High-phytate/low-calcium diet is a risk factor for crystal nephropathies, renal phosphate wasting, and bone loss
Figures
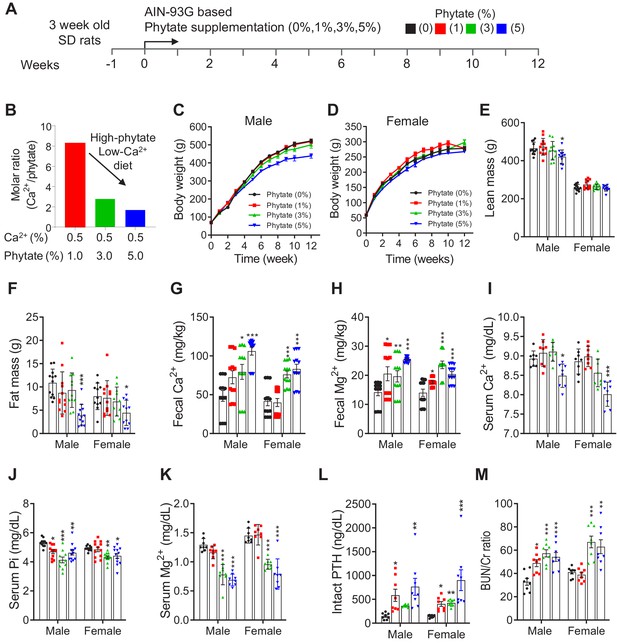
Phytate-fed rats develop secondary hyperparathyroidism with hypophosphatemia and azotemia.
(A) Experimental design. After a 1-week period of adaptation with control AIN93G diet, we fed 4-week-old male and female Sprague–Dawley rats with AIN-93G diets supplemented with 0% (control), 1%, 3%, or 5% phytate for 12 weeks. (B) Molar ratio of Ca2+ to phytate content in the AIN93G diet (0.5% Ca2+) supplemented with 1%, 3%, and 5% phytate. (C–D) Mean body weights of male (C) and female (D) rats of all groups after 12 weeks of feeding with the experimental diets. (E–F) Mean whole-body lean mass (E) and fat mass (F) values for all groups. Whol-body lean mass and fat mass were measured by nuclear magnetic resonance spectroscopy. (G–H) ICP-MS analysis of fecal excretion of Ca2+ (G) and Mg2+ (H) in all groups after 3 weeks of feeding with the experimental diets. (I–M) Serum levels of Ca2+ (I), phosphate (J), Mg2+ (K), intact PTH (L), and blood urea nitrogen (BUN)/Cr ratio (M) of all groups after 12 weeks of feeding with the experimental diets. Serum intact PTH levels were measured by enzyme-linked immunosorbent assay (ELISA). All comparisons were performed by one-way ANOVA with Tukey’s post hoc multiple comparison test or the Kruskal–Wallis test (G, H, L) for non-parametric statistical analysis of data that did not have a normal distribution. The groups in panels G and H and 5% phytate in female rats failed the normality test, but no outliers were identified. Panel L failed the normality test, one sample of 3% phytate in male rats was out of range according to ROUT (Q = 1%), but it did not alter the statistical outcome and no data were excluded from that group. All data are presented as the mean ± standard deviation (SD) for each group (n = 8 – 12 per group). *p < 0.05, **p < 0.01, ***P < 0.001 compared with controls.
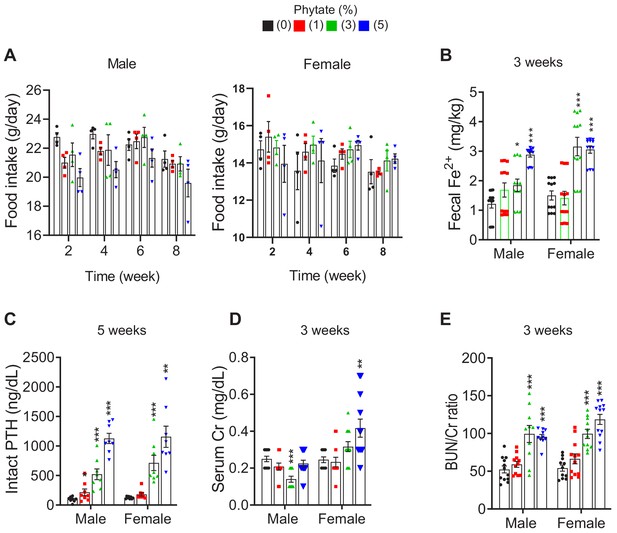
Metabolic characteristics of Sprague-Dawley rats fed control and phytate-supplemented diets.
(A) Food intake of rats fed control or phytate-supplemented diets. (B) Fecal excretion of Fe3+ in rats fed control or phytate-supplemented diets. (C–E) The serum levels of intact PTH (C), creatinine (D), and BUN/Cr ratio (E) in rats after 3 or 5 weeks on a control or a phytate-supplemented diet. All comparisons were performed by one-way ANOVA, with Tukey’s post hoc multiple comparison test or the Kruskal–Wallis (E) test for non-parametric statistical analysis of data that did not have a normal distribution. Panel (E) failed the normality test, but no outliers were identified. All data are presented as the mean ± standard deviation (SD) for each group (n = 8–12 per group). *, p<0.05 compared with controls.
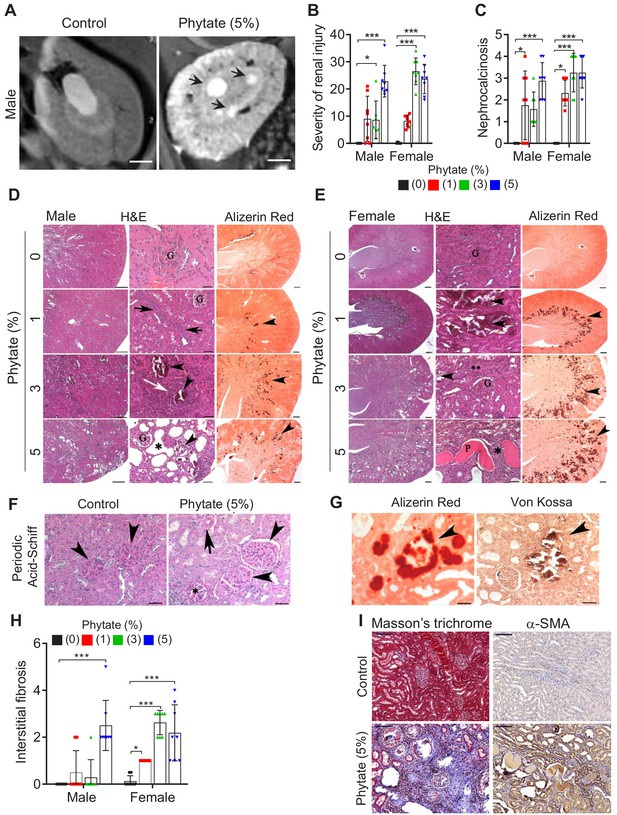
Phytate-fed rats develop crystal nephropathies.
(A) Representative T2-weighted magnetic resonance images (MRIs) of kidneys from male rats fed a 5% phytate or control diet. The arrows point to kidney cysts in a phytate-fed rat. Scale bars, 250 µm. (B–E) Kidney injury was examined by staining with hematoxylin and eosin, Alizarin Red S (AR), von Kossa (VK), or periodic acid-Schiff (PAS) staining. Scale bars in panels (D, E): left, 500 µm; center, 50 µm; right, 500 µm. Granular material within the tubules (nephrocalcinosis) (arrowheads in panels [D, E) was present in the kidneys of phytate-fed rats visualized using Alizarin Red S staining (D, E, right panels). Pathologic changes included tubular regeneration (black arrows), inflammation (white arrow), ectatic tubules (*), protein casts ('P'), interstitial fibrosis (**), and small shrunken glomeruli (G). (F) Representative PAS-stained sections from control (left) or phytate-fed (right) groups. (G) Calcification was detected using Alizarin Red S (left) and VK (right) stains. VK staining for Ca2+ was similar to that of Alizarin Red S (arrowheads). In phytate-fed rats with severe pathologic changes, glomeruli were often large (5% phytate, arrowheads) with increased PAS-positive material in the mesangium, Bowman’s capsule of the glomeruli (*), and in the basement membrane of the tubules (arrow). Kidneys from control rats were unremarkable. Scale bar, 50 µm. (H, I) Interstitial fibrosis was further detected by staining with Masson’s trichrome (H) or by immunohistochemistry using antibodies against α-smooth muscle actin (I). Scale bar, 100 µm. All comparisons were performed by one-way ANOVA, with Tukey’s post hoc multiple comparison test or the Kruskal–Wallis test (B, C, H) for non-parametric statistical analysis of data that did not have a normal distribution. The groups in panels (G) and (H), as well as the5% phytate in female rats, failed the normality test, but no outliers were identified. Panel (L) failed the normality test and one sample of 3% phytate in male rats was out of range according to ROUT (Q = 1%), but it did not alter the statistical outcome and no data were excluded from that group. All data are presented as the mean ± standard deviation (SD) for each group (n = 8–12 per group). *, p<0.05; **, p<0.01; ***, p<0.001 compared with controls.
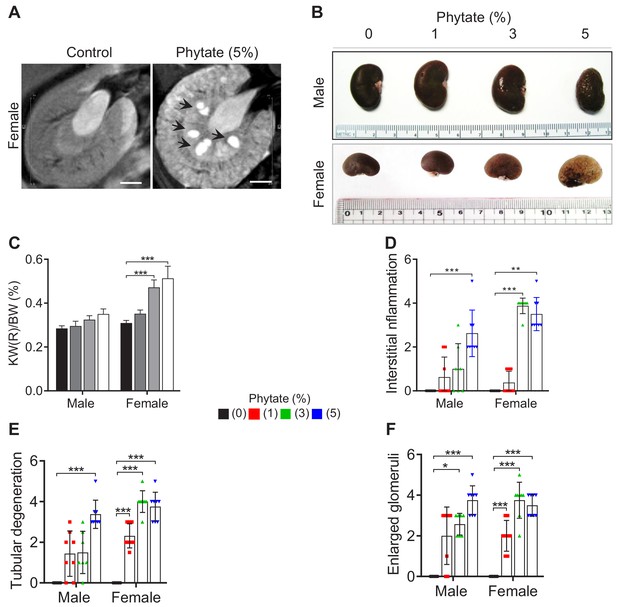
Histopathological features of the kidneys of phytate-fed rats.
(A) Representative T2-weighted magnetic resonance images (MRIs) of the kidneys of female rats fed either a control or a 5% phytate diet. (B, C) Representative gross morphological features of the kidneys (B) and kidney weight (%) relative to body weight (KW/BW) (C) of rats fed control and phytate-supplemented diets. (D–F) The renal histopathological scores for interstitial inflammation (D), tubular degeneration (E), and enlarged glomeruli (F) from rats fed control and phytate-supplemented diets. All comparisons were performed by one-way ANOVA with Tukey’s post hoc multiple comparison test or the Kruskal–Wallis (D–F) test for non-parametric statistical analysis of data that did not have a normal distribution. Panels (D–F) failed the normality test, but no outliers were identified. All data are presented as the mean ± SD for each group (n = 8 per group). *, p <0.05, compared with controls.
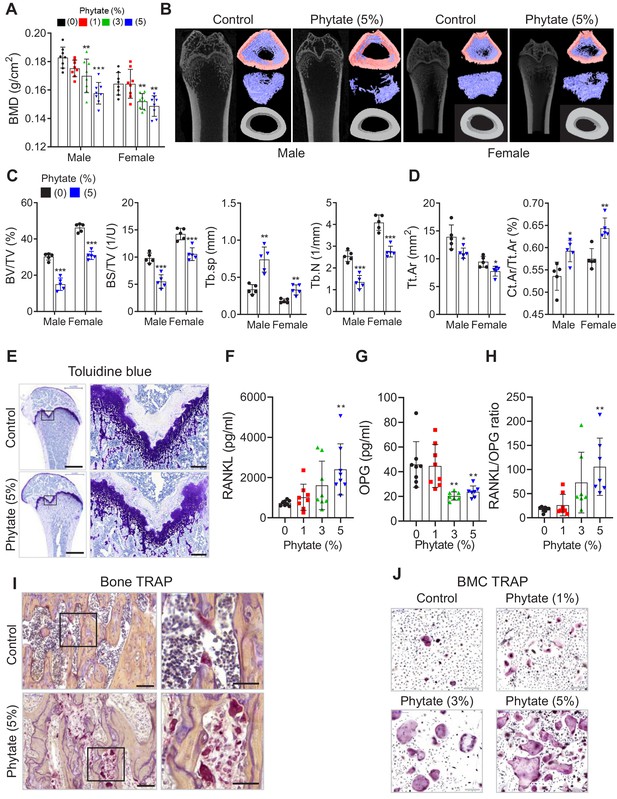
Phytate-fed rats develop severe bone loss.
(A) Bone mineral density (BMD) was examined using peripheral dual-energy X-ray absorptiometry (pDEXA) of whole femora from rats fed control or phytate-supplemented diets (n = 8 per group). (B) Three-dimensional reconstructed μ-CT images of distal femora of rats fed control or phytate-supplemented diets. (C) Analysis of secondary spongiosa of the distal femur: bone volume fractions (BV/TV), bone surface density (BS/TV), trabecular separation (Tb.Sp), and trabecular number (Tb.N). (D) Analysis of the cortical bone of the distal femur: total cross-sectional area inside the periosteal envelope (Tt.Ar) and cortical area fraction (Ct.Ar/Tt.Ar). (E) Representative toluidine blue-stained sections of decalcified femora from female rats fed control or phytate-supplemented diets. Scale bars: left, 1000 µm; right, 100 µm. (F–H) Serum levels of soluble RANKL (F), OPG (G), and sRANKL/OPG ratio (H) in female rats fed control or phytate-supplemented diets. Serum sRANKL and OPG were measured using an ELISA (n = 8 per group). (I) Representative images of tartrate-resistant acid phosphatase (TRAP)-stained, EDTA-decalcified femur sections of rats fed control (upper) or phytate-supplemented (lower) diets. Red reaction product represents TRAP+ multinucleated osteoclasts. Scale bars: left, 500 µm; right, 100 µm. (J) Bone marrow macrophages of rats fed control or phytate-supplemented diets for 12 weeks were cultured with RANK and macrophage colony-stimulating factor for 5 days. Osteoclasts were stained to detect TRAP activity. Scale bars: 200 µm. Comparisons were performed by unpaired Student’s t test (C, D), one-way ANOVA with Tukey’s post hoc multiple comparison test (F–H), or the Kruskal–Wallis test (C) for non-parametric statistical analysis of data that did not have a normal distribution. The HP-LCa2+ diet group in panel (C) failed the normality test, but no outliers were identified. All data are presented as the mean ± SD of each group ([C, D] 0% and 5% phytate, n = 5 per group; [F–H] n = 8 per group). *, p<0.05; **, p<0.01; ***, p<0.001 compared with controls.
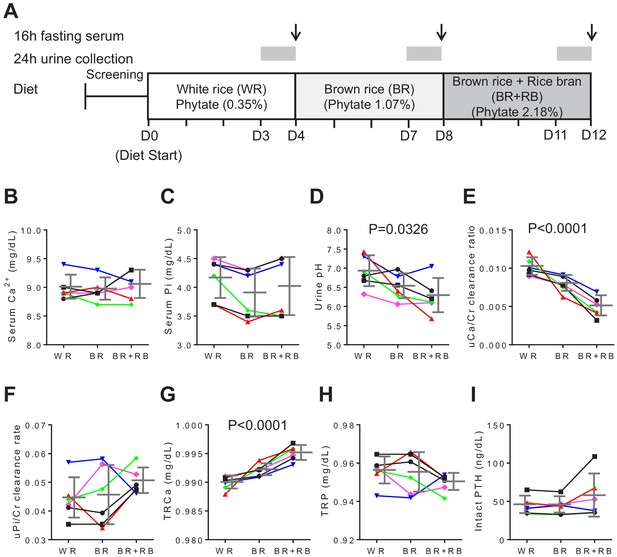
High-phytate diets dysregulate mineral and phosphate metabolism in humans.
(A) Experimental design (six healthy female volunteers). (B, C) Time course of serum levels of Ca2+ (B) and phosphate (C) for three different phytate doses. (D–H) Kinetics of urine pH (D), 24 hr urine Ca2+/Cr ratio (E), 24 hr urine Pi/Cr ratio (F), renal tubular Ca2+ reabsorption (TRCa) (G), and renal tubular phosphate reabsorption (TRP) (H) for three different phytate doses. (I) Time-course analysis of serum levels of intact PTH for different phytate doses. Data are presented as the mean ± SD for each dose (n = 6 per dose). Statistical significance was tested by repeated measure ANOVA between subjects and groups. All data are presented as the mean ± SD for each dose (n = 6 per dose).
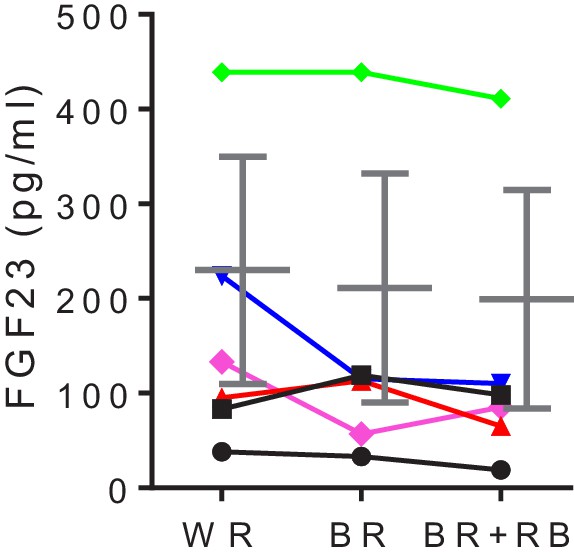
High-phytate diets dysregulate mineral and phosphate metabolism in humans.
Time-course analysis of serum levels of intact FGF23 for three consecutive phytate doses. Statistical significance was tested by repeated measure ANOVA between subjects and groups. All data are presented as the mean ± SD for each dose (n = 6 per dose).
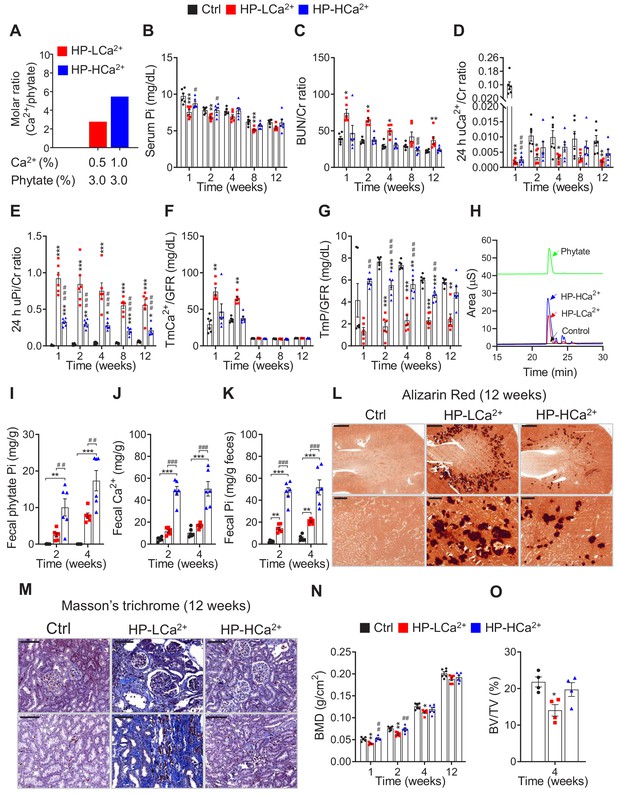
Ca2+ supplementation protects phytate-fed rats from renal phosphate wasting disorders, crystal nephropathies, and bone loss via indigestible Ca2+-phytate salts.
(A) Molar ratio of Ca2+ to phytate content when the diet was supplemented with 1.0% Ca2+. (B–C) Time-course analysis of serum Pi level (B) and BUN/Cr ratio (C) in rats fed control, HP-LCa2+, and HP-HCa2+ diets for 12 weeks. (D–G) Time-course analysis of 24 hr urinary Ca2+/Cr ratio (D), 24 hr urinary Pi/Cr ratio (E), ratio of tubular maximum reabsorption rate of renal tubular Ca2+ to glomerular filtration rate (TmCa2+/GFR) (F), and ratio of tubular maximum reabsorption of renal tubular phosphate to glomerular filtration rate (TmP/GFR) (G) in all groups during 12 weeks of experimental feeding. (H) Representative data of fecal phytate analysis by HPIC. (J–K) Time-course analysis of fecal Ca2+ (J) and Pi (K) by ICP-MS in all groups after 2 and 4 weeks. Fecal phytate phosphorus was calculated as follows: [Phytate Pi] = [([Pi] from rats fed phytate diets) − ([Pi] from rats fed control diet)]. (L–M) Representative data of Alizarin Red S staining (L) and Masson’s trichrome staining (M) of kidney sections in all groups after 12 weeks. (N–O) Bone mineral density (BMD) (N) and bone surface/volume ratio (BS/BV) (O) in all groups. All comparisons were conducted by one-way ANOVA with Tukey’s post hoc multiple comparison test. All data are presented as the mean ± SD for each group (n = 4–6 per group). *, p<0.05; **, p<0.01; ***, p<0.001, compared with controls. #, p<0.05; ##, p<0.01; ###, p<0.001, compared with HP-LCa2+ diet group.
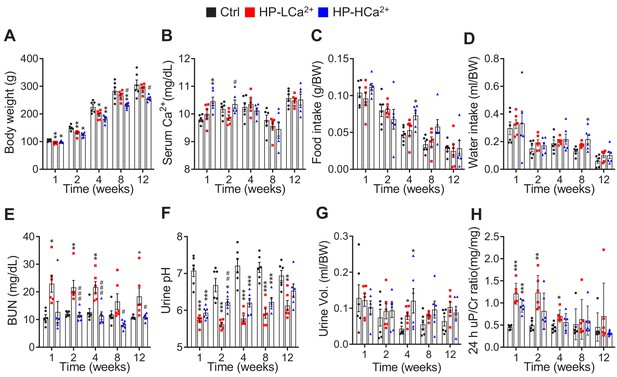
Ca2+ supplementation protects rats fed phytate from crystal nephropathies.
(A–F) Time-course analysis of body weight (A), serum Ca2+ (B), food intake (C), water intake (D), serum BUN (E), urine pH (F), urine volume (G), and 24-h urinary protein/Cr ratio (H) in rats fed control, HP-LCa2+ , and HP-HCa2+ diets for 12 weeks. The data are presented as the mean ± SD for each group (n = 6 per group).
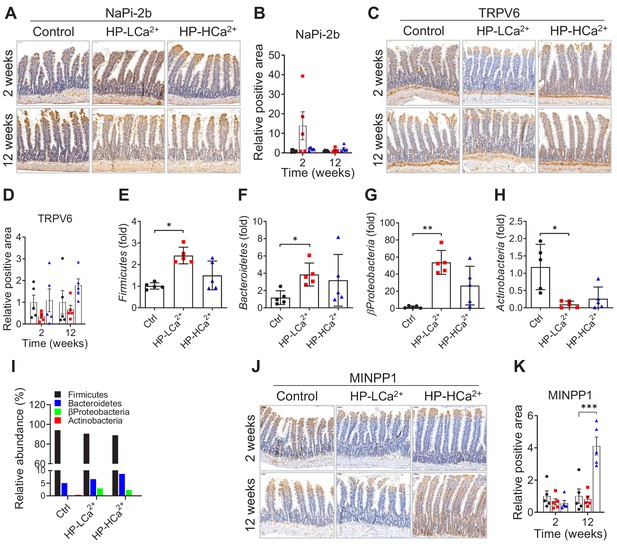
The expression levels of intestinal MINPP1, TRPV6, and NaPi-2b and real-time PCR analysis of stool microbiota in rats fed control, HP-LCa2+ , and HP-HCa2+ diets for 12 weeks.
(A, C) Time-course analysis of immunohistochemical staining of formalin-fixed intestinal sections for anti-NaPi-2b (A) and anti-TRPV6 (B) from rats fed control, HP-LCa2+ , and HP-HCa2+ diets for 12 weeks. (E–H) Real-time PCR analysis of microbiota in the stool of rats fed control, HP-LCa2+ , and HP-HCa2+ diets after 8 weeks. (I) Relative abundance of microbiota composition was calculated from real-time PCR. (J) Time-course analysis of immunohistochemical staining of formalin-fixed intestinal sections for anti-MINPP1 from rats fed control, HP-LCa2+ , and HP-HCa2+ diets for 12 weeks. (B, D, K) The relative positive area was quantified from the immunohistochemical staining of intestinal sections for anti-NaPi-2b (A), anti-TRPV6 (C), anti-MINPP1 (J) using ImageJ software (NIH, Bethesda, MD, USA). The data are presented as the mean ± SD for each group (n = 5–6 per group). All comparisons were conducted using two-way ANOVA with Tukey’s post hoc multiple comparison testing. All data are presented as the mean ± SD of each group (n = 4–6 per group). *, p<0.05; **, p<0.01; ***, p<0.001, compared with controls.
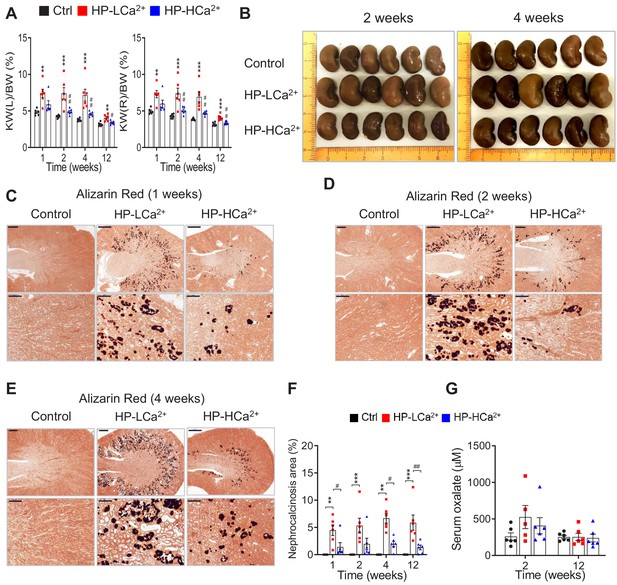
Ca2+ supplementation protects rats fed phytate from crystal nephropathies.
(A–D) Time-course analysis of kidney weight (%) relative to body weight (KW/BW) (A), representative gross morphological features of kidneys (B, C) and Alizarin red S staining (D–F) of kidney sections from rats fed control, HP-LCa2+ , and HP-LCa2+ diets. (G) The nephrocalcinosis area was quantified from the Alizarin Red S staining of kidney sections using ImageJ software (NIH, Bethesda, MD, USA). All comparisons were conducted by one-way ANOVA with Tukey’s post hoc multiple comparison test. All data are presented as the mean ± SD for each group (n = 4–6 per group). *, p<0.05; **, p<0.01; ***, p<0.001, compared with controls. #, p<0.05; ##, p<0.01; ###, p<0.001, compared with HP-LCa2+ diet group.
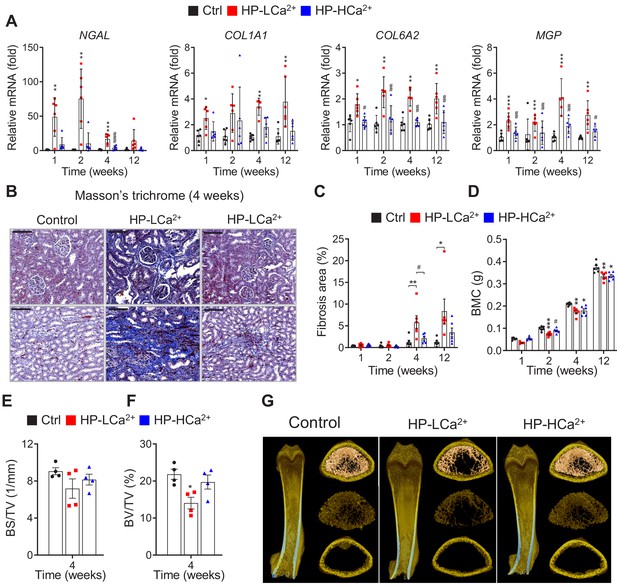
Ca2+ supplementation protects phytate-fed rats from renal fibrosis and skeletal abnormalities.
(A) Time-course analysis of renal expression of NGAL, COL1A1, COL6A2, and MGP in rats fed control, HP-LCa2+ , and HP-HCa2+ diets for 12 weeks. (B) Masson’s trichrome staining from rats fed control, HP-LCa2+, and HP-HCa2+ diets. (C) Renal fibrosis area was quantified from Masson’s trichrome staining of kidney sections using ImageJ software. (D–H) Time course analysis of BMC (D), bone surface density (BS/TV) (E), and percent bone volume (BV/TV) (F) from rats fed control, HP-LCa2+, and HP-HCa2+ diets. Representative three-dimensional reconstructed µ-CT images of distal femora (G) from rats fed control, HP-LCa2+ , and HP-HCa2+ diets. All comparisons were conducted using two-way ANOVA with Tukey’s post hoc multiple comparison testing or the Kruskal–Wallis test (A) for non-parametric statistical analysis of data that did not have a normal distribution. Panel (A) failed the normality test, but no outliers were identified. All data are presented as the mean ± SD of each group (n = 4–6 per group). *, p<0.05; **, p<0.01; ***, p<0.001, compared with controls. #, p<0.05; ##, p<0.01; ###, p<0.001, compared with HP-LCa2+ diet group.
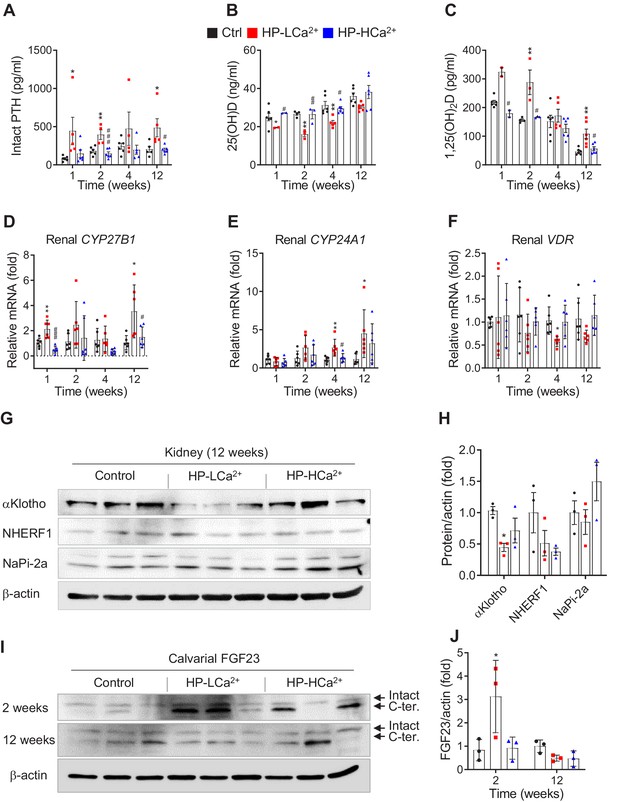
Phytate-mediated Ca2+ deficiency promotes vitamin D insufficiency and renal phosphate wasting independent of FGF23 expression.
(A–C) Time-course analysis of serum levels of intact PTH (A), 25(OH)D (B), and 1,25(OH)2D (C) in rats fed control, HP-LCa2+, and HP-HCa2+ diets. For the early measurement of 25(OH)D (B) and 1,25(OH)2D, we pooled the sera from 2 to 3 rats. (D–F) Time-course analysis of renal CYP27B1 (D), CYP24A1 (E), and VDR (F) in rats fed control, HP-LCa2+, and HP-HCa2+ diets. (G) Immunoblot analysis of renal αKlotho, NHERF1, NaPi-2a in rats fed control, HP-LCa2+, and HP-HCa2+ diets. (H) The levels of renal proteins were quantified using ImageJ software (NIH, Bethesda, MD, USA). (I) Immunoblot analysis of calvarial FGF23 in rats fed control, HP-LCa2+, and HP-HCa2+ diets. (J) The levels of total calvarial FGF23 were quantified using ImageJ software. All comparisons were conducted using two-way ANOVA with Tukey’s post hoc multiple comparison testing or the Kruskal–Wallis test (A–C) for non-parametric statistical analysis of data that did not have a normal distribution. Panels (A–C) failed the normality test, but no outliers were identified. All data are presented as the mean ± SD of each group (n = 4–6 per group). *, p<0.05; **, p<0.01; ***, p<0.001, compared with controls.
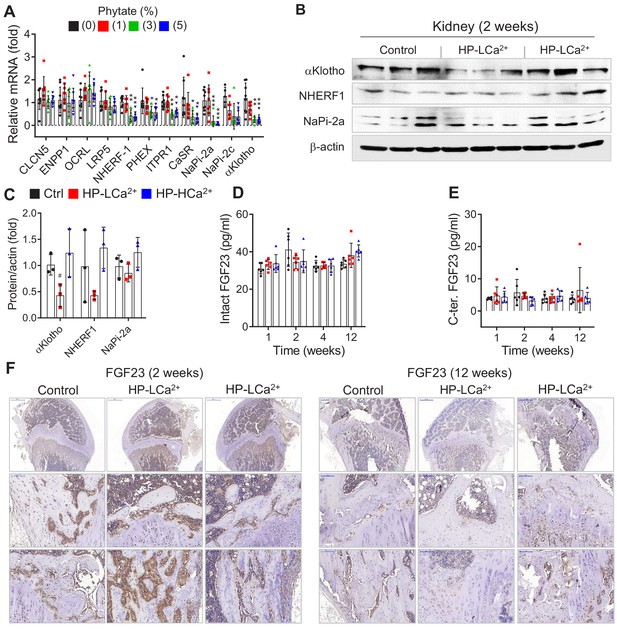
High phytate-induced renal phosphate wasting is independent of FGF23.
(A) The renal expression of downregulated genes associated with Ca2+ and phosphate homeostasis in rats fed control or phytate-supplemented diets (n = 8 per group). (B) Results of immunoblot analyses and protein levels of renal aKlotho, NHERF1, and NaPi-2a after 2 weeks for rats fed control, HP- LCa2+ , and HP-HCa2+ diets. (C) The levels of renal proteins were quantified using ImageJ software (n = 6 per group). (D, E) Time course analysis of serum levels of intact FGF23 (D) and C-terminal FGF23 (E) in rats fed control, HP-LCa2+ , and HP-HCa2+ diets. (F) Representative immunohistochemical staining of EDTA-decalcified femur sections for FGF23 from rats fed control, HP-LCa2+ , and HP-HCa2+ diets. All data are presented as the mean ± SD of each group (n = 3–8 per group). *, p<0.05; **, p<0.01; ***, p<0.001, compared with controls.
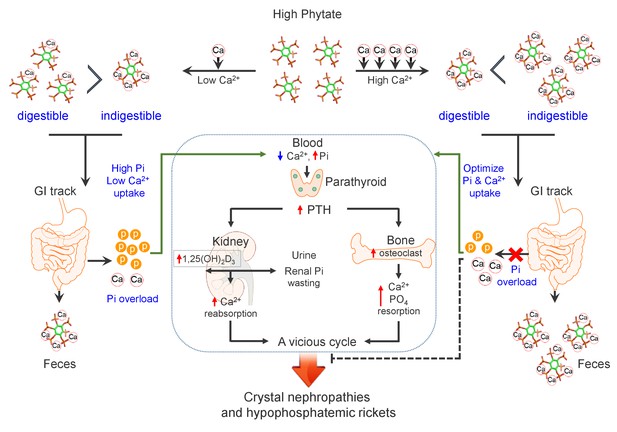
Model for the effects of phytate diets supplemented with low-Ca2+ or high-Ca2+ on Ca2+ and phosphate homeostasis.
In the presence of a low-Ca2+ diet (Ca2+/phytate <5), phytate can adversely affect Ca2+ and phosphate homeostasis in normal healthy rats by inhibiting intestinal Ca2+ absorption while increasing phosphate overload through phytate hydrolysis in the intestine. These effects lead to crystal nephropathies, severe renal phosphate wasting, and pathologic bone loss via increased PTH and 1,25(OH)2D3. By contrast, in the presence of a high-Ca2+ diet, phytate forms multiple Ca2+-phytate salts, which makes them indigestible and unabsorbable in vivo, leading to their excretion in the feces as undigested Ca2+-phytate salts. This process prevents a vicious cycle of intestinal phosphate overloading and renal phosphate wasting while improving intestinal Ca2+ bioavailability, which eventually alleviates the detrimental effects of excess phytate hydrolysis.
Tables
Reagent type (species) or resource | Designation | Source or reference | Identifiers | Additional information |
---|---|---|---|---|
Genetic reagent (Rattus norvegicus) | Sprague Dawley rat | Orient Bio, Seoul, Korea | Developed by Sprague Dawley, Inc. | |
Chemical compound, drug | AIN-93G | Dyets Inc. | DYET# 10700 | Control diet |
Chemical compound, drug | Phytic acid | Sigma-Aldrich | Cat. #: P-8810 | Phytate diet |
Chemical compound, drug | HNO3 | Sigma-Aldrich | Cat. #: 438073 | Fecal mineral analysis |
Chemical compound, drug | HF | Sigma-Aldrich | Cat. #: 695068 | Fecal mineral analysis |
Chemical compound, drug | HCl | Sigma-Aldrich | Cat. #: H1758 | Fecal mineral analysis |
Chemical compound, drug | EDTA | Sigma-Aldrich | Cat. #: 93283 | Fecal phytate analysis |
Chemical compound, drug | NaOH | Sigma-Aldrich | Cat. #: 415413 | Fecal phytate analysis |
Chemical compound, drug | Calcium | Beckman Coulter | OSR6113 | Serum/urine biochemistry |
Chemical compound, drug | Phosphate | Beckman Coulter | OSR6122 | Serum/urine biochemistry |
Chemical compound, drug | BUN (Urea) | Beckman Coulter | OSR6134 | Serum/urine biochemistry |
Chemical compound, drug | Creatinine | Beckman Coulter | OSR6178 | Serum/urine biochemistry |
Chemical compound, drug | Magnesium | Beckman Coulter | OSR6189 | Serum/urine biochemistry |
Chemical compound, drug | Urine protein | Beckman Coulter | OSR6170 | Urine biochemistry |
Commercial assay or kit | Rat intact PTH | Immutopics | Cat. #: 60–2500 | ELISA kit |
Commercial assay or kit | Human PTH | Abcam | Cat. #: ab230931 | ELISA kit |
Commercial assay or kit | Rat soluble RANKL | Immundiagnostik | Cat. #: K1019 | ELISA kit |
Commercial assay or kit | Rat osteoprotegerin | Alpco Immunoassay | Cat. #: 30–1020 | ELISA kit |
Commercial assay or kit | Rat intact FGF23 | Elabscience | Cat. #: E-EL-R3031 | ELISA kit |
Commercial assay or kit | Rat C-terminal FGF23 | Elabscience | Cat. #: E-EL-RB0377 | ELISA kit |
Commercial assay or kit | Human FGF23 | Elabscience | Cat. #: E-EL-H1116 | ELISA kit |
Commercial assay or kit | 25(OH)-Vitamin D | Abbott/USA | ARCHITECT 25-OH Vitamin D | Chemiluminescence microparticle immunoassay |
Commercial assay or kit | 1,25-(OH)two vitamin D | DIAsoure | 1,25(OH)2-VIT,D-RIA-CT/Belgium | Radioimmunoassay |
Commercial assay or kit | Hematoxylin solution | Thermo scientific | Cat. #: TA-125-MH | H&E staining |
Commercial assay or kit | Eosin Y solution | Sigma-Aldrich | Cat. #: 318906 | H&E staining |
Commercial assay or kit | Alizarin Red S | Sigma-Aldrich | Cat. #:130-22-3 | Kidney calcium staining |
Commercial assay or kit | Von Kossa stain kit | Abcam | Cat. #:ab150687 | Kidney calcium staining |
Commercial assay or kit | Trichrome stain kit | Abcam | Cat. #:ab150686 | Kidney fibrosis staining |
Commercial assay or kit | TRAP kit | Sigma-Aldrich | Cat. #:387A | Bone ostaoclast |
Commercial assay or kit | Oxalate (oxalic acid) Colorimetric assay kit | BioVision | Cat. #: K663 | ELISA kit |
Commercial assay or kit | QIAamp Fast DNA Stool Mini Kit | Quigen | Cat. #:51604 | Stool DNA prepraration |
Commercial assay or kit | THUNDERBIRD SYBR qPCR mix | Toyobo | Cat. #:QPS-101 | |
Commercial assay or kit | TB Green Premix Ex Taq | TaKaRa | RR420L | |
Commercial assay or kit | Antibody diluent with background-reducing components | DaKo | Cat. #:S3022 | IHC |
Commercial assay or kit | Protein block, serum free | DaKo | Cat. #:X0909 | IHC |
Commercial assay or kit | Liquid DAB + substrate chromogen system | DaKo | Cat. #:K3468 | IHC |
Peptide, recombinant protein | Mouse RANKL | R and D Systems | Cat. #:462-TEC-010 | In vitro osteoclast |
Peptide, recombinant protein | Mouse M-CSF | R and D Systems | Cat. #: 416 ML | In vitro osteoclast |
Antibody | Mouse monoclonal β-actin | Santa Cruz | Cat. #: AC-15 | WB (1:1000) |
Antibody | Rabbit polyclonal FGF23 | Bioss | Cat. #: bs-5768R | WB (1:200) IHC (1:100) |
Antibody | Rabbit polyclonal αklotho | Novus Biologicals | Cat. #: NBP1-76511 | WB (1:500) |
Antibody | Rabbit polyclonal SLC34A1/NaPi-2a | Abcam | Cat. #: ab151129 | WB (1:500) |
Antibody | Rabbit polyclonal SLC34A2/NaPi-2b | LSBio | Cat. #: LS-C782282 | IHC (1:100) |
Antibody | Rabbit polyclonal α-SMA | Abcam | Cat. #:ab5694 | IHC (1:100) |
Antibody | Rabbit polyclonal TRPV6 | Novus Biologicals | Cat. #: NBP2-32372 | IHC (1:100) |
Antibody | Rabbit polyclonal MINPP1 | LSBio | Cat. #: LS-C368910 | IHC (1:100) |
Software, algorithm | GraphPad Prism 8.0 | GraphPad software | Graph and statistics |
Additional files
-
Supplementary file 1
Composition of the AIN-93G rodent diets for experimental studies with rats.
- https://cdn.elifesciences.org/articles/52709/elife-52709-supp1-v1.docx
-
Supplementary file 2
The nutrient compositions for three different diets.
- https://cdn.elifesciences.org/articles/52709/elife-52709-supp2-v1.docx
-
Supplementary file 3
Biochemical properties during three consecutive diets.
- https://cdn.elifesciences.org/articles/52709/elife-52709-supp3-v1.docx
-
Supplementary file 4
Phylum- and class-specific primers for real-time PCR.
- https://cdn.elifesciences.org/articles/52709/elife-52709-supp4-v1.docx
-
Supplementary file 5
Biochemical and structural properties of mammalin, gut microbial, and bacterial phytases.
- https://cdn.elifesciences.org/articles/52709/elife-52709-supp5-v1.docx
-
Supplementary file 6
The qRT-PCR primer lists.
- https://cdn.elifesciences.org/articles/52709/elife-52709-supp6-v1.docx
-
Transparent reporting form
- https://cdn.elifesciences.org/articles/52709/elife-52709-transrepform-v1.docx