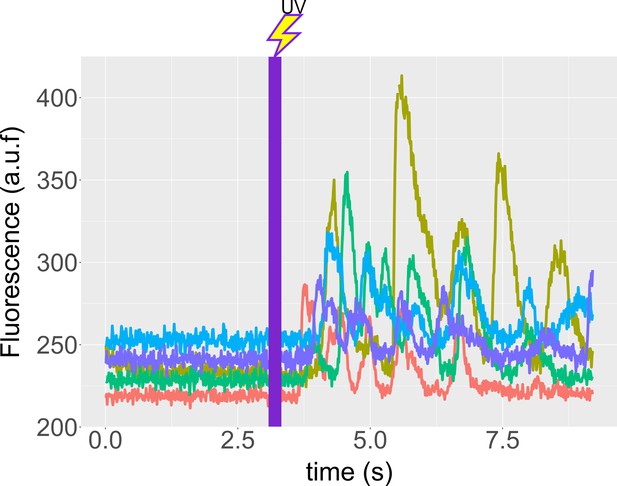
Speract induces Ca2+ oscillations in immobilized S. purpuratus spermatozoa.
Spermatozoa were immobilized on cover slips coated with poly-D-lysine (see Materials and methods), and ASW containing 500 nM caged speract added. Recordings were performed 3 s before and during 6 s after 200 ms of UV irradiation. f4 optical fiber was used for the UV light path, to generate the speract concentration gradient. Time traces indicate the [Ca2+]i of selected spermatozoa of Appendix 1—video 8. Note that the photo-release of speract induces a train of [Ca2+]i increases in immobilized spermatozoa, and hence provides evidence for the presence of an internal Ca2+ oscillator triggered by speract.
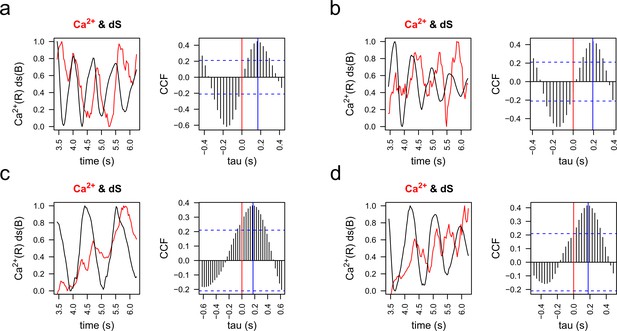
Cross-correlation analysis of [Ca2+]i and stimulus function derivative (dS) signals.
Representative examples of [Ca2+]i (red) and the derivative of the stimulus function (dS) (black) were plotted and then analyzed by cross-correlation analysis (CCF). Red vertical lines indicate the zero, blue vertical lines indicate the point of maximum correlation for each case, which means that the phase shifting between [Ca2+]i and dS is around 200 ms in these cases. Examples of a pair of spermatozoa for the two principal chemotactic gradients (f2 and f3) are shown. (a, b) Representative examples of two spermatozoa in an f2 gradient. (c, d) Representative examples of two spermatozoa in an f3 gradient.
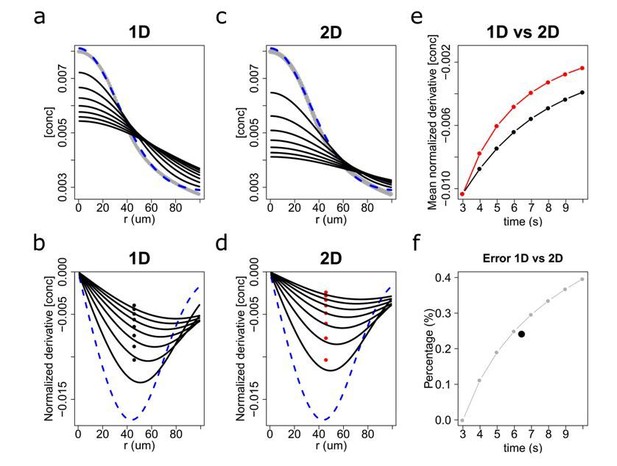
Diffusion solutions in 1 dimension (1D) and 2 dimensions (2D).
Diffusion simulation in 1D (a,b) and 2D (c,d) and the estimated relative error percentage between 1D and 2D (f). The numerical solution for the time dependent diffusion equation (i.e. the concentration profiles) for 1D and 2D, respectively, is shown in (a) and (c). The initial profile set at the time when the chemoattractant was activated with a UV flash (t = 3s) is highlighted as a blue dash line. Time evolution is shown in 1s intervals up to t = 10s (black lines). (b and d) depict the relative gradients (i.e. normalized derivatives) of the chemoattractant concentration. Black and red solid points indicate their spatial average at each time step, which in panel (e) is plotted as a function of time. In (f), the error percentage between 1D and 2D models was computed as (2D-1D)/1D from panel (e), and its temporal average is marked by a black solid point that indicates that the mean error between the 1D and 2D diffusion models for a typical chemotactic trajectory is about 25%.
Videos
Typical motility and Ca2+ responses of S. purpuratus spermatozoa toward an f2-generated speract concentration gradient.
Spermatozoa swimming in artificial sea water containing 10 nM caged speract, 3 s before and 5 s after 200 ms UV irradiation. An optical fiber of 0.6 mm internal diameter (f2) was used for the UV light path to generate the speract concentration gradient. Real time: 30.8 frames s−1, 40x/1.3NA oil-immersion objective. Note that spermatozoa located at R2, R3 and R4 regions prior to speract exposure swim up the speract concentration gradient, toward the center of the imaging field. The pseudo-color scale represents the relative fluorescence of fluo-4, a Ca2+ indicator, showing maximum (red) and minimum (blue) relative [Ca2+]i. Six S. purpuratus spermatozoa were manually tracked for visualization purposes. Scale bar of 50 µm.
Sperm trajectory analysis and stimulus function.
Single-cell analysis was performed for approximately 1000 sperm trajectories for the different speract gradients (f1-f5 and negative controls). The sperm trajectory shown here is representative of a chemotactic sperm. This analysis was implemented after speract uncaging at 3 s (from 3.2 to 10 s). Trajectory before, after and during the 200 ms UV flash is shown in gray, black and purple, respectively.
Sperm trajectory analysis and chemotactic index (CI).
Single-cell analysis was performed for approximately 1000 sperm trajectories from the different speract gradients (f1-f5 and negative controls). Angle ϕ is calculated just once and is always the same for each sperm trajectory. Angle θ is calculated per frame of the video for each sperm trajectory, resulting in the chemotactic index kinetics for each sperm trajectory (right panel). The sperm trajectory shown here represents a chemotactic sperm. This analysis was implemented from 4.5 s to 10 s. Speract uncaging was induced at 3 s. Trajectory before and after speract release is shown in gray and black dots, respectively.
Typical motility and Ca2+ responses of S. purpuratus spermatozoa toward an f3-generated speract concentration gradient.
An optical fiber of 2 mm internal diameter (f3) was used for the UV light path to generate the speract concentration gradient. Other imaging conditions were set up as for Video 1. Note that spermatozoa located at R2, R3 and R4 regions prior to speract exposure swim up the concentration field toward the center of the gradient (R1). The pseudo-color scale represents the relative fluorescence of fluo-4, a Ca2+ indicator, showing maximum (red) and minimum (blue) relative [Ca2+]i. Six S. purpuratus spermatozoa were manually tracked for visualization purposes. Scale bar of 50 µm.
Chemotaxis of S. purpuratus spermatozoa requires extracellular calcium.
Spermatozoa swimming in artificial sea water with nominal calcium containing 10 nM caged speract 3 s before and 5 s after exposure to 200 ms UV light. Nominal calcium disrupts the electrochemical gradient required for Ca2+ influx, hence blocking the triggering of the internal Ca2+ oscillation by speract. The f2 fiber (0.6 mm diameter) was used to uncage speract in this control. Other imaging conditions were set up as for Video 1. Note that spermatozoa re-located after speract uncaging but they failed to experience the Ca2+-driven motility alteration triggered by speract. As a consequence, they failed to experience chemotaxis (compare with Video 1). The pseudo-color scale represents the relative fluorescence of fluo-4, a Ca2+ indicator, showing maximum (red) and minimum (blue) relative [Ca2+]i. Six S. purpuratus spermatozoa were manually tracked for visualization purposes. Scale bar of 50 µm.
Disrupting the K+ electrochemical gradient blocks chemotaxis of S. purpuratus spermatozoa.
Cells were swimming in artificial sea water containing 40 mM of KCl, and 10 nM caged speract 3 s before and 5 s after exposure to 200 ms UV light. High K+ in the ASW blocks the membrane potential hyperpolarization required for opening Ca2+ channels, and hence prevents the triggering of the internal Ca2+ oscillator by speract exposure. The f2 fiber (0.6 mm diameter) was used to uncage speract in this control. Other imaging conditions were set up as for Video 1. Note that spermatozoa re-located after speract uncaging but they failed to experience the Ca2+-driven motility alteration triggered by speract, and thus they failed to experience chemotaxis (compare with Video 1). The pseudo-color scale represents the relative fluorescence of fluo-4, a Ca2+ indicator, showing maximum (red) and minimum (blue) relative [Ca2+]i. Six S. purpuratus spermatozoa were manually tracked for visualization purposes. Scale bar of 50 µm.
Typical motility and Ca2+ responses of S. purpuratus spermatozoa toward an f1-generated speract concentration gradient.
An optical fiber of 0.2 mm internal diameter (f1) was used for the UV light path to generate the speract concentration gradient. Other imaging conditions were set up as for Video 1. Note that some spermatozoa re-located after speract uncaging but they failed to experience chemotaxis (compare with Video 1). The pseudo-color scale represents the relative fluorescence of fluo-4, a Ca2+ indicator, showing maximum (red) and minimum (blue) relative [Ca2+]i. Six S. purpuratus spermatozoa were manually tracked for visualization purposes. Scale bar of 50 µm.
Typical motility and Ca2+ responses of S. purpuratus spermatozoa toward an f4-generated speract concentration gradient.
An optical fiber of 4 mm internal diameter (f4) was used for the UV light path to generate the speract concentration gradient. Other imaging conditions were set up as for Video 1. Note that spermatozoa re-located after speract uncaging but they failed to experience chemotaxis (compare with Video 1). The pseudo-color scale represents the relative fluorescence of fluo-4, a Ca2+ indicator, showing maximum (red) and minimum (blue) relative [Ca2+]i. Six S. purpuratus spermatozoa were manually tracked for visualization purposes. Scale bar of 50 µm.
Typical motility and Ca2+ responses of S. purpuratus spermatozoa toward an f5-generated speract concentration gradient.
An optical fiber of 4 mm internal diameter (f5) was used for the UV light path to generate the speract concentration gradient. Other imaging conditions were set up as for Video 1. Note that spermatozoa located at R2, R3 and R4 regions prior to speract exposure swim up the speract concentration gradient, toward the center of the imaging field (R1). The pseudo-color scale represents the relative fluorescence of fluo-4, a Ca2+ indicator, showing maximum (red) and minimum (blue) relative [Ca2+]i. Six S. purpuratus spermatozoa were manually tracked for visualization purposes. Scale bar of 50 µm.
Chemotactic index distributions.
Radial histograms of chemotactic indices from each different speract gradient. Black (f2) or red (rest) lines represent the median of each distribution. This analysis was implemented from 4.5 s to 10 s. Speract uncaging was induced at 3 s.
Photo-release of caged speract induces Ca2+ oscillations in immobilized S. purpuratus spermatozoa.
Spermatozoa were immobilized, by coating the cover slip with poly-D-lysine, in artificial sea water containing 500 nM caged speract, 3 s before and during 6 s after 200 ms of UV irradiation. The f4 optical fiber was used for the UV light path to generate the speract concentration gradient. The optical fiber was mounted in a ‘defocused’ configuration to minimize the generation of UV light heterogeneities. 93 frames s−1, 40x/1.3NA oil-immersion objective, 4×4 binning. The pseudo-color scale represents the relative fluorescence of fluo-4, a Ca2+ indicator, showing maximum (red) and minimum (blue) relative [Ca2+]i. The brightness and contrast scale was adjusted for better visualization of [Ca2+]i transients in the sperm flagella (as a consequence some heads look artificially oversaturated, however no fluorescence saturation was observed in the raw data).
Tables
Sperm accumulation responses triggered by different speract gradients.
Optical fiber | Sperm accumulation at the central regions of the imaging field | Sperm depleted of distal regions of the imaging field | [Ca2+]i rise (fold) |
---|---|---|---|
f1 | No | No | <2 |
f2 | R1 and R2 | R3 and R4 | >2 |
f3 | R1, R2 and R3 | R4 | >2 |
f4 | No | No | >2 |
f5 | No | No | ~2 |
-
Accumulation responses were evaluated at second 6, that is 3 s after photo-liberation of speract by a 200 ms UV flash.
Additional files
-
Supplementary file 1
Parameters of the chemoattractant sampling model for each species.
Note that the main differences between species are the number of receptors . number of receptors that allows half maximal binding rate for any concentration of chemoattractant, that is πa/s. D diffusion coefficient of the chemoattractant; association rate constant; effective radius of the chemoattractant (as proxy of chemoattractant receptor's binding site radius); sampling time (time to swim half the circumference in the boundary close to the water-glass interface); v mean linear speed of the spermatozoa, ; sampling distance (circumference diameter); L length of sperm flagellum; a spermatozoa radius, assuming that flagella are spheres; Pe Peclet number for a spherical cell approximation (sphere), or cylindrical flagellum geometry (cylinder). a Measured in this study (mean ± sd); N = 3 sea urchins; n = 495 (S. purpuratus), n = 56 (L. pictus) spermatozoa. bNishigaki and Darszon (2000); Nishigaki et al. (2001). c Calculated in this study (see section 1.1. On the estimate of maximal chemoattractant absorption). d Measured in this study (mean ± sd); N = 1 sea urchin; n = 26 (S. purpuratus), n = 39 (L. pictus) spermatozoa. eKashikar et al. (2012). fPichlo et al. (2014) reported 6.5 x 10−8 cm for the resact radius. g Calculated in this study.
- https://cdn.elifesciences.org/articles/50532/elife-50532-supp1-v2.docx
-
Supplementary file 2
Physical diameter of the optical fibers, and UV light power measured at the back focal plane of the objective.
*Typically, there is an extra 20% loss of light power between the back focal plane of the objective and the sample, due to scattering within the optics.
- https://cdn.elifesciences.org/articles/50532/elife-50532-supp2-v2.docx
-
Transparent reporting form
- https://cdn.elifesciences.org/articles/50532/elife-50532-transrepform-v2.docx