Dynamic post-translational modification profiling of Mycobacterium tuberculosis-infected primary macrophages
Figures
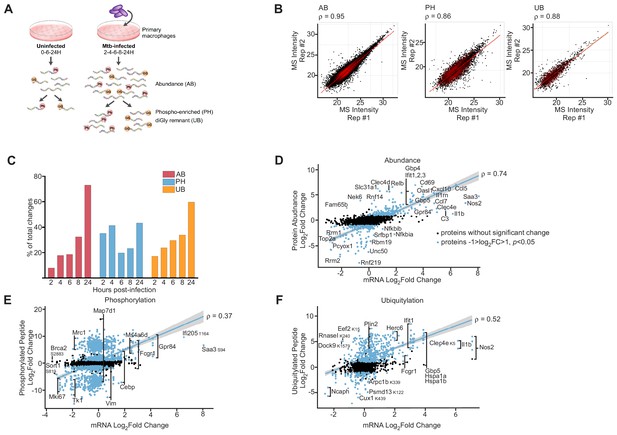
Proteomics and RNAseq profiling of Mtb infected bone-marrow-derived macrophages.
(A) Schematic for the experimental design indicating the hours post-infection at which point mock-infected or Mtb infected macrophages were harvested and peptides were generated for global protein abundance measurements by LC-MS. Phosphorylated peptides (Ph) or ubiquitylated peptides containing the diGly-remnant (GG) were separately enriched. (B) Replica plots of MS intensity measurements for individual peptides in global protein abundance (AB), phosphopeptide (PH), or ubiquitylated peptide (UB) samples. The correlation coefficient is displayed (ρ). (C) Percent of total changes (log2(fold change) greater than one or less than −1, p value less than 0.05) occurring at each time point 2–24 hr post-infection for global protein abundance, phosphorylation, or ubiquitylation. (D–F) Correlation plot of changes in gene transcription and protein abundance (AB; D), phosphorylation (PH; E), or ubiquitylation (UB; F) at 24 hr post-infection with Mtb. Proteins with no statistically significant changes during Mtb infection are colored black. Proteins with statistically significant changes during Mtb infection are colored blue. The correlation coefficient (ρ) for proteins with statistically significant changes during Mtb infection and mRNA levels are shown. Multiple phosphorylation sites were detected in Gpr84, Fcgr1, Ms4a6d, Vim, Map7d1, Mrc1, Tk1, and Mki67 (Supplementary file 5).
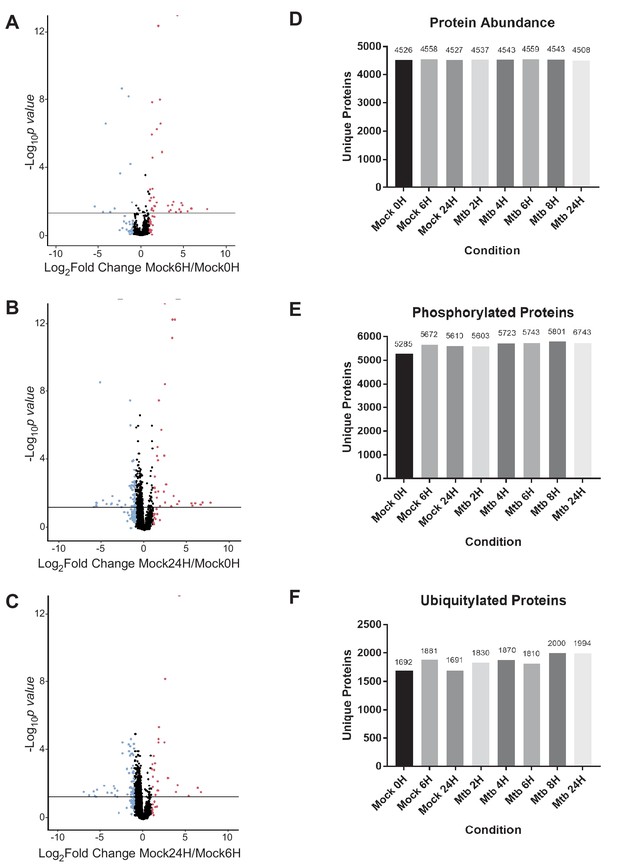
Host protein abundance changes in mock-infected macrophages, and unique host proteins identified at each time point 2–24 hr post-infection with Mtb or mock infection.
(A–C) Volcano plots displaying the proteins changing in abundance during mock infection between 0- and 6 hr (A), 0- and 24 hr (B), and 6- and 24 hr (C). Proteins with a p value less than 0.05 and log2(fold change) greater than one are colored red. Proteins with a p value less than 0.05 and log2(fold change) less than −1 are colored blue. (D–F) Graph of the number of unique proteins identified by mass spectrometry in biological triplicate, technical duplicate samples for each experimental condition from unenriched (D), phosphoenriched (E), or di-Gly enriched (F) peptide samples.
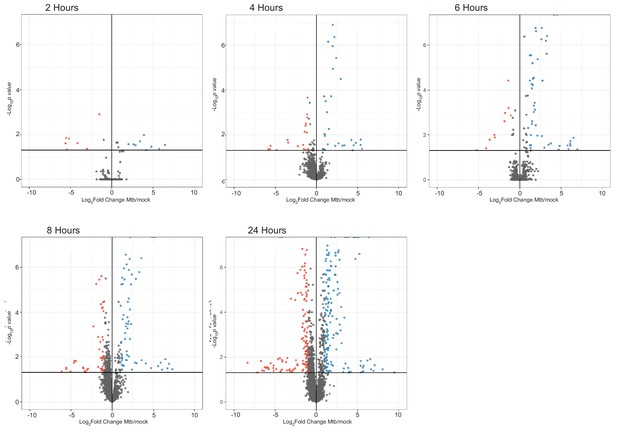
Host protein abundance changes during a time course of Mtb infection.
Volcano plots displaying the proteins changing in abundance during Mtb infection. Proteins with a p value less than 0.05 and log2(fold change) greater than one are colored red. Proteins with a p value less than 0.05 and log2(fold change) less than −1 are colored blue.
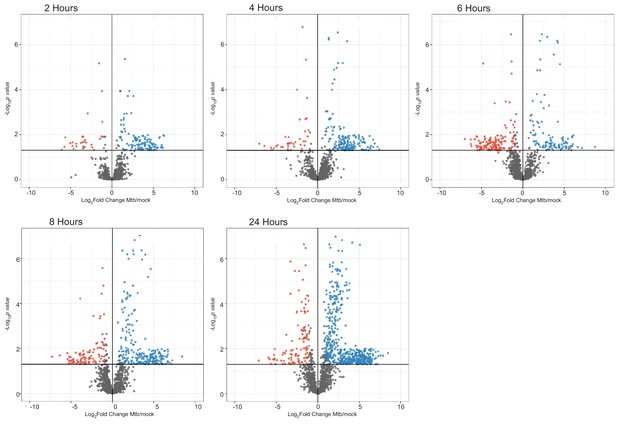
Changes in host protein ubiquitylation during a time course of Mtb infection.
Volcano plots displaying the changes in di-Gly modified peptides during Mtb infection. Ubiquitylated peptides with a p value less than 0.05 and log2(fold change) greater than one are colored red. Ubiquitylated peptides with a p value less than 0.05 and log2(fold change) less than −1 are colored blue.
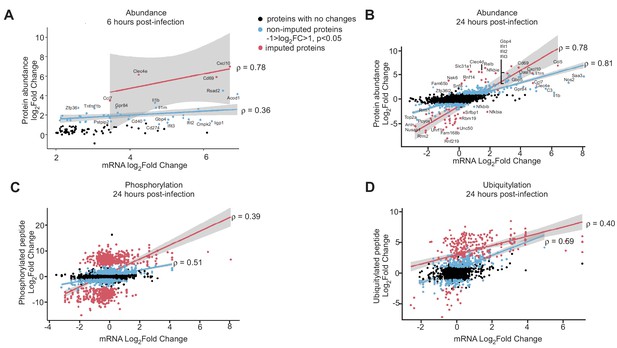
Correlation between host mRNA and protein abundance or PTMs changes during Mtb infection.
(A, B) Correlation plot of changes in mRNA and protein abundance 6- (A) and 24 hr (B) post-infection with Mtb. (C, D) Correlation plot of changes in mRNA and phosphorylation (C) or ubiquitylation (D) at 24 hr post-infection with Mtb. Proteins with no statistically significant changes during Mtb infection are colored black. Non-imputed proteins with statistically significant changes during Mtb infection are colored blue. Imputed proteins during Mtb infection are colored red. The correlation coefficient for non-imputed and imputed proteins are both shown.
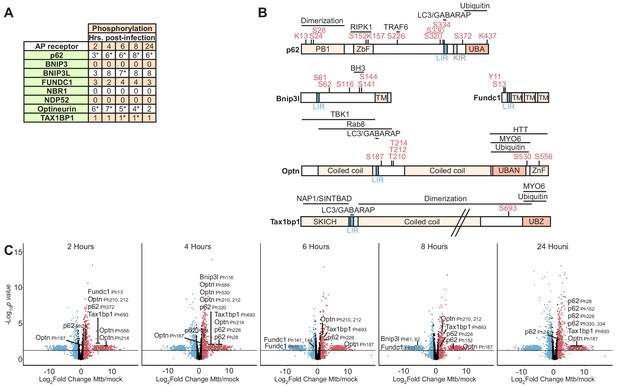
Autophagy receptor phosphorylation during Mtb infection.
(A) Table showing the number of unique phosphosites in each autophagy receptor at 2–24 hr post-infection. * p value less than 0.05 and log2(fold change) greater than one or less than −1 for at least one of the non-imputed phosphosites. (B) Domain organization displaying post-translational modifications in autophagy receptors. The LC3-interacting region (LIR), ubiquitin binding domain (UBAN, UBZ), myosin-6 binding domain (MYO6), SKIP carboxyl homology domain (SKICH), and TANK-binding kinase-1 (TBK1) binding domains are labeled. (C–G) Volcano plots highlighting changes in autophagy receptor phosphorylation at each time point 2–24 hr post-Mtb infection. Proteins with a log2(fold change) greater than one are colored red. Proteins with a log2(fold change) less than −1 are colored blue. Proteins with a p value less than 0.05 (or -log10 (p value) greater than 1.3) are above the horizontal black line. Phosphorylated residues and ubiquitylated lysine residues are noted. Statistical analysis of phosphopeptide data was performed with artMS version 1.4.0.
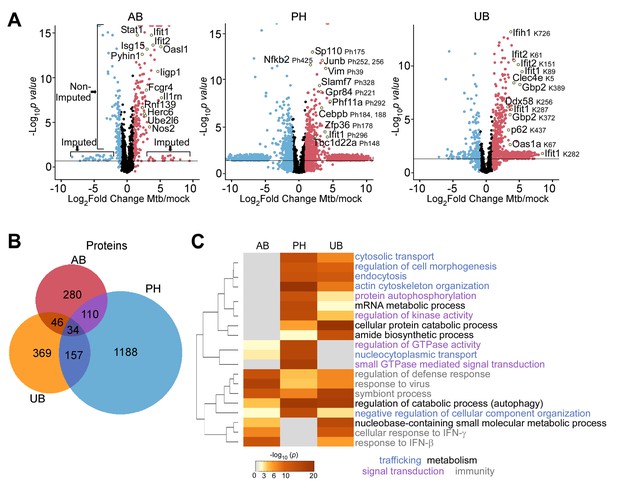
Comparison of macrophage proteins changing in abundance, phosphorylation, or ubiquitylation during Mtb infection.
(A) Volcano plots displaying proteins changing in abundance, phosphorylation, or ubiquitylation at 24 hr post-infection. Proteins with a log2(fold change) greater than one are colored red. Proteins with a log2(fold change) less than −1 are colored blue. Proteins with a p value less than 0.05 (or -log10(p value) greater than 1.3) are above the horizontal black line. Phosphorylated residues and ubiquitylated lysine residues are noted. (B) Venn diagram displaying the number of unique and overlapping proteins changing in abundance (AB), phosphorylation (PH), or ubiquitylation (UB) in the aggregate measurements 2–24 hr post-infection. (C) Enriched ontogeny clusters highlighting commonly enriched pathways. Pathways are colored coded into common groups (trafficking, metabolism, signal transduction, immunity). The dendrogram displays clustering of the gene ontogeny pathways.
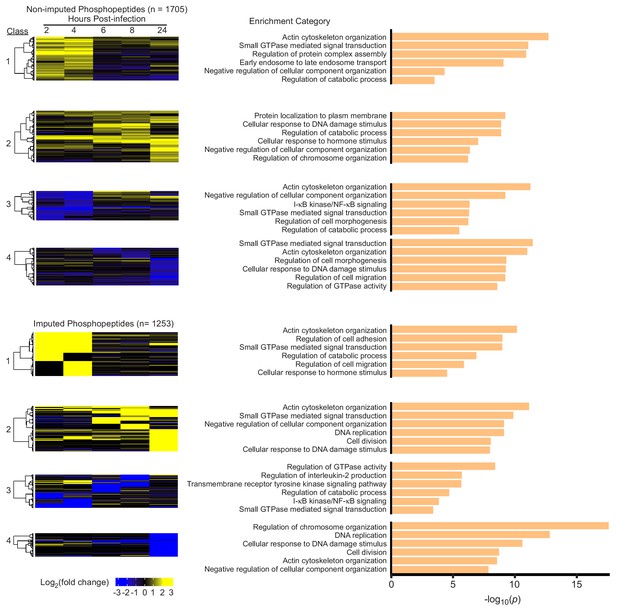
Changes in host protein phosphorylation during Mtb infection.
(A) Cluster analysis and heat map displaying the log2(fold change) in phosphorylated peptide levels for non-imputed and imputed proteins with a p value less than 0.05. The most statistically significant functional enrichment term and representative proteins are indicated for each class of proteins. (B) Heat map visualization of functional enriched terms for each protein class. Statistical analysis of phosphopeptide data was performed with artMS version 0.9.
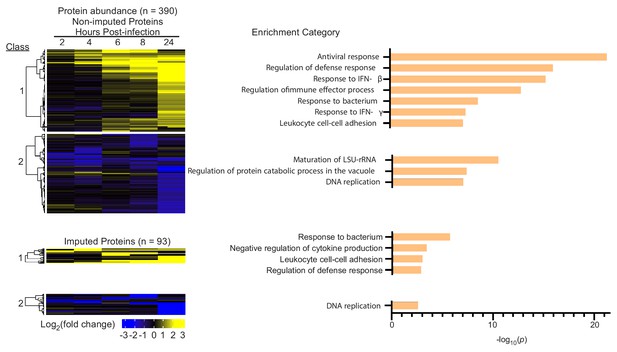
Host protein abundance changes during infection with Mtb.
(A) Cluster analysis and heat map displaying the log2(fold change) in protein abundance for non-imputed and imputed proteins with a p value less than 0.05. The most statistically significant functional enrichment term is indicated for each class of proteins. (B) Graph of the functional enriched terms for protein class 1 and 2.
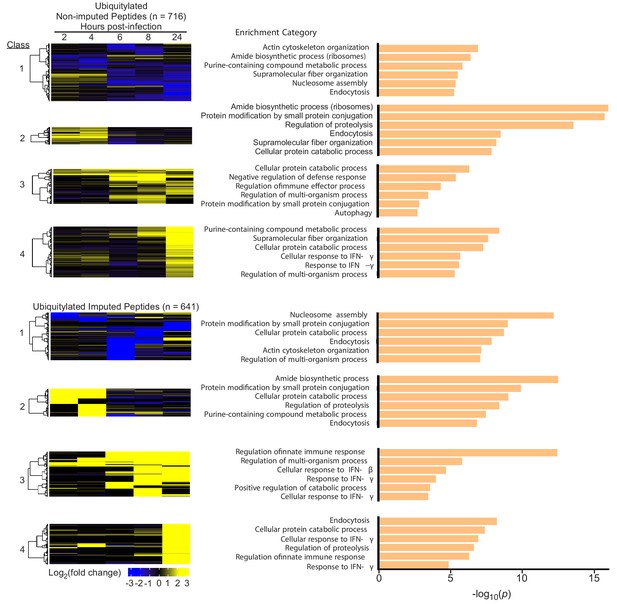
Changes in host protein ubiquitylation during Mtb infection.
(A) Cluster analysis and heat map displaying the log2(fold change) in ubiquitylated peptide levels for non-imputed and imputed proteins with a p value less than 0.05. The most statistically significant functional enrichment term and representative proteins are indicated for each class of proteins. (B) Heat map visualization of functional enriched terms for each protein class.
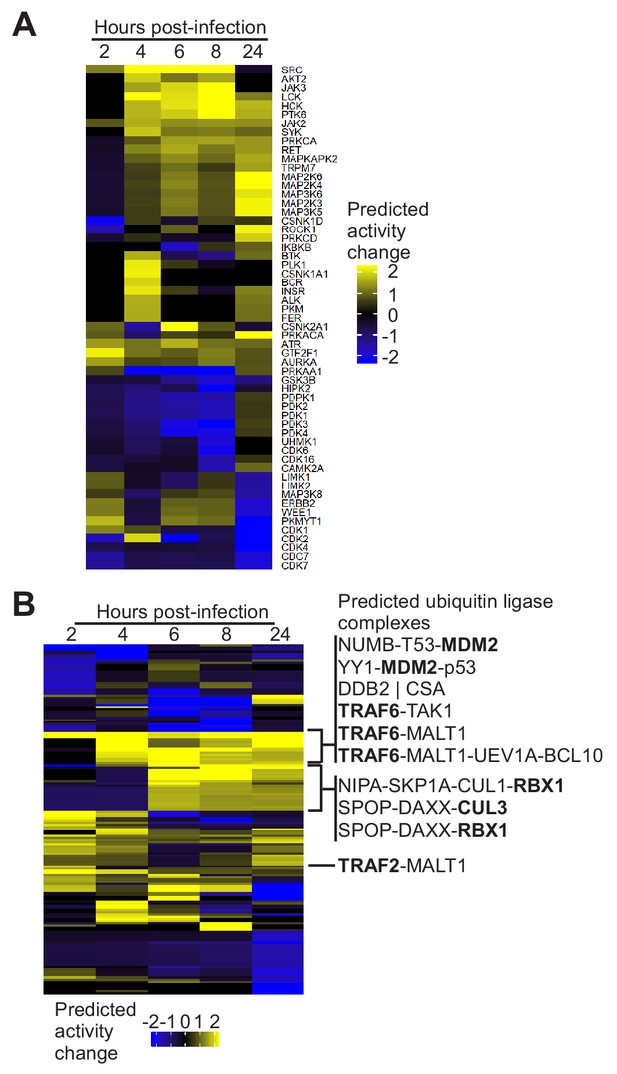
Protein kinase and effector complex activity predictions.
(A) Cluster analysis and heat map displaying the predicted host protein kinase activity. Mouse phosphopeptide sites (artMS version 0.9) were mapped to the human proteome and kinase activity predictions were performed with PhosFate (Ochoa et al., 2016). (B). Effector complex activity predictions. Effector complexes containing ubiquitin ligases (bold) are listed.
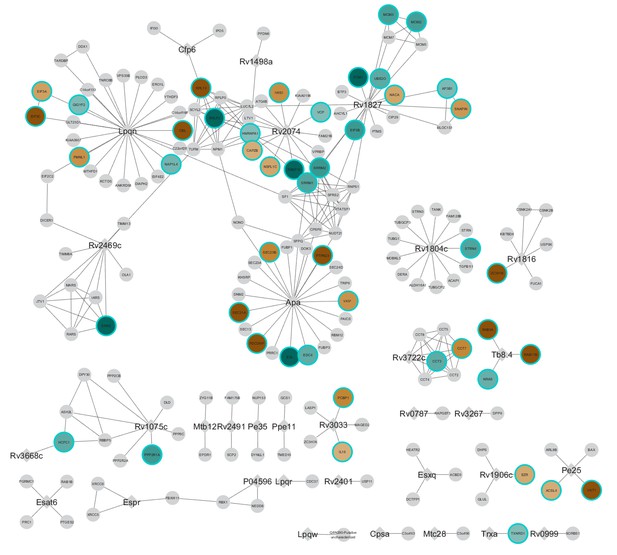
Overlap of PTMs and host proteins that interact with Mtb.
Mtb proteins (diamonds) and host protein (circle) interactions (Penn et al., 2018) are connected by dotted lines. Phosphorylated or ubiquitylated sites from primary macrophage proteins that increased during infection are brown, and those that decreased during infection are green. PTMs were aggregated from all of the time points measured and statistically significant non-imputed changes ((log2(fold change)>1 or <-1, p value less than 0.05) and imputed changes are displayed. The post-translationally modified residue is noted after the Unitprot ID (UnitprotID_PTM). The figure is viewable and searchable on the on-line NDEx server: http://ndexbio.org/#/network/2f38c264-1e05-11ea-bb65-0ac135e8bacf.
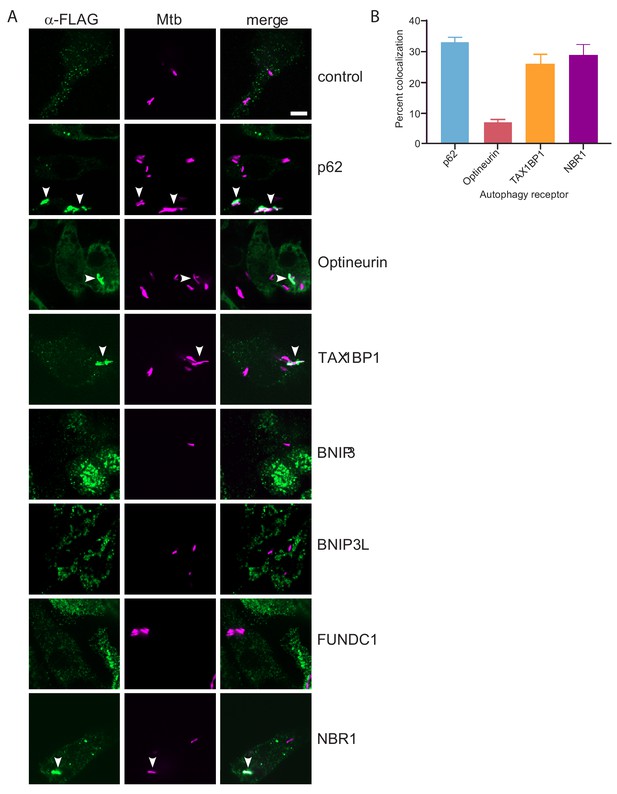
TAX1BP1, p62, optineurin, and NBR1 colocalize with Mtb.
(A) Fluorescence images of RAW macrophages expressing FLAG-tagged autophagy receptors (green) with mCherry-expressing Mtb (magenta). White bar denotes 10 µm. (B) Quantitative analysis of Mtb colocalization with FLAG-tagged autophagy receptors. Results are the ± SEM for three technical replicates. Colocalization of mCherry-expressing Mtb with the FLAG-tagged autophagy receptors was 33 ± 1.7% for p62, 29% ± 3.3% for NBR1, 7% ± 1% for Optineurin, and 26 ± 3.2% for TAXBP1. There were not significant amounts of colocalization with Mtb in macrophages expressing FLAG-tagged BNIP3, BNIP3L, or FUNDC1.
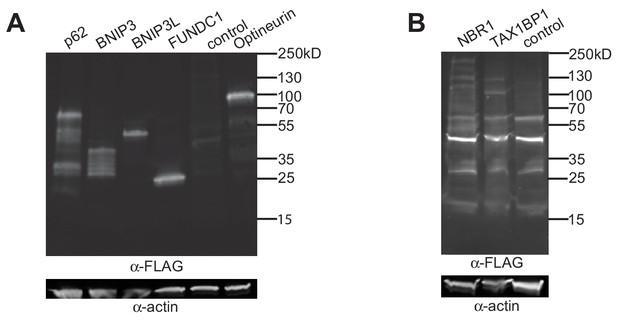
Epitope tagged autophagy receptors were expressed in RAW macrophages.
(A and B) Immunoblot showing expression of FLAG-tagged autophagy receptors in RAW macrophages. RAW macrophages were transduced with FLAG-tagged autophagy receptor constructs and cell lysates probed with α-FLAG and α-actin antisera. Immunoblots were imaged with a short (A) or a long (B) exposure time to better detect the higher molecular weight autophagy receptors (Tumbarello et al., 2015; Ito et al., 2015).
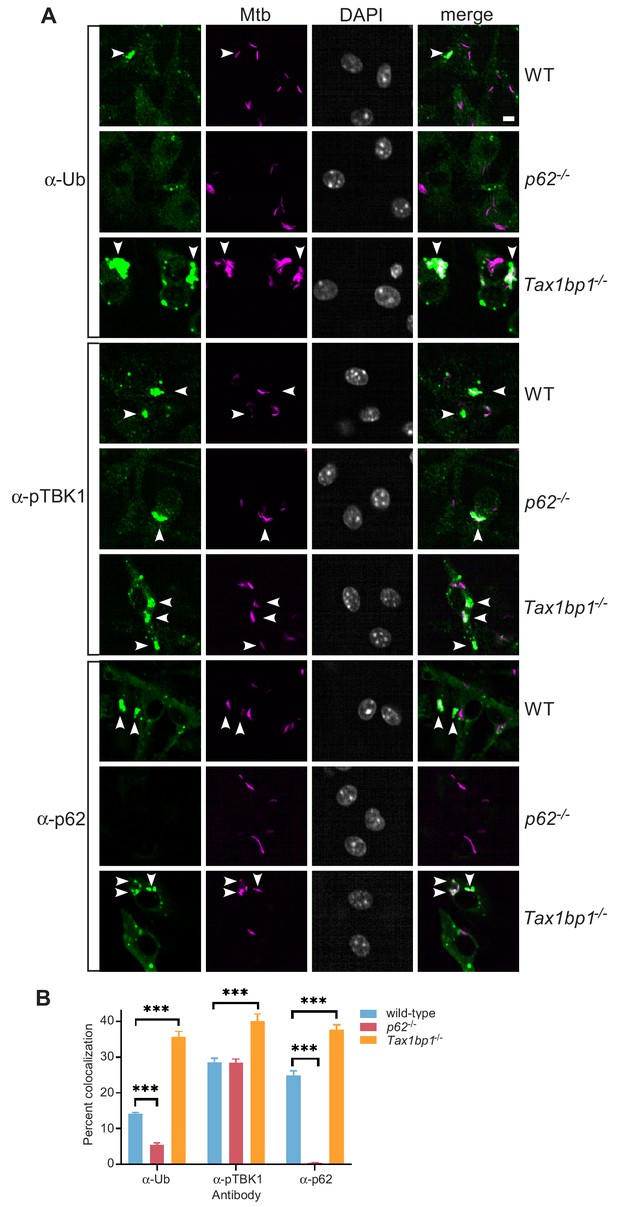
Accumulation of Mtb targeted to ubiquitin (FK2), p62, and phospho-TBK1 in TAX1BP1 targeted knockout macrophages.
(A) Fluorescence images of wild-type, p62-/-, or Tax1bp1-/- bone marrow-derived macrophages infected with mCherry-expressing Mtb (magenta) and immunostained with antibodies to ubiquitin (FK2), p62, or phospho-TBK1 (green). Nuclei were stained with DAPI (white). White bar denotes 10 µm. (B) Quantitative analysis of Mtb colocalization with ubiquitin, p62, and phospho-TBK1 at 8 hr post-infection. Results are the means ± SEM from at least four technical replicates and a single experiment representative of three independent experiments. *** p value less than 0.001 by t-test.
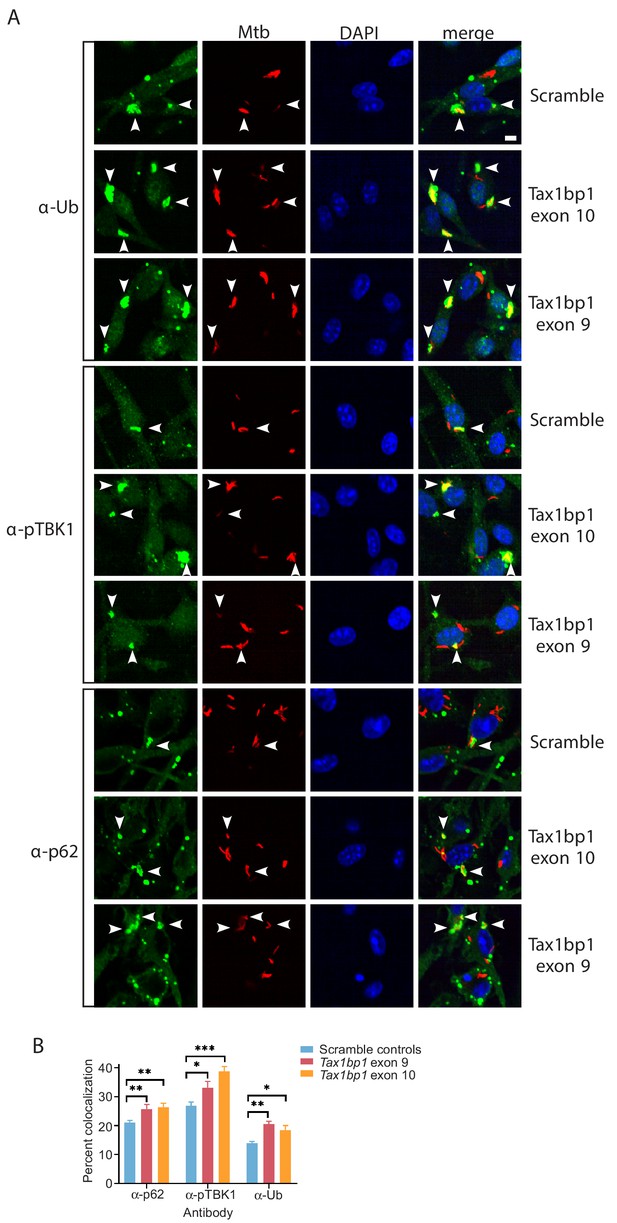
Accumulation of Mtb targeted to ubiquitin (FK2), p62, and phospho-TBK1 in TAX1BP1 edited macrophages.
(A) Fluorescence images of bone-marrow-derived macrophages transduced with scramble control guide RNAs or Tax1bp1 exon 10 or nine guide RNAs infected with mCherry-expressing Mtb (red) and immunostained with antibodies to ubiquitin (FK2), p62, or phospho-TBK1 (green). Nuclei were stained with DAPI (blue). White bar denotes 10 µm. (B) Quantitative analysis of Mtb colocalization with ubiquitin, p62, and phospho-TBK1 at 8 hr post-infection. Results are the means ± SEM of four technical replicates. * p value less than 0.05 by t-test. ** p value less than 0.01. *** p value less than 0.001.
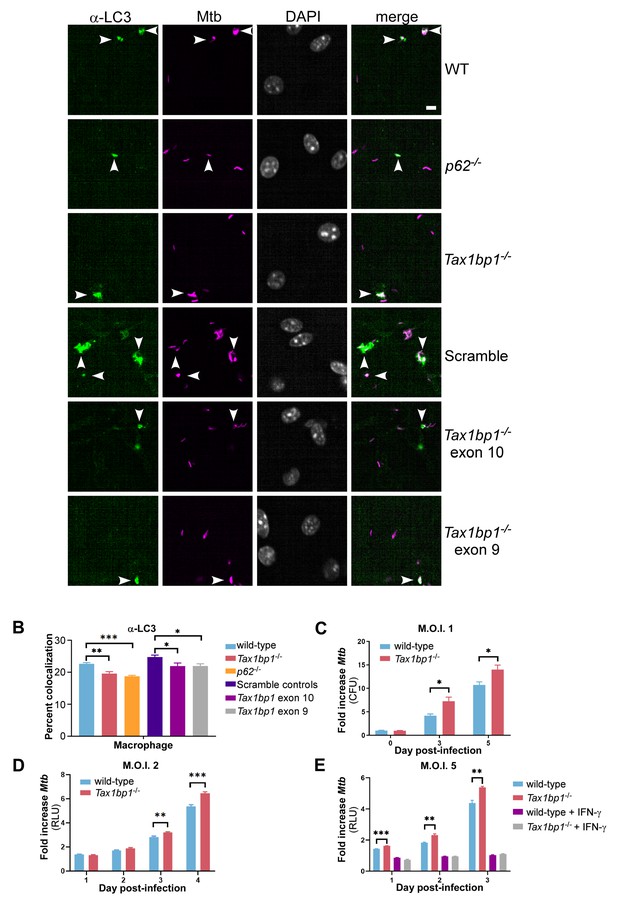
TAX1BP1 deficiency abrogates targeting of Mtb to LC3 and enhances the growth of Mtb in macrophages ex vivo.
(A) Fluorescence images of bone-marrow-derived macrophages infected with mCherry-expressing Mtb (magenta) and immunostained with α-LC3 antisera (green). Nuclei were stained with DAPI (white). White bar denotes 10 µm. (B) Quantitative analysis of Mtb colocalization with LC3 at 8 hr post-infection. Results are the means ± SEM for four technical replicates of a single experiment representative of two independent experiments. * p value less than 0.05 by t-test. ** p value less than 0.01. *** p value less than 0.001. (C) Bone-marrow-derived macrophages infected with Mtb at a multiplicity of infection of 1 were enumerated for CFU at days 0, 3, and 5 post-infection. Fold change in CFUs is displayed. Results are the means ± SEM of three technical replicates. (D and E) Luminescence from macrophages infected with Mtb harboring luxBCADE at a multiplicity of infection of 2 (D) or 5 (E) was measured daily. Fold change in relative light units (RLUs) is displayed. * p value less than 0.05 by t-test. ** p value less than 0.01. *** p value less than 0.001. Results are the means ± SEM of five technical replicates of two independent experiments.
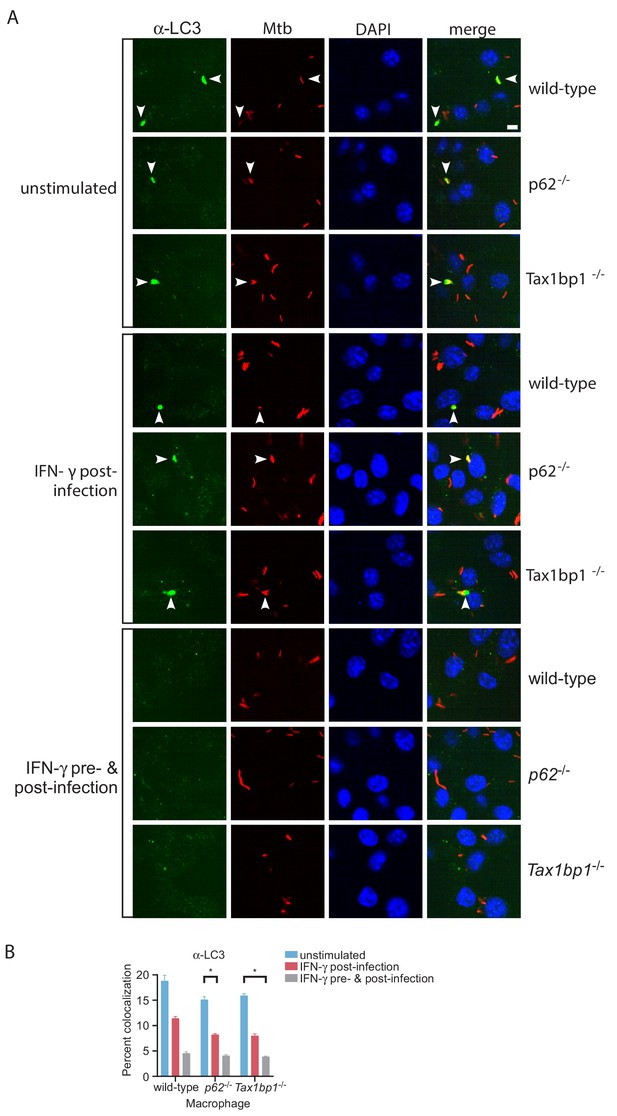
IFN-γ stimulation abrogates targeting of Mtb to LC3.
(A) Fluorescence images of bone-marrow-derived macrophages infected with mCherry-expressing Mtb (red) and immunostained with antibodies to LC3 (green). Nuclei were stained with DAPI (blue). White bar denotes 10 µm. (B) Quantitative analysis of Mtb colocalization with LC3 at 8 hr post-infection. Results are the means ± SEM for six technical replicates of a single experiment representative of three independent experiments. * p value less than 0.05 by t-test when compared to the respective wild-type control.
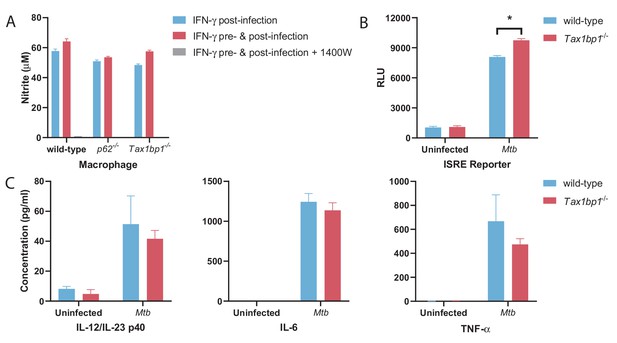
Nitrite and cytokine measurements from supernatants of macrophages infected with Mtb.
(A) Infected macrophages were stimulated with IFN-γ in the presence or absence of nitric oxide synthase inhibitor (1400W), and nitrite was measured from the supernatants. Results are the means ± SEM for six technical replicates. (B) ISRE reporter activity was measured from the supernatants of uninfected or Mtb-infection macrophages. Results are the means ± SEM for two independent biological replicates. * p value less than 0.05 by t-test. (C) IL-12, IL-6, and TNF-α levels were measured from the supernatants of uninfected or Mtb-infected macrophages. Results are the means ± SEM for two independent biological replicates.
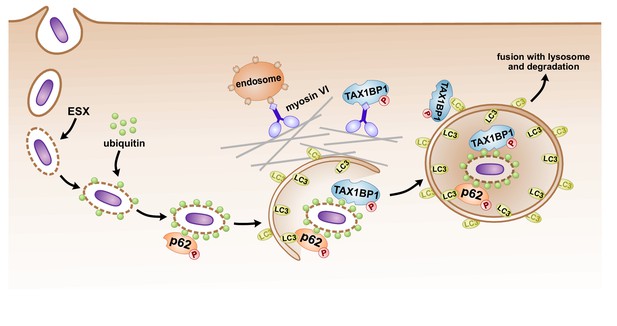
Model of autophagosomal targeting Mtb by sequential recruitment of autophagy receptors and their binding proteins.
Initial recruitment of p62 to the nascent autophagosome leads to amplification of ubiquitin recruitment to Mtb. Subsequently in a step downstream of p62 recruitment, TAX1BP1 mediates targeting of ubiquitylated Mtb to the LC3-positive phagophore.
Tables
Reagent type (species) or resource | Designation | Source or reference | Identifiers | Additional information |
---|---|---|---|---|
Cell line (M. musculus) | RAW 264.7 | ATCC | TIB-71 | |
Transfected construct | pENTR-N-FLAG | Parry et al., in preparation | Lentiviral construct to transfect and express FLAG-tagged autophagy receptors | |
Transfected construct | plentiGuide puro | Addgene | 52963 | Lentiviral construction to transfect and expressed guide RNAs |
Biological sample (M. musculus) | Primary bone marrow-derived macrophages, WT C57BL/6J | Jackson Laboratory | Stock # 000664 | Freshly isolated from mice |
Biological sample (M. musculus) | Primary bone marrow-dervied macrophages Rosa26-Cas9 knockin mouse | Jackson Laboratory (Platt et al., 2014) | Stock # 026179 | Freshly isolated from mice |
Antibody | anti-beta-actin (Mouse monoclonal) | Santa Cruz | 47778 | WB (1:1000) |
Antibody | Anti-FLAG M2 (Mouse monoclonal) | Sigma | F3165 | WB (1:1000) IF (1:500) |
Antibody | anti-p62 (Mouse monoclonal) | Abcam | 109012 | 1 μg/ml |
Antibody | Anti-phospho-Ser172-TBK1 (D52C2) | Cell signalling | 5483 | IF (1:400) |
Antibody | Anti-Ubiquitin (FK2) | Millipore Sigma | 04–263 | IF (1:400) |
Antibody | Anti-LC3 (clone 2G6) | Nanotools | 0260–100/LC3-2G6 | IF (1:200) |
Sequence-based reagent | PCR primers | IDT | PCR primers | Please see Supplementary file 2 |
Commercial assay | Luciferase assay system | Promega | E1500 | |
Commercial kit | Mouse IL-6 DuoSet ELISA | R and D | DY406 | |
Commercial kit | Mouse TNF-α DuoSet ELISA | R and D | DY410 | |
Commercial kit | Mouse IL-12/IL-23 p40 allele-specific DuoSet ELISA | R and D | DY499 | |
Chemical compound, drug | Recombinant murine IFN-g | Peprotech | 315–05 | |
Chemical compound, enzyme | Sequencing grade modified trypsin | Promega | V511 | |
Software, algorithm | MaxQuant | (Cox and Mann, 2008) | MS Database search software | |
Software, algorithm | artMS | (Jimenez-Morales et al., 2019) | MS Statistical analysis software | |
Other | DAPI stain | Invitrogen | D1306 | (1 μg/mL) |
Strain | Mtb: Erdman::pmv261Zeo_MCherry | (Penn et al., 2018) | mCherry-expressing Mtb Erdman strain | |
Strain | Mtb: Erdman (expressing luxCDABE operon) | (Roberts et al., 2019) | luciferase-expressing Mtb Erdman strain | |
Strain | Mtb: Erdman | ATCC | 35801 |
Additional files
-
Supplementary file 1
Rho values for peptide intensity levels from biological and technical replicates.
- https://cdn.elifesciences.org/articles/51461/elife-51461-supp1-v2.xlsx
-
Supplementary file 2
Primers used in this study.
- https://cdn.elifesciences.org/articles/51461/elife-51461-supp2-v2.xlsx
-
Supplementary file 3
Autophagy receptors changing in protein abundance, phosphorylation, or ubiquitylation during Mtb infection.
- https://cdn.elifesciences.org/articles/51461/elife-51461-supp3-v2.xlsx
-
Supplementary file 4
Protein abundance changes during Mtb infection.
- https://cdn.elifesciences.org/articles/51461/elife-51461-supp4-v2.xlsx
-
Supplementary file 5
Changes in phosphorylation during Mtb infection.
- https://cdn.elifesciences.org/articles/51461/elife-51461-supp5-v2.xlsx
-
Supplementary file 6
Changes in ubiquitylation during Mtb infection.
- https://cdn.elifesciences.org/articles/51461/elife-51461-supp6-v2.xlsx
-
Supplementary file 7
p values for Figure 5, Figure 5—figure supplement 1, Figure 6, Figure 6—figure supplement 1, Figure 6—figure supplement 2.
- https://cdn.elifesciences.org/articles/51461/elife-51461-supp7-v2.xlsx
-
Transparent reporting form
- https://cdn.elifesciences.org/articles/51461/elife-51461-transrepform-v2.docx