Auditory cortex shapes sound responses in the inferior colliculus
Figures
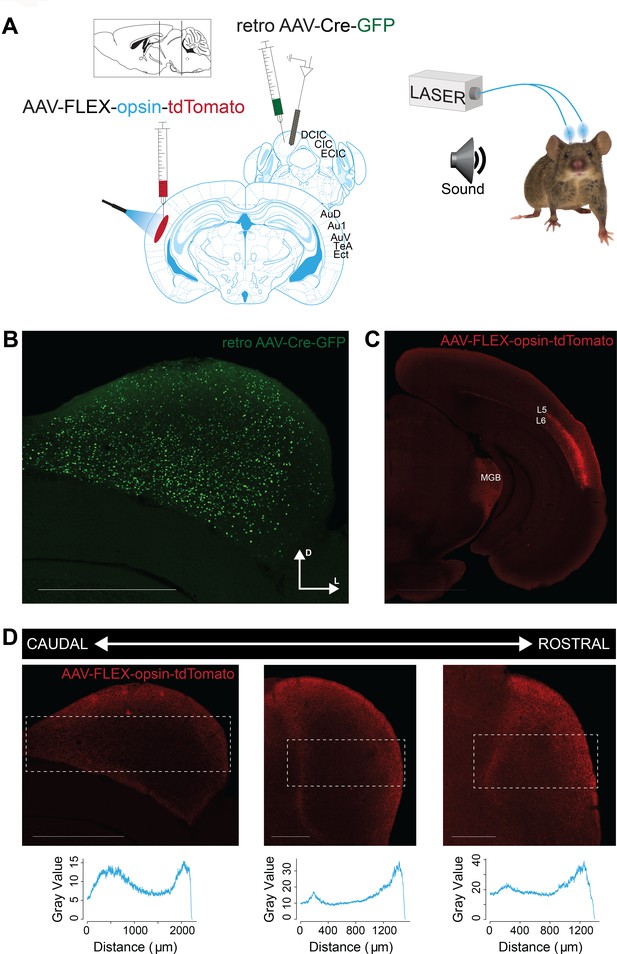
Opsin expression in corticocollicular feedback.
(A) Experimental design: To yield selective labeling of auditory cortico-collicular projections, a retroAAV-Cre-GFP construct is injected into the IC and AAV-FLEX-opsin-tdTomato construct is injected into the AC bilaterally. Recordings are made in multiple bilateral locations of the IC (left) of awake mice. Cannulas are implanted bilaterally in the auditory cortex, and a green/blue laser is used to silence/activate the cortico-collicular pathway (right). (B) Expression of the retro AAV-Cre-GFP construct in the IC. Scale bar: 1000 μm. (C) Selective expression of the AAV-FLEX-opsin-tdTomato construct in cell bodies in layer 5 (L5) and 6 (L6) of the AC that project to the IC. Fiber and terminal labeling is also present in the medial geniculate body, a known target of auditory cortico-collicular cells. Scale bar: 2000 μm. (D) Expression of the AAV-FLEX-opsin-tdTomato construct in fibers and terminals at multiple rostro-cadual levels of the IC. Expression, as measured by fluorescence intensity (bottom), is strongest in medial portions of the central nucleus of the IC and lateral portions of the external nucleus of the IC. Scale bar: left, 1000 μm; middle and right, 500 μm.
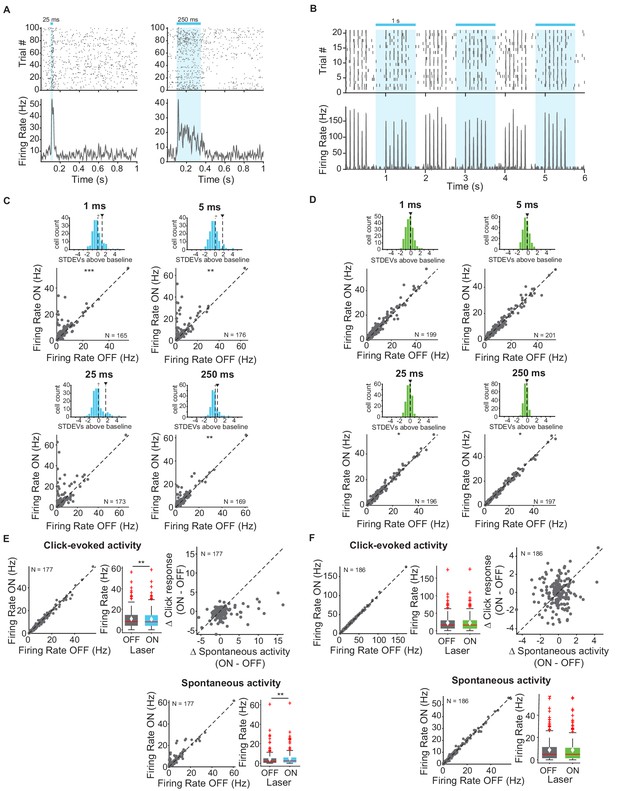
Effects of activating and inactivating cortico-collicular feedback activity on firing rate of neurons in IC in the absence of sounds and in response to sound clicks.
(A) Example IC unit response to two laser pulse durations, activation (ChR2) (blue), 25 ms (left) and 250 ms (right). Top: raster plot of spike times. Bottom: average firing rate. Blue bar indicates the timing of the laser. (B) Example unit response to clicks with (blue) and without laser (activation, ChR2). Axes same as A. We presented 6 clicks at 10 Hz every second, starting at 0 s. (C) Change in IC unit activity in response to different laser durations with cortico-collicular acitvation using ChR2 (1 ms, 5 ms, 25 ms, 250 ms). Top panels: Histogram of changes in unit activity between laser ON and laser OFF conditions, + indicates median, ∨ indicates mean. These distributions are not normally distributed (Kolmogorov-Smirnov test; 1 ms: p=3.07e-15, mean = 1.1 stdevs, median = 0.21 stdevs; 5 ms: p=4.4e-20, mean = 1.7 stdevs, median = 0.19 stdevs; 25 ms: p=5.5e-17, mean = 1.6 stdevs, median = −0.021 stdevs; 250 ms: p=3.4e-12, mean = 0.58 stdevs, median = 0.101 stdevs). Bottom panels: Firing rate of IC units in laser ON versus laser OFF conditions (Statistics for the difference across the neuronal population: 1 ms: p=5.3e-4, ON = 5.6 ± 0.6 Hz, OFF = 4.9 ± 0.5 Hz; 5 ms: p=0.0027, ON = 6.9 ± 0.7 Hz, OFF = 4.8 ± 0.5 Hz; 250 ms: p=0.005, ON = 5.6 ± 0.6 Hz, OFF = 4.8 ± 0.5 Hz)). (D) Change in IC unit activity in response to different laser durations with cortico-collicular inactivation using ChR2 (1 ms, 5 ms, 25 ms, 250 ms). Top panels: Histogram of changes in unit activity between laser ON and laser OFF conditions, + indicates median, ∨ indicates mean. These distributions are normally distributed (Kolmogorov-Smirnov test). Bottom panels: Firing rate of units in laser ON versus laser OFF conditions (Statistics for the difference across the neuronal population: 25 ms: p=0.039, ON = 9.5 ± 0.7 Hz, OFF = 9.5 ± 0.7 Hz; 250 ms: p=0.02, ON = 9.5 ± 0.8 Hz, OFF = 9.5 ± 0.8 Hz). (E) IC population responses to clicks exhibit a decrease in click response with cortico-cllicular activation using ChR2 (left; p=0.001, click ON = 10.4 ± 1.2 Hz, click OFF = 10.7 ± 1.1 Hz) and an increase in spontaneous activity (bottom; p=0.0031, spont ON = 5 ± 0.87 Hz, spont OFF = 4.3 ± 0.92 Hz). Left panel: Click-evoked firing rate of IC units on laser ON versus laser OFF conditions. Center panel: box plot of change in click-evoked firing rate On box plots, red line indicates median, white ◆ indicates mean. Right panel: Change in click response versus change in spontaneous activity due to laser activation. Bottom panel: Spontaneous activity on laser ON versus laser OFF trials. (F) IC population responses to clicks exhibit no change in activity with cortico-collicular suppression using ArchT. On box plots, red line indicates median, white ◆ indicates mean. Axes as in E.
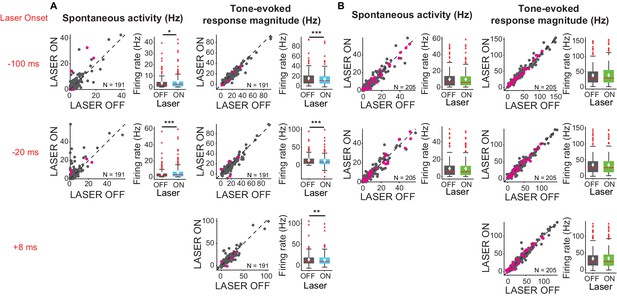
Effects of modulating feedback activity on spontaneous and tone-evoked response magnitude in IC.
Left panels: neuronal activity (spontaneous, left or tone-evoked, right) on laser ON versus laser OFF trials. Right panels: Box plots of neuronal activity (spontaneous, left or tone-evoked, right) for laser ON and laser OFF trials. Magenta circles indicate single units. On box plots, red line indicates median, white ◆ indicates mean. (A) Activating feedback using ChR2 increased IC spontaneous activity (left; −100 ms: p=0.022, ON = 4.3 ± 0.47 Hz; OFF = 3.6 ± 0.38; −20 ms: p=8.6e-6, ON = 5.6 ± 0.63, OFF = 3.7 ± 0.47) and decreased tone-evoked response magnitude (right; −100 ms: p=3.2e-5, ON = 14.2 ± 0.98 Hz; OFF = 15.5 ± 1.04 Hz; −20 ms: p=1.4e-6, ON = 11.8 ± 1.08 Hz, OFF = 13.9 ± 1 Hz; +8 ms: p=0.0034, ON = 13.03 ± 1.03, OFF = 13.7 ± 1.03). (B) Suppressing feedback using ChR2 had no effect on IC spontaneous activity or tone-evoked response magnitude.
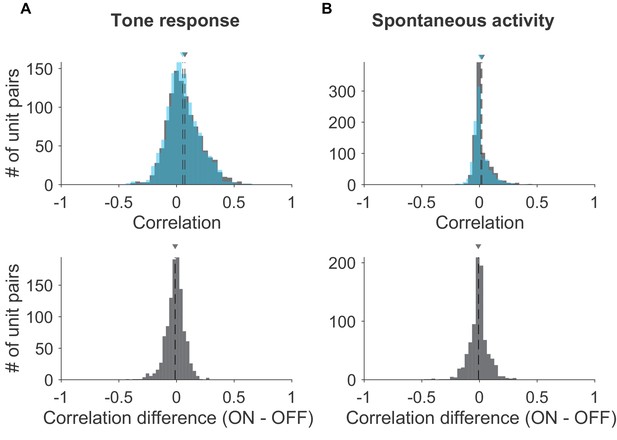
Effects of activating cortico-collicular feedback on noise correlations in IC.
(A) TOP: Distribution of noise correlations of IC tone response for laser OFF (gray) condition (mean = 0.069) and laser ON (blue, activation with ChR2) condition (mean = 0.057) BOTTOM: Distribution of change in correlation between laser ON and laser OFF conditions (mean = −0.017). (B) TOP: Distribution of noise correlations of spontaneous activity for laser OFF (gray) condition (mean = 0.021) and laser ON (blue) condition (mean = 0.012) BOTTOM: Distribution of change in correlation between laser ON and laser OFF conditions (mean = −0.008).
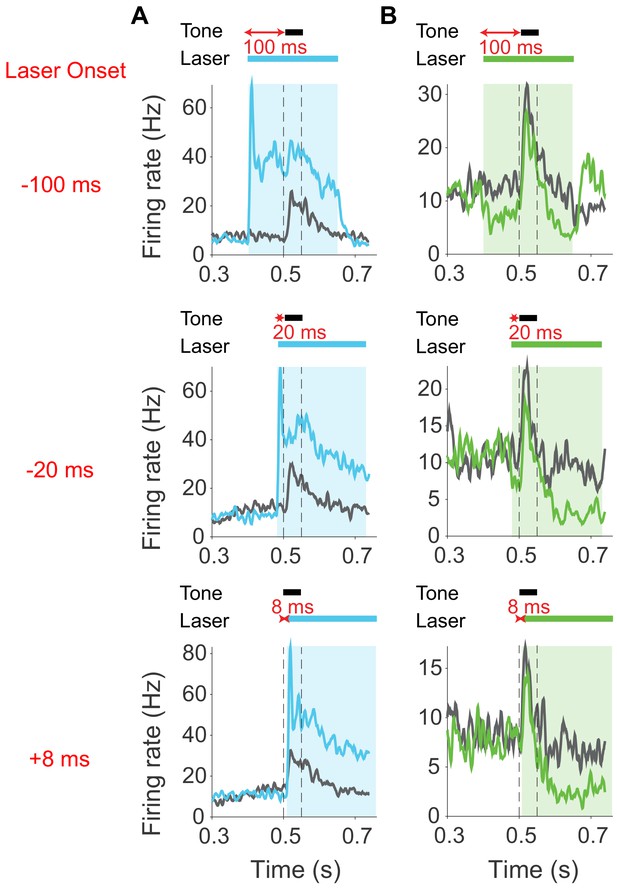
Effects of modulating cortico-collicular feedback in AC on activity in AC in two example units.
(A) Example unit with increased spontaneous and tone-evoked activity in AC with activation of cortico-collicular feedback using ChR2 in AC averaged across all frequencies. (B) Example unit with decreased spontaneous and tone-evoked activity in AC with suppression of cortico-collicular feedback using ArchT in AC averaged across all frequencies.
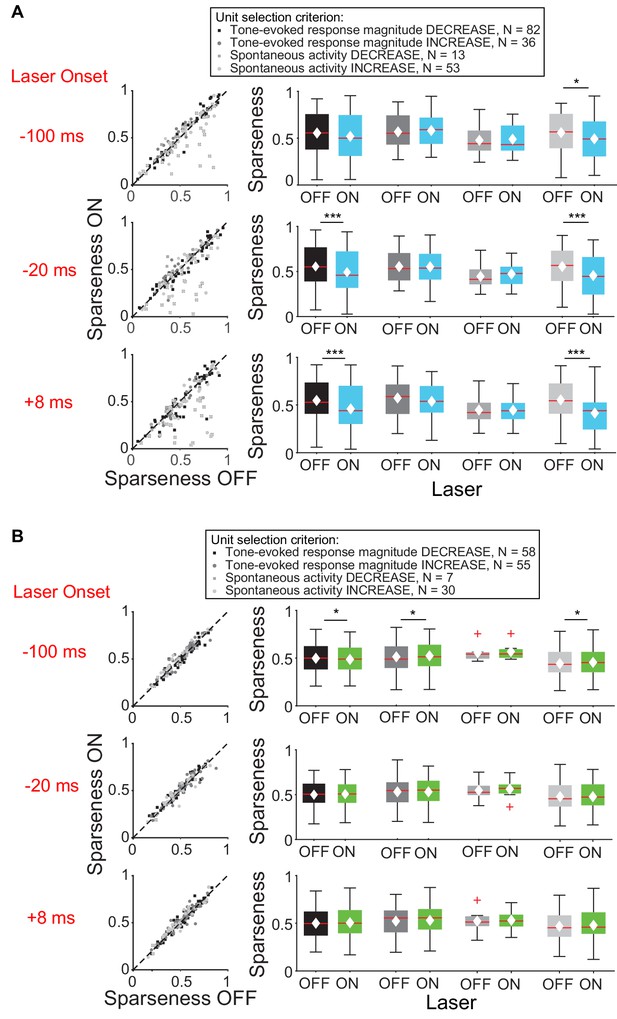
Effect of modulating direct cortico-collicular feedback at different latencies relative to tone onset on frequency selectivity in IC.
On box plots, red line indicates median, white ◆ indicates mean. (A) Left panels: Sparseness for IC units on laser ON (activation with ChR2) versus laser OFF trials. Right panels: Average sparseness for laser ON and laser OFF trials. Activating feedback using ChR2 decreased frequency selectivity in a subset of IC units (mag decrease: −20 ms, p=0.00031, sparse ON = 0.49 ± 0.027, sparse OFF = 0.55 ± 0.025; +8 ms, p=0.00029, sparse ON = 0.46 ± 0.027, sparse OFF = 0.55 ± 0.024; spont increase: −100 ms, p=0.011, sparse ON = 0.49 ± 0.032, sparse OFF = 0.56 ± 0.031; −20 ms, p=0.00018, sparse ON = 0.46 ± 0.034, sparse OFF = 0.56 ± 0.029; +8 ms, p=2.2e-6, sparse ON = 0.41 ± 0.032, sparse OFF = 0.55 ± 0.029). (B) Left panels: Sparseness for laser ON (suppression with ArchT) versus laser OFF trials. Right panels: Average sparseness for laser ON and laser off trials. Suppressing feedback using ArchT has little effect on frequency selectivity in IC (mag decrease: −100 ms, p=0.011, sparse ON = 0.53 ± 0.021, sparse OFF = 0.51 ± 0.021; mag increase: −100 ms, p=0.019, sparse ON = 0.48 ± 0.021, sparse OFF = 0.49 ± 0.021; spont increase: −100 ms, p=0.043, sparse ON = 0.46 ± 0.029, sparse OFF = 0.45 ± 0.029).
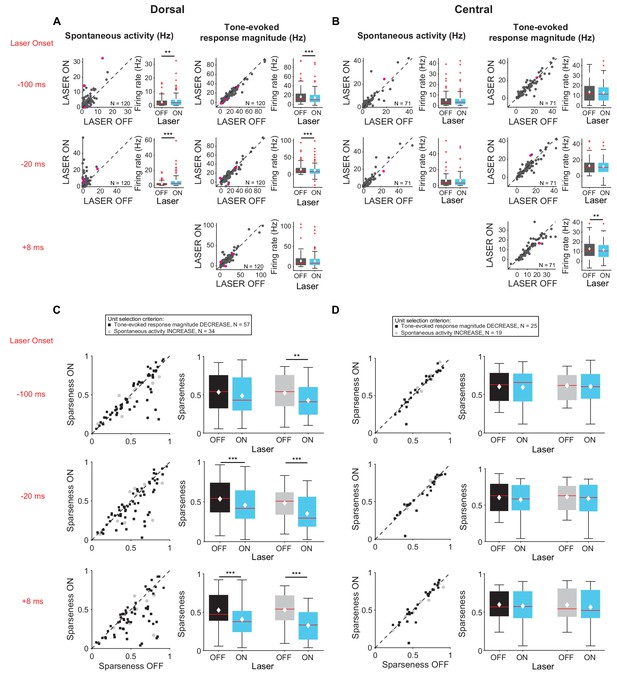
Effects of activating cortico-collicular feedback with ChR2 on responses in dorsal and central IC (A,C) and DCIC (B,D) recording sites.
On box plots, red line indicates median, white ◆ indicates mean. (A) Increase in spontaneous activity (left; −100 ms: p=0.0204, ON = 3.5 ± 0.5 Hz, OFF = 2.5 ± 0.3 Hz; −20 ms: p=2.8e-7, ON = 5.2 ± 0.7 Hz, OFF = 2.3 ± 0.3 Hz) and decrease in tone-evoked response magnitude (right, −100 ms: p=3.3e-6, ON = 14.8 ± 1.4 Hz, OFF = 16.5 ± 1.5 Hz; −20 ms: p=6.3e-7, ON = 11.8 ± 1.6 Hz, OFF = 14.7 ± 1.4 Hz) for cells in DCIC with activation of cortico-collicular feedback using ChR2. Left panels: neuronal activity (spontaneous, left or tone-evoked, right) on laser ON versus laser off trials. Right panels: Average neuronal activity (spontaneous, left or tone-evoked, right) for laser on and laser off trials. (B) Little change in spontaneous activity or tone-evoked response magnitude (right; +8 ms: p=0.0027, ON = 13 ± 1.2 Hz, OFF = 13.6 ± 1.2 Hz) for cells in CNIC with activation of cortico-collicular feedback using ChR2. Panels as in A. (C) Activating feedback with ChR2 decreases frequency selectivity in a subset of units (mag decrease: −20 ms, p=6.3e-4, sparse ON = 0.46 ± 0.03, sparse OFF = 0.53 ± 0.03; +8 ms, p=4.7e-5, sparse ON = 0.41 ± 0.03, sparse OFF = 0.53 ± 0.03; spont increase: −100 ms, p=0.0044, sparse ON = 0.43 ± 0.04, sparse OFF = 0.52 ± 0.04; −20 ms, p=0.00013, sparse ON = 0.39 ± 0.04, sparse OFF = 0.53 ± 0.04; +8 ms, p=5.2e-6, sparse ON = 0.33 ± 0.03, OFF = 0.53 ± 0.04) for cells in DCIC. Left panels: Sparseness for laser ON versus laser OFF trials. Right panels: Average sparseness for laser on and laser off trials. (D) Activating feedback has no effect on frequency selectivity of cells in CNIC. Panels as in C.
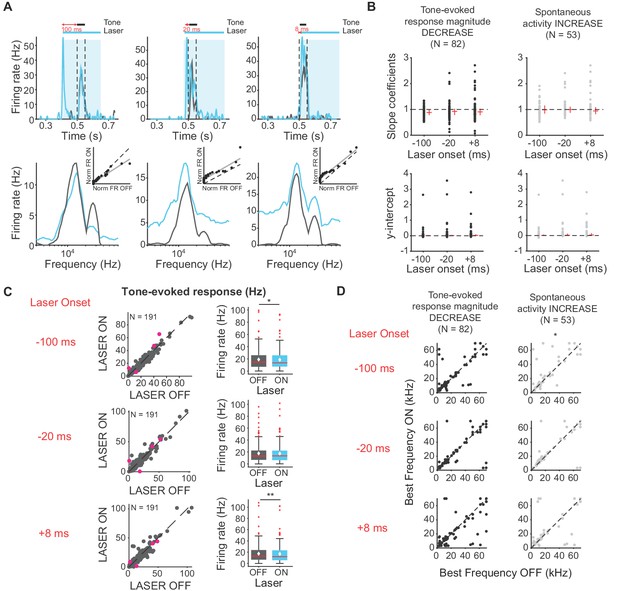
Effects of activating direct cortico-collicular feedback on frequency tuning properties of IC neurons.
(A) Example IC unit response timecourse (top) and frequency response function (bottom) for laser ON (blue) and laser off (gray) conditions (activation using ChR2). Bottom insets represent ranked linear fits for example unit. (B) Slope coefficients (top, mag decrease: −100 ms, median = 0.88; −20 ms, median = 0.92; +8 ms, median = 0.903; spont increase: −100 ms, median = 0.99; −20 ms, median = 1; +8 ms, median = 0.94) and y-intercepts (bottom, mag decrease: −100 ms, median = 0.0086; −20 ms, median = 0.014; +8 ms, median = 0.022; spont increase: −100 ms, median = 0.014; −20 ms, median = 0.025; +8 ms, median = 0.037) of ranked linear fits of the frequency response function of IC neurons , red horizontal lines indicate median, red vertical lines indicate interquartile range. (C) Left panels: Tone-evoked response for laser ON versus laser off trials. Magenta circles indicate single units. Right panels: Average tone-evoked response for laser ON and laser OFF trials. On box plots, red line indicates median, white ◆ indicates mean. Activating feedback slightly decreased tone-evoked response, averaged across 7 most preferred frequencies (−100 ms: p=0.018, ON = 19.01 ± 1.2 Hz, OFF = 18.5 ± 1.2 Hz; +8 ms: p=0.007, ON = 17.3 ± 1.2 Hz; OFF = 16.9 ± 1.2 Hz) (D) Best frequencies for laser off versus laser ON trials. Activating feedback had little effect on best frequency (spont increase: −100 ms, p=0.022).
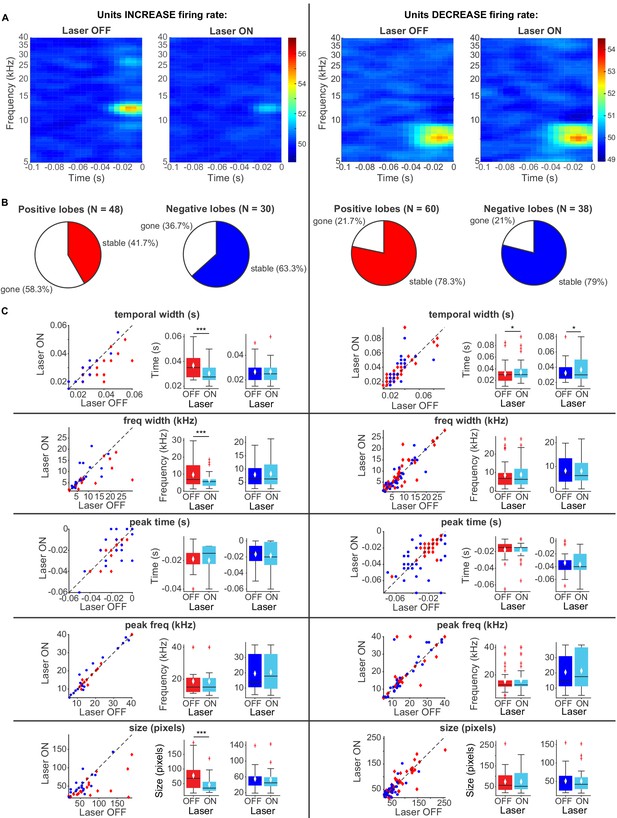
Effects of activating direct cortico-collicular feedback using ChR2 on STRF properties of IC units.
(A) Example STRF with and without activation of feedback for IC units that increase firing rate (left) and decrease firing rate (right) in response to cortico-collicular feedback activation using ChR2. (B) Number of positive and negative lobes that persisted with laser (stable). (C) Changes in STRF parameters of stable positive and negative lobes. Left panels: STRF parameter for laser OFF versus laser ON trials. Center panels: Average positive lobe STRF parameter for laser ON and laser off trials. Right panels: Average negative lobe STRF parameter for laser on and laser off trials. On box plots, red line indicates median, white ◆ indicates mean. For units with an increase in firing rate we observed a decrease in temporal width (p=0.00098, ON = 0.0303 ± 0.0018 s, OFF = 0.037 ± 0.0023 s), frequency width (p=0.00042, ON = 6.7 ± 1.1 Hz, OFF = 9.6 ± 1.8 Hz), and overall size (p=0.00036, ON = 46.05 ± 6.9 pixels, OFF = 77.5 ± 12.04 pixels) of positive lobes. For units with a decrease in firing rate we observed only a small increase in temporal width for both positive (p=0.026, ON = 0.034 ± 0.0025 s, OFF = 0.032 ± 0.0025 s) and negative (p=0.02, ON = 0.037 ± 0.0028 s, OFF = 0.032 ± 0.0024 s) lobes.
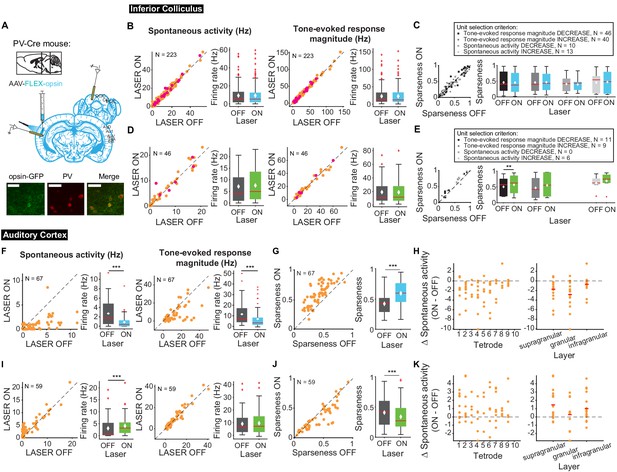
Effects of modulating PV interneurons in AC on tone-evoked responses in IC and AC.
On box plots, red line indicates median, white ◆ indicates mean. (A) Stain for PV (center) and opsin-GFP (left). Scale bar: 50 um. (B) Activating PVs had no effect on spontaneous activity or tone-evoked response magnitude in IC. Magenta circles indicate single units. (C) Activating PVs had no effect on frequency selectivity in IC. (D) Suppressing PVs had no effect on spontaneous activity or tone-evoked response magnitude in IC. Magenta circles indicate single units. (E) Suppressing PVs had weak effects on frequency selectivity in IC (mag decrease: p=0.0068, ON = 0.57 ± 0.092, OFF = 0.5 ± 0.087). (F) Activating PVs decreased spontaneous activity (p=2.2e-9, ON = 0.88 ± 0.16 Hz, OFF = 2.7 ± 0.34 Hz) and tone-evoked response magnitude (p=8.02e-7, ON = 6.9 ± 1.06 Hz, OFF = 11.5 ± 1.3 Hz) in AC. (G) Activating PVs increased frequency selectivity in putative excitatory units in AC (p=3.3e-12, ON = 0.59 ± 0.022, OFF = 0.42 ± 0.02). (H) Activating PVs affected putative excitatory units across all layers of AC. (I) Suppressing PVs increased spontaneous activity (p=5.2e-5, ON = 4.08 ± 0.54 Hz, OFF = 3.06 ± 0.55 Hz) but did not affect tone-evoked response magnitude in AC. (J) Suppressing PVs decreased frequency selectivity in AC (p=3.8e-5, ON = 0.34 ± 0.027, OFF = 0.41 ± 0.031). (K) Suppressing PVs affected putative excitatory units across all layers of AC. B,D,F,I Left panels: neuronal activity (spontaneous, left or tone-evoked, right) on laser ON versus laser OFF trials. Right panels: Average neuronal activity (spontaneous, left or tone-evoked, right) for laser ON and laser OFF trials. C,E,G,J Left panels: Sparseness for laser ON versus laser off trials. Right panels: Average sparseness for laser on and laser off trials. (H,K) Change in spontaneous activity (laser ON trials – laser off trials), left panels: for units at each tetrode; right panels: separated into supragranular: tetrodes 1–3; granular: tetrodes 5,6; infragranular: tetrodes 7–10.
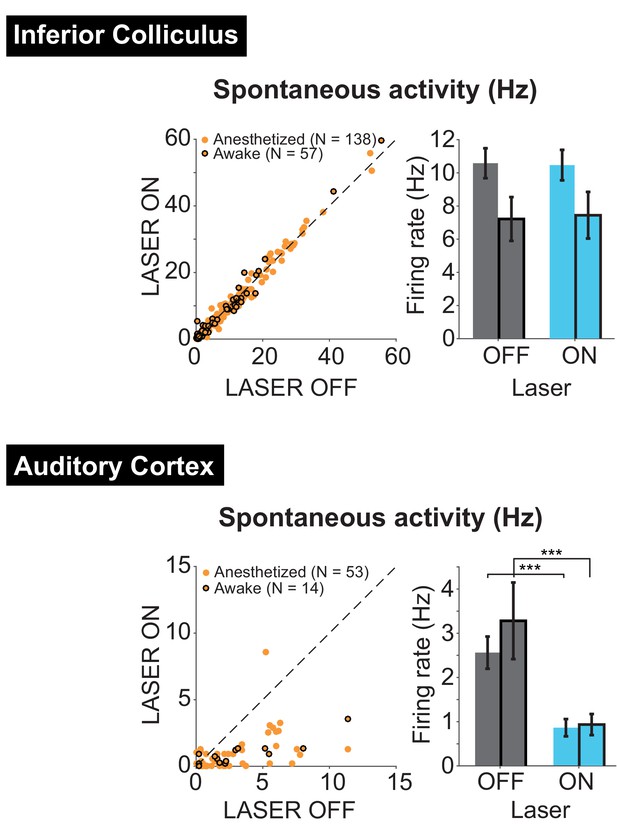
Effects of activating PV interneurons in AC on spontaneous activity in IC (top) and AC (bottom) in both anesthetized and awake (black edges) preparations.
TOP: Activation of PV interneurons in AC had no effect on spontaneous activity in IC for either awake (N = 57 units, ON = 7.4 ± 1.4 Hz, OFF = 7.2 ± 1.3 Hz) or anesthetized (N = 138, ON = 10.5 ± 0.92 Hz, OFF = 10.6 ± 0.9 Hz) recordings. BOTTOM: Activation of PV interneurons in AC decreased spontaneous activity in AC for both awake (N = 14, p=8.5e-4, ON = 0.93 ± 0.24 Hz, OFF = 3.3 ± 0.87 Hz) and anesthetized (N = 53, p=2.2e-7, ON = 0.87 ± 0.19, OFF = 2.6 ± 0.36) recordings.
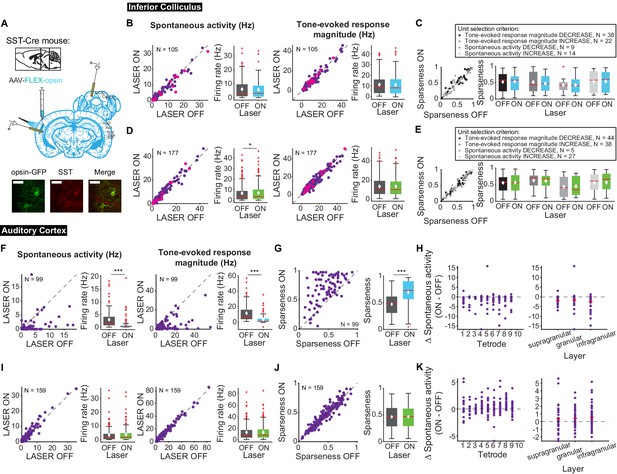
Effects of modulating SST interneurons in AC on tone-evoked responses in IC and AC.
On box plots, red line indicates median, white ◆ indicates mean. (A) Stain for SST (center) and opsin-GFP (left). Scale bar: 50 um. (B) Activating SSTs had no effect on spontaneous activity or tone-evoked response magnitude in IC. Magenta circles indicate single units. (C) Activating SSTs had no effect on frequency selectivity in IC. (D) Suppressing SSTs increased spontaneous activity (p=0.029, ON = 6.9 ± 0.66 Hz, OFF = 6.7 ± 0.63 Hz) but did not affect tone-evoked response magnitude in IC. Magenta circles indicate single units. (E) Suppressing SSTs had no effect on frequency selectivity in IC. (F) Activating SSTs decreased spontaneous activity (p=1.4e-12, ON = 0.97 ± 0.25 Hz, OFF = 2.9 ± 0.37 Hz) and tone-evoked response magnitude (p=1.3e-15, ON = 3.3 ± 0.59 Hz, OFF = 11.4 ± 1.2 Hz) in AC. (G) Activating SSTs increased frequency selectivity in putative excitatory units in AC (p=1.6e-13, ON = 0.69 ± 0.024, OFF = 0.47 ± 0.019). (H) Activating SSTs affected putative excitatory units across all layers of AC. (I) Suppressing SSTs increased spontaneous activity (p=8.1e-4, ON = 3.6 ± 0.41 Hz, OFF = 2.2 ± 0.38 Hz) but did not affect tone-evoked response magnitude in AC. (J) Suppressing SSTs had no effect on frequency selectivity in AC. (K) Suppressing SSTs affected putative excitatory units across all layers of AC. B,D,F,I Left panels: neuronal activity (spontaneous, left or tone-evoked, right) on laser on versus laser off trials. Right panels: Average neuronal activity (spontaneous, left or tone-evoked, right) for laser on and laser off trials. C,E,G,J Left panels: Sparseness for laser on versus laser off trials. Right panels: Average sparseness for laser on and laser off trials. (H,K) Change in spontaneous activity (laser on trials – laser off trials), left panels: for units at each tetrode; right panels: separated into supragranular: tetrodes 1–3; granular: tetrodes 5,6; infragranular: tetrodes 7–10.
Tables
Reagent type (species) or resource | Designation | Source or reference | Identifiers | Additional information |
---|---|---|---|---|
Strain, strain background (Mus musculus) | Pvalb-Cre mice | Jackson Laboratories | B6;129P2-Pvalbtm1(cre)Arbr/J RRID:IMSR_JAX:008069 | |
Strain, strain background (Mus musculus) | Sst-Cre mice | Jackson Laboratories | Ssttm2.1(cre)Zjh/J RRID:IMSR_JAX:013044 | |
Strain, strain background (Mus musculus) | Wild type | Jackson Laboratories | C57BL/6J RRID:IMSR_JAX:000664 | |
Strain, strain background (Mus musculus) | Cdh23 mice | Jackson Laboratories | Cdh23tm2.1Kjn/J RRID:IMSR_JAX:018399 | |
Recombinant DNA reagent | AAV9-CAG-FLEX-ChR2-tdTomato | Penn VectorCore | Addgene 18917 | |
Recombinant DNA reagent | AAV9-CAG-FLEX-ArchT-GFP | UNC Vector Core | Addgene 99039 | |
Recombinant DNA reagent | AAV9-CAG-FLEX-ArchT-tdTomato | UNC Vector Core | Addgene 28305 | |
Recombinant DNA reagent | RetroAAV2 hSyn Cre-GFP | In house | Vector generated and maintained in the di Biasi lab | |
Software, algorithm | Matlab | Mathworks | Mathworks.com RRID:SCR_001622 | |
Software, algorithm | ImageJ | NIH | RRID:SCR_003070 |
Number of recorded units and mice used in our analyses for each experimental group and condition.
Group | Recording area | Single units | Multi units | Awake total mice | Awake total units | Anesthetized total mice | Anesthetized total units | Total units |
---|---|---|---|---|---|---|---|---|
Cdh23-ChR2 | IC | 4 | 187 | 5 Male | 191 | - | - | 191 |
Cdh23-ArchT | IC | 24 | 181 | 4 Male | 205 | - | - | 205 |
PV-ChR2 | IC | 25 | 198 | 4 Male | 85 | 12 Male | 138 | 223 |
AC | 3 | 64 | 14 | 53 | 67 | |||
PV-ArchT | IC | 6 | 40 | 4 Male | 46 | - | - | 46 |
AC | 3 | 56 | 59 | - | 59 | |||
SST-ChR2 | IC | 15 | 90 | 7 Male | 76 | 8 Male | 29 | 105 |
AC | 9 | 90 | 49 | 50 | 99 | |||
SST-ArchT | IC | 16 | 161 | 2 Male 2 Female | 140 | 4 Male 2 Female | 37 | 177 |
AC | 4 | 155 | 123 | 36 | 159 |