Non-enzymatic primer extension with strand displacement
Figures
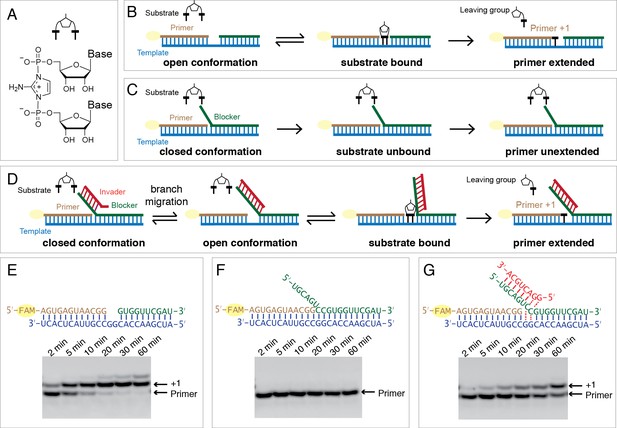
Single step non-enzymatic primer extension with strand displacement.
(A) Chemical structure of the 5ʹ−5ʹ-phosphorimidazolium-bridged dinucleotide. (B)-(D) Experimental design illustrations in three different cases. (E) PAGE analysis of a primer extension reaction corresponding to (B), the imidazolium-bridged dinucleotide C*C binds the open GG templating region and reacts with the 3ʹ-end of the primer, resulting in +1 extension product. (F) PAGE analysis of a primer extension reaction corresponding to (C). The blocker (green), an oligonucleotide complementary to the templating region, inhibits the primer extension reaction. (G) PAGE analysis of a primer extension reaction corresponding to (D). An invader (red), a short oligonucleotide partially complementary to the blocker, is able to rescue the primer extension reaction by sequestering the blocker and allowing C*C to bind and react. All primer extension reactions were conducted at room temperature, 50 mM Na+-HEPES, pH 8.0, 50 mM MgCl2, 3 mM C*C, 1.5 μM primer, 2.5 μM template, 0 or 3.5 μM blocker, 0 or 5 μM invader.
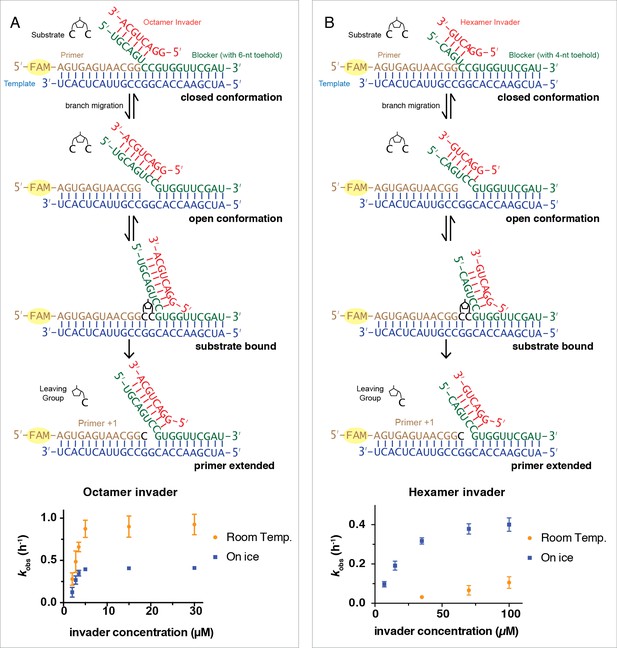
Rates of primer extension reactions as a function of invader concentration.
(A) Reactions with the blocker possessing a 6-nt toehold, and an 8-nt long invader. (B) Reactions with the blocker possessing a 4-nt toehold, and a 6-nt long invader. All primer extension reactions were conducted in 50 mM Na+-HEPES, pH 8.0, 50 mM MgCl2, 3 mM C*C, 1.5 μM primer, 2.5 μM template, 3.5 μM blocker, at room temperature or on ice as indicated. Reaction products were analyzed by denaturing PAGE. (n ≥ 3, The error bars are smaller than the symbol when they are not visible.).
-
Figure 2—source data 1
Numerical data represented in Figure 2.
- https://cdn.elifesciences.org/articles/51888/elife-51888-fig2-data1-v2.docx

Representative denaturing PAGE data and plots of ln(P/P0) vs. time for the reaction kinetics with an octamer invader, as described in Figure 2.

Representative denaturing PAGE data and plots of ln(P/P0) vs. time for the reaction kinetics with a hexamer invader as described in Figure 2.
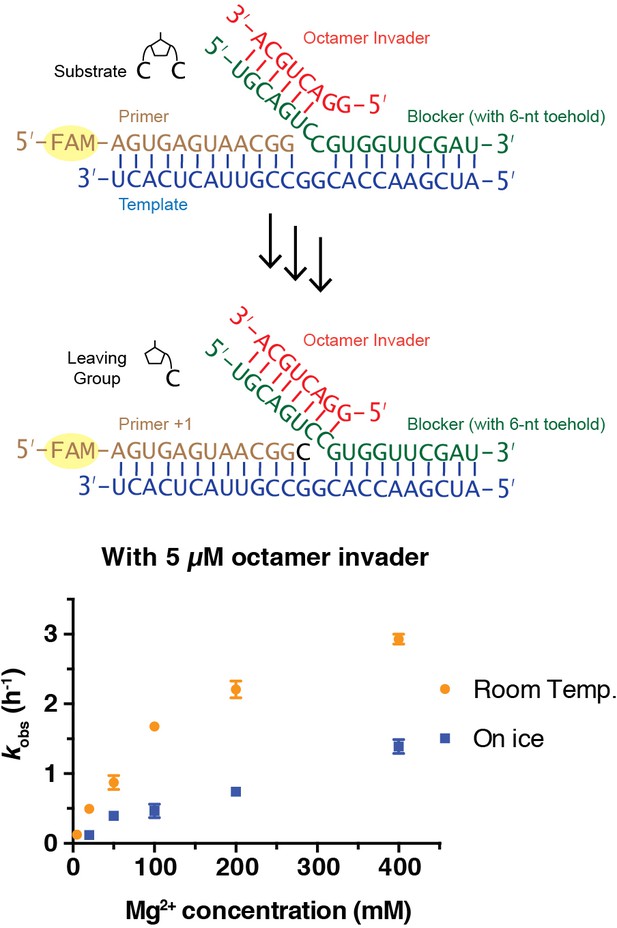
Rates of primer extension reactions as a function of Mg2+ concentration.
All primer extension reactions were conducted in 50 mM Na+-HEPES, pH 8.0, 3 mM C*C, 1.5 μM primer, 2.5 μM template, 3.5 μM blocker and 5 μM octamer invader, at room temperature or on ice as indicated. (n ≥ 3).
-
Figure 3—source data 1
Numerical data represented in Figure 3.
- https://cdn.elifesciences.org/articles/51888/elife-51888-fig3-data1-v2.docx

Representative denaturing PAGE data and plots of ln(P/P0) vs. time for the reaction kinetics with an octamer invader, as described in Figure 3.
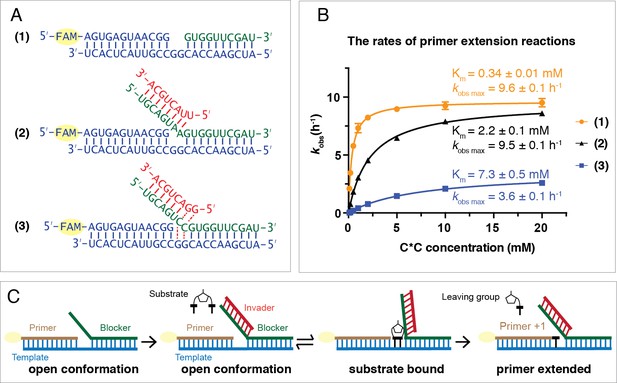
C*C dependent primer extension reactions in three different template regimes.
(A) Three different template configurations. In case (1), the template region GG is open. In case (2), the blocker oligonucleotide cannot base-pair with the template region GG. The complex is constitutively open. In case (3), the template region GG is partitioned between open and closed states. (B) Rates of primer extension reactions as a function of C*C concentration. (C) An experimental design illustration for case (2). Case (1) and (3) schematics have been shown in Figure 1B and D, respectively. All primer extension reactions were conducted at room temperature, 50 mM Na+-HEPES, pH 8.0, 50 mM MgCl2, 1.5 μM primer, 2.5 μM template, 3.5 μM blocker, 0 or 5 μM invader as indicated. Reaction products were analyzed by urea PAGE. (n = 4).
-
Figure 4—source data 1
Numerical data represented in Figure 4.
- https://cdn.elifesciences.org/articles/51888/elife-51888-fig4-data1-v2.docx

Representative denaturing PAGE data and plots of ln(P/P0) vs. time for the reaction kinetics in case (1) as described in Figure 4.

Representative denaturing PAGE data and plots of ln(P/P0) vs. time for the reaction kinetics in case (2) as described in Figure 4.

Representative denaturing PAGE data and plots of ln(P/P0) vs. time for the reaction kinetics in case (3) as described in Figure 4.
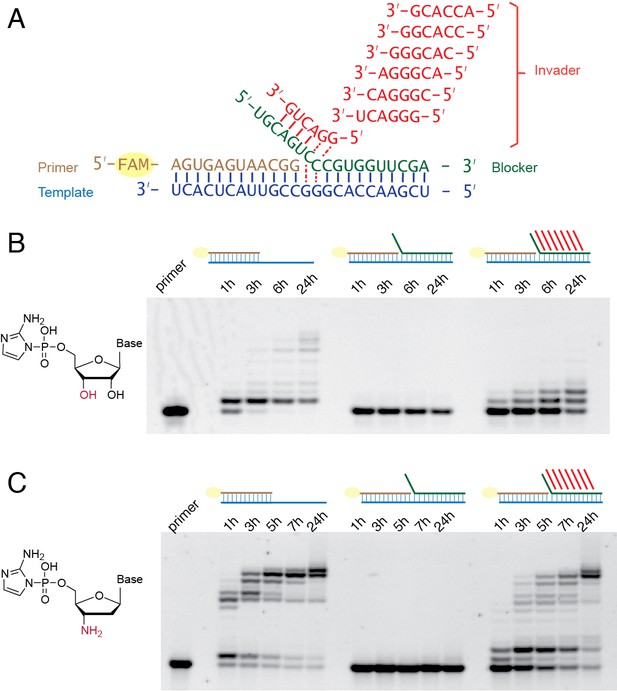
Multi-step primer extension with strand displacement.
(A) Illustration of the experimental configuration with seven different hexamer invaders. (B) PAGE analysis of primer extension with RNA monomers. The reactions were conducted at room temperature, 50 mM Na+-HEPES, pH 8.0, 400 mM MgCl2, 1.5 μM primer, 2.5 μM template, with or without 3.5 μM blocker as indicated, with or without 20 μM each hexamer invader as indicated, 5 mM C*C, 10 mM 2-AIpG and 10 mM 2-AIp2sU. (C) PAGE analysis of primer extension with 3′-amino-2′,3′-dideoxyribonucleotide monomers. Reactions were conducted as in (B), except that 10 mM 3′-NH2-2AIpddA, 10 mM 3′-NH2-2AIpddG and 10 mM 3′-NH2-2AIpddT were used instead of RNA monomers, and the 3′-amine primer was used instead of the RNA primer.
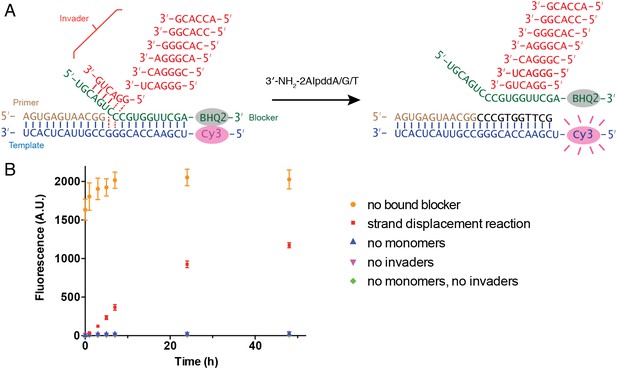
Fluorescence-quencher assay for non-enzymatic primer extension with strand displacement.
(A) Illustration of the experimental design. The template was labeled with Cy3 at its 5′-end. The blocker was modified by Black Hole Quencher−2 at the 3′-end. When the blocker forms a stable duplex with the template, the fluorescent signal of Cy3 is quenched. In the presence of invaders and 3′-NH2-2AIpddN, the primer extends, resulting in blocker dissociation and increasing Cy3 signal. (B) Time course of the fluorescent signal in five different experiment groups. Strand displacement reactions contained 1.5 μM template, 2 μM blocker, 2.5 μM primer, 50 mM Na+-HEPES, pH 8.0, 50 mM MgCl2, 20 μM each hexamer invader and 10 mM 3′-NH2-2AIpddA/G/T. Positive control reactions contained the same components, with the addition of 2 μM of complementary strand RNA to the template, so that the blocker was unbound. The negative control reactions contained the same components but omitted monomers and/or invaders, as indicated. The experiments were performed at room temperature in triplicate.
-
Figure 6—source data 1
Numerical data represented in Figure 6.
- https://cdn.elifesciences.org/articles/51888/elife-51888-fig6-data1-v2.docx