The structure of the endogenous ESX-3 secretion system
Figures
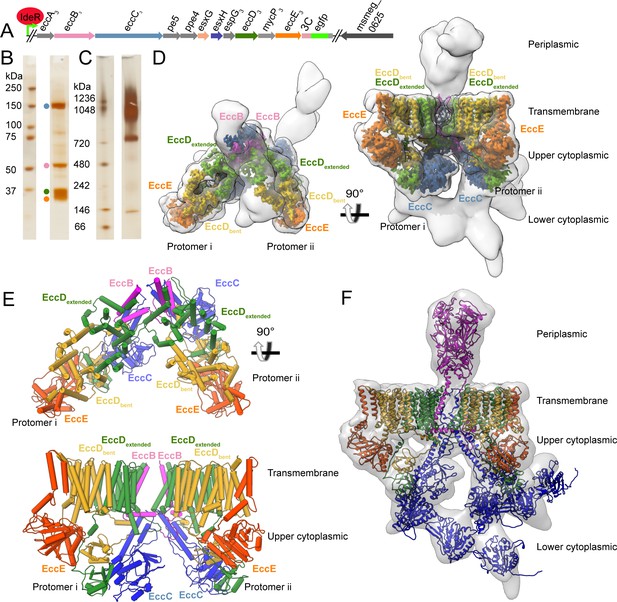
Overview of the ESX-3 tagging, purification, and structure.
(A) The ESX-3 operon in M. smegmatis and the placement of the purification tag. Genomic deletion of ideR derepresses ESX-3 to boost expression for purification. (B) SDS-PAGE of purified ESX-3 shows four major bands corresponding to EccB3, EccC3, EccD3, and EccE3. (C) Blue native page of the purified ESX-3 complex shows a large molecular weight band around 900 kDa. (D) Merged maps of all focused refinement maps (gray transparency) of the ESX-3 dimer filtered to 10 Å resolution. The transmembrane and upper cytoplasmic focused maps (3.7 Å) are segmented by subunit showing one copy per protomer of EccB3 (pink), EccC3 (blue), EccE3 (orange), EccD3-bent (yellow), and EccD3-extended (green). (E) Atomic models of the transmembrane and upper cytoplasmic regions. (F) A combined map of the full complex filtered to 10 Å resolution (gray transparency) with full models for each protein, EccD3-bent (yellow), EccD3-extended (green), EccE3 (orange), EccC3 (blue), and EccB3 (pink).
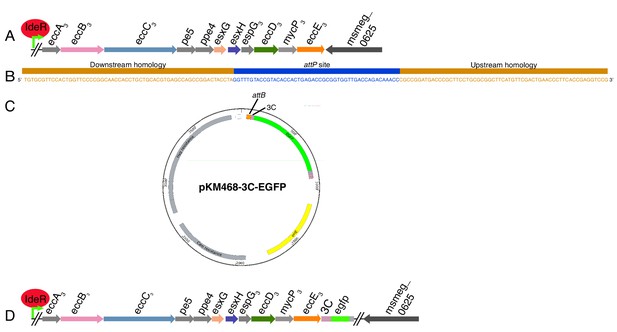
ORBIT tagging of the chromosomal copy of EccE3.
(A) Diagram of the ESX-3 operon in M. smegmatis mc(2)155. (B) The targeting oligo used to insert the attP site into the chromosome. (C) The tagging plasmid.(D) Cartoon of the resulting change to the chromosome after ORBIT.
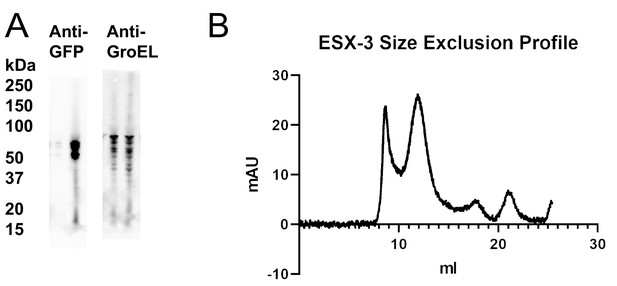
ESX-3 dimer purification optimization.
(A) Western blot of solubilized cell material with EccE3 tagged with EGFP. Lane 1, wild type background. Lane 2, ΔideR background. (B) Size exclusion profile for the ESX-3 dimer purified on a Superose 6 10/300column.
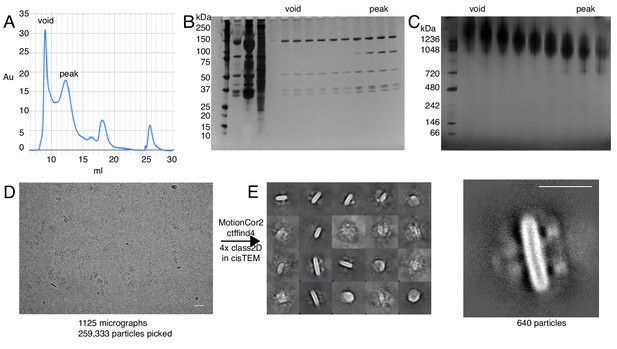
Examination of the void volume.
(A) Size exclusion profile with void volume and peak fractions indicated. (B) SDS-PAGE of all fractions from the beginning of elution until the end of the peak fraction. (C) BN-PAGE of the void and peak fractions (D) Cryo-EM of the void volume. Scale bar 50 nm (E) 2D class averages of the particles picked from the void volume, some classes reveal possible higher order oligomers. Scale bar 20 nm.
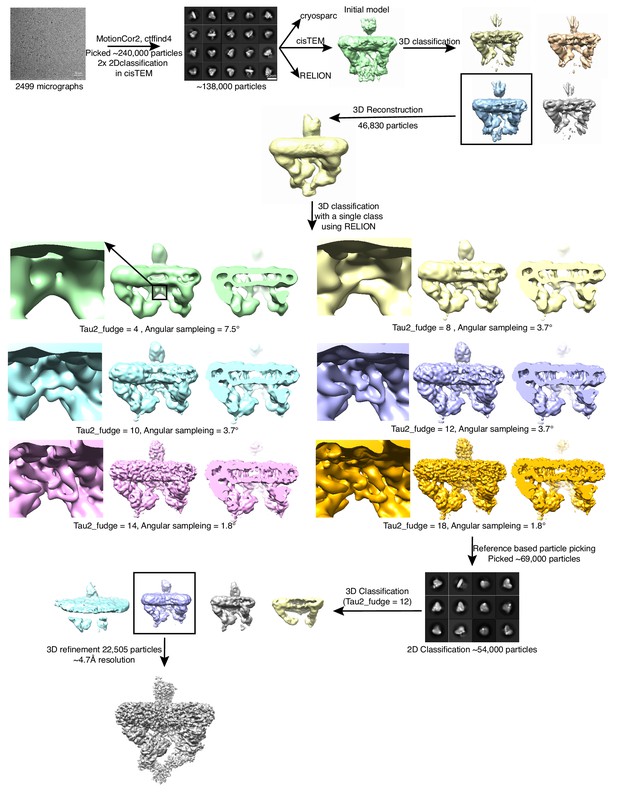
Initial data collection and initial model generation.
An initial data set was collected on a Talos Arctica microscope. The final refinement from this data processing was used as the starting model for future data processing.
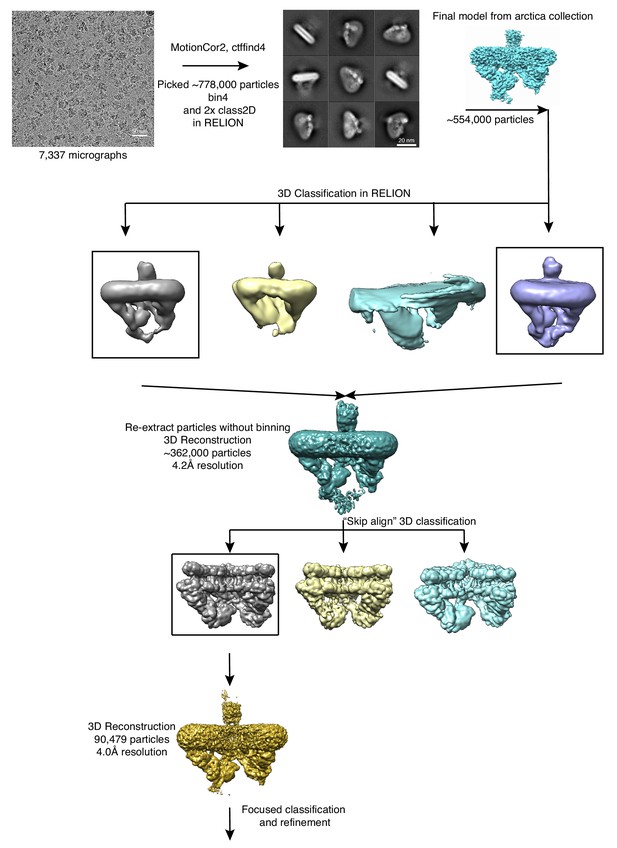
Data processing workflow for final data collection.
Data was collected on a Titan Krios. Particles from the final reconstruction were subsequently used for focused classification and refinement.
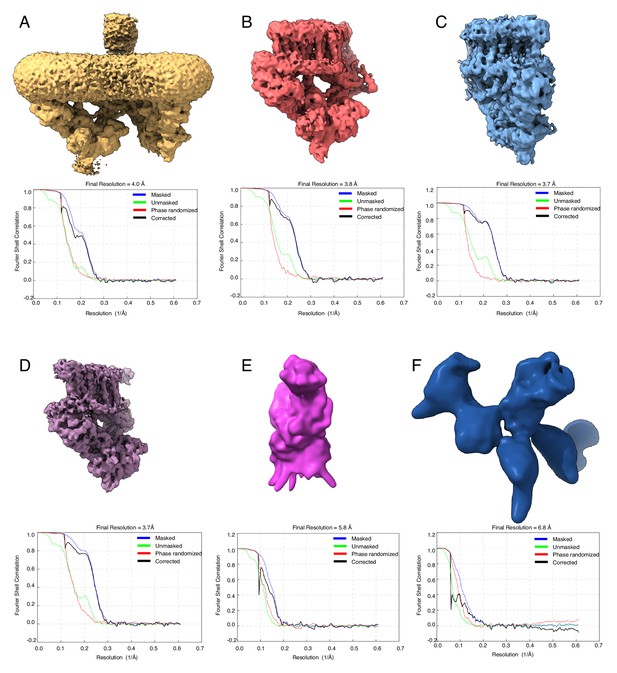
Consensus and focused refinements.
(A) Consensus refinement map and FSC curve for the ESX-3 complex. Focused refinement maps and FSC curves for (B) the left protomer of the ESX-3 complex, (C) the right protomer of the ESX-3 complex, (D) a symmetry expanded protomer, (E) the periplasmic domain of EccB and (F) the lower ATPase domains of EccC. All maps are unsharpened and unmasked.
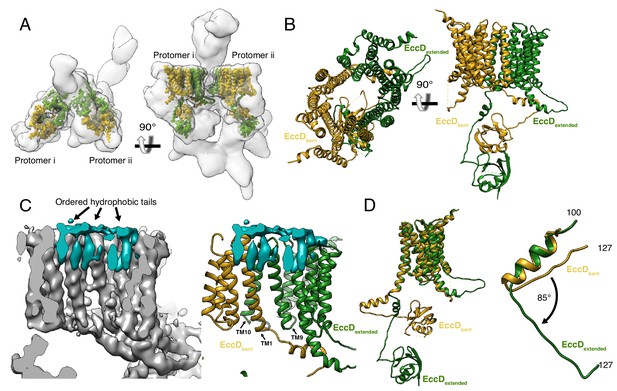
The structure of EccD3.
(A) EccD3-bent (yellow) and EccD3-extended (green) in the context of the overall ESX-3 dimer (gray transparency). (B) Atomic models of EccD3-bent and EccD3-extended (C) An unsharpened electron microscopy density map of the ESX-3 dimer shows extra densities consistent with lipid or detergent molecules (teal) on the periplasmic face of the EccD3 cavity. (D) EccD3-bent (yellow) and EccD3-extended (green) aligned based on the transmembrane regions shows two distinct conformations of the EccD3 cytoplasmic domains. Amino acids 100–127 of EccD3 adopt a bent (yellow) and an extended (green) conformation.
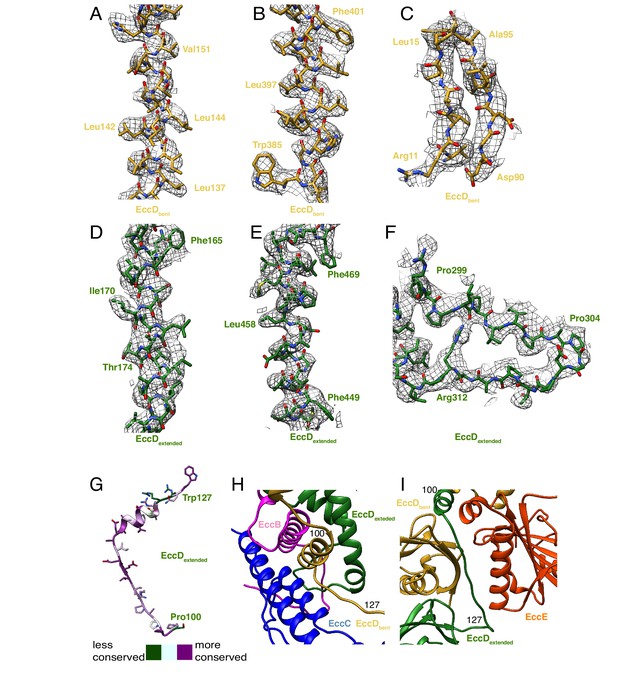
EccD3 map and model.
Map to model fits for EccD3-bent for (A) transmembrane helix 1, amino acids 136–153, (B) transmembrane α-helix 1, amino acids 385–402, and (C) soluble domain β-strands amino acids 10–15 and 90–96. Map to model fits for EccD3-extended for (D) transmembrane helix 1, amino acids 163–181 (E) transmembrane helix 11, amino acids 446–469 (F) soluble loop, amino acids 297–315. (G) Conservation mapping onto the residues of the EccD3 linker, amino acids 100–127. (H) Amino acids 100–127 from EccD3-bent (yellow) interact with EccB3 (pink) and EccC3 (blue). (I) Amino acids 100–127 of EccD3-extended (green), in a distinct conformation, interact with EccE3 (orange) and EccD3-bent (yellow).
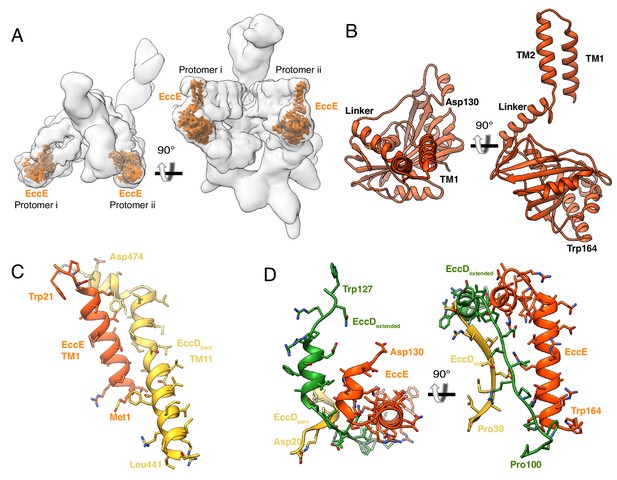
The structure and protein-protein interactions of EccE3.
(A) The placement of EccE3 in the overall ESX-3 dimer. (B) Atomic model of EccE3 (C) Transmembrane helix 1 of EccE3 interacts with transmembrane helix 11 of EccD3-bent (D) Two soluble helices of EccE3 interact with EccD3-extended and EccD3-bent.
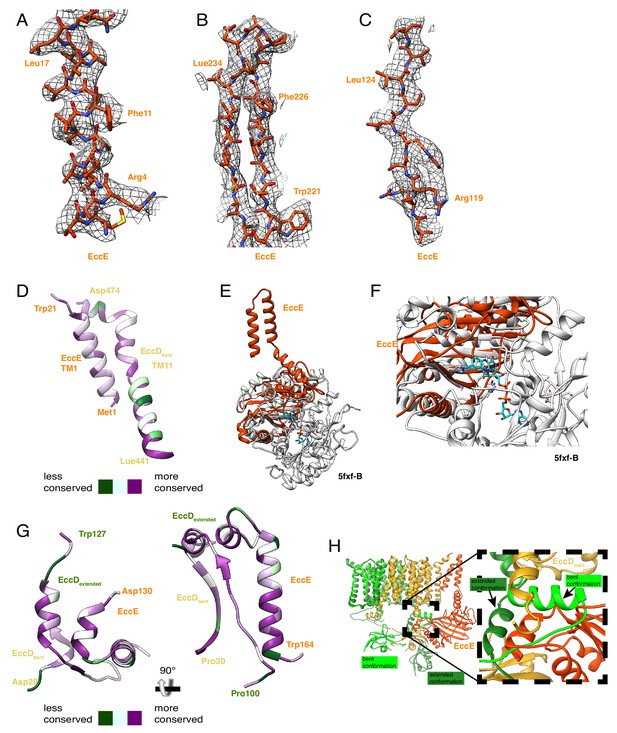
EccE3 map and model.
(A) Map and model comparison for transmembrane helix 1, amino acids 1 to 18. (B) Beta strand separation, amino acids amino acids 221 to 240. (C) A single beta strand, amino acids 117 to 126. (D) Conservation mapping onto transmembrane helix1 of EccE3 and transmembrane helix11 of EccD3-bent. (E) Comparison between the structure of EccE3 and the top DALI hit 5FXF-B. (F) Close up on the FADH (cyan) binding pocket of 5fxf-B which is not present in EccE3. (G) Conservation mapping onto the protein-protein interaction soluble helix of EccE3. (H) Residues 133–163 of EccE3 interact substantially with EccD3-bent and EccD3-extended, holding the flexible linker of EccD3-extended in the extended conformation. (H) EccE3 (orange) stabilizes the extended conformation of EccD3-extended (green) and sterically hinders it from adopting the bent conformation (neon green).
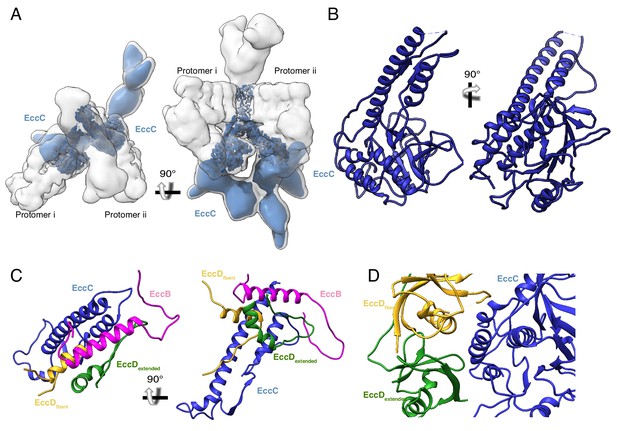
The structure and protein-protein interactions of EccC3.
(A) The placement of EccC3 in the overall ESX-3 dimer. (B) Atomic model of the EccC3 DUF (C) The stalk helices of EccC3 interact with EccB3, EccD3-bent, and EccD3-extended (D) Interactions between EccC3 and the ubiquitin-like domains of EccD3-bent and EccD3-extended in the cytoplasm.
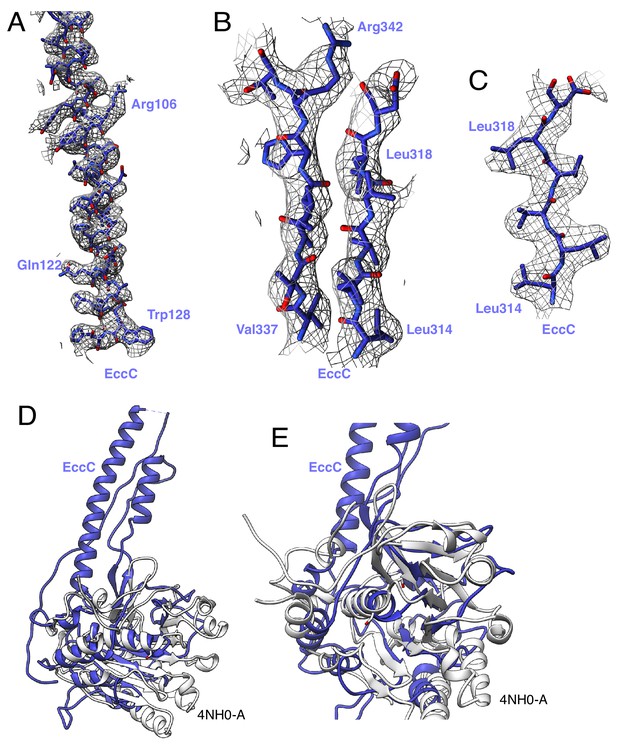
EccC3 map and model.
(A) Map and model of the EccC DUF domain, amino acids amino acids 97 to 130. (B) Two beta strands in the EccC DUF domain, amino acids 314 to 319 and 337 to 343. (C) Beta strand in the EccC DUF domain, amino acids 314 to 319. (D) Overlay of the model of the EccC DUF domain with the top DALI hit, 4NH0-A (white). (E) Close up of the ATP binding pocket of 4NH0-A. The ATPase fold remains intact in the DUF domain.
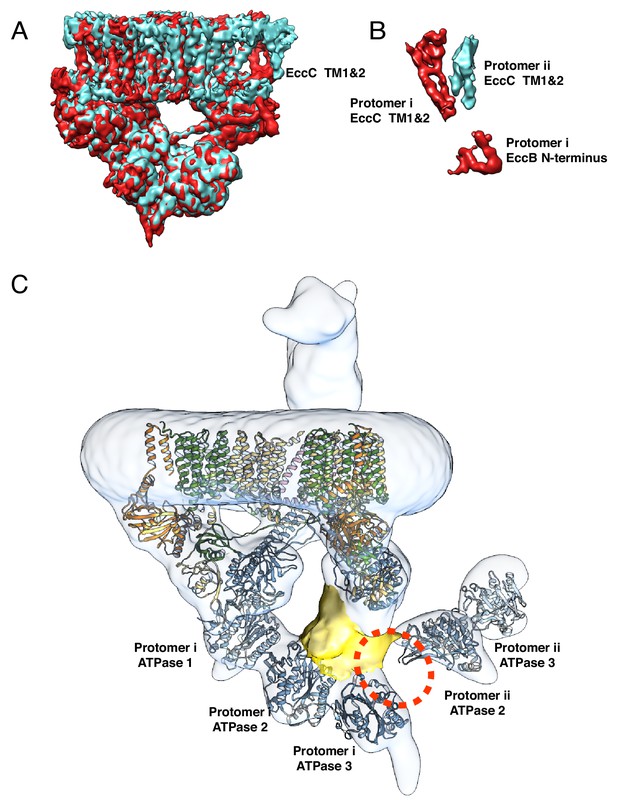
Conformational differences between protomer i and protomer ii.
(A) Overlay of the focused refined maps of the transmembrane and upper cytoplasmic regions of protomer i (red) and protomer ii (blue). The two protomers are nearly identical except in the transmembrane domains of EccC3 and the N-terminal tail of EccB3. (B) Density subtraction of the two protomers in A highlights the regions of difference. Specifically, the transmembrane helixes of EccC3 adopt distinct conformations across protomers. (C) In protomer i, 4NH0, the crystal structure of EccC from T. curvata, fits well in the density. In protomer ii, ATPase 2 and 3 fit well into the density but the position of ATPase 1 in the crystal structure, shown by the red dotted circle, does not fit into the density. A density of about the right size for ATPase 1 is shown in yellow. Fitting of ATPase 1 in this density requires the disruption of the interface between ATPase 1 and ATPase 2.
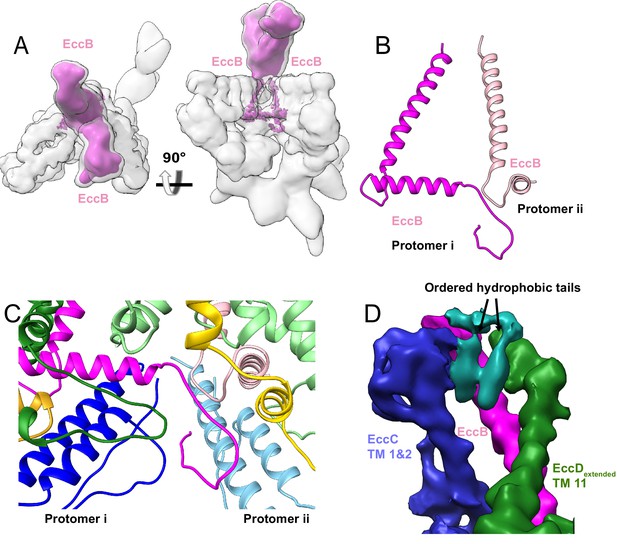
The periplasmic multimerization domain.
(A) EccB3 (pink) in the context of the overall ESX-3 dimer (gray transparency). EccB3 has a single-pass transmembrane domain which extends into a large periplasmic domain which was resolved at 5.8 Å resolution. (B) Atomic models of the EccB3 cytoplasmic and transmembrane domains, amino acids 14–93 and 32–93. (C) The N-terminus of EccB3 forms extensive cross-protomer contacts with EccC3 (blue), EccD3-bent (yellow), and EccD3-extended (green). (D) An unsharpened map of the ESX-3 dimer reveals ordered densities consistent with lipids or detergent molecules mediating the interactions between the EccB3 transmembrane helix (marked with a pink dot) and the EccC3 transmembrane helices.
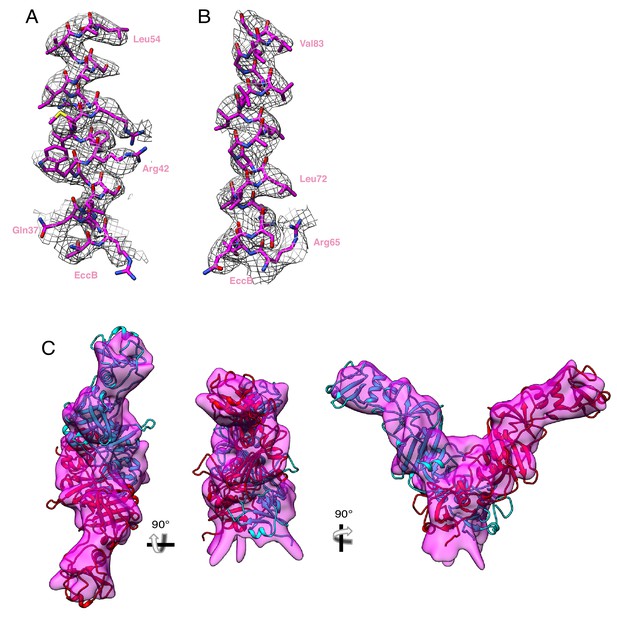
EccB3 maps and models.
(A) Fit between map and model in the linker helix, amino acids 34 to 54. (B) Fit between map and model in the transmembrane helix, amino acids 65 to 85. (C) Fit between homology models of the soluble domain of EccB3 and the periplasmic focused refinement map.
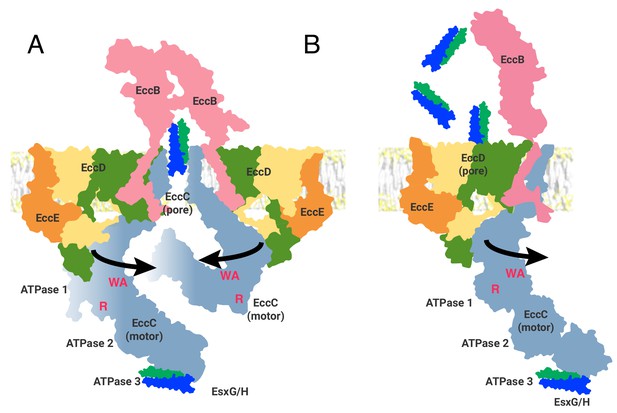
Two models of the ESX-3 translocon complex.
ATPase activity entails, at a minimum, oligomerization of ATPase 1 to bring the R-finger (R) into proximity of the catalytic site, marked by the Walker A motif (WA). This requires at least 65 Å of movement from the position seen in the structure. (A) The first model of ESX substrate secretion involves trimerization of the ESX-3 dimer followed by multimerization of the EccC ATPase domains into a stack of one to four rings of ATPases (B) The second model shown through the function of a single protomer of the ESX-3 complex. Substrates are selected by interaction with ATPase 3 of EccC and transported via the upper cytoplasmic region to the EccD cavity for secretion.
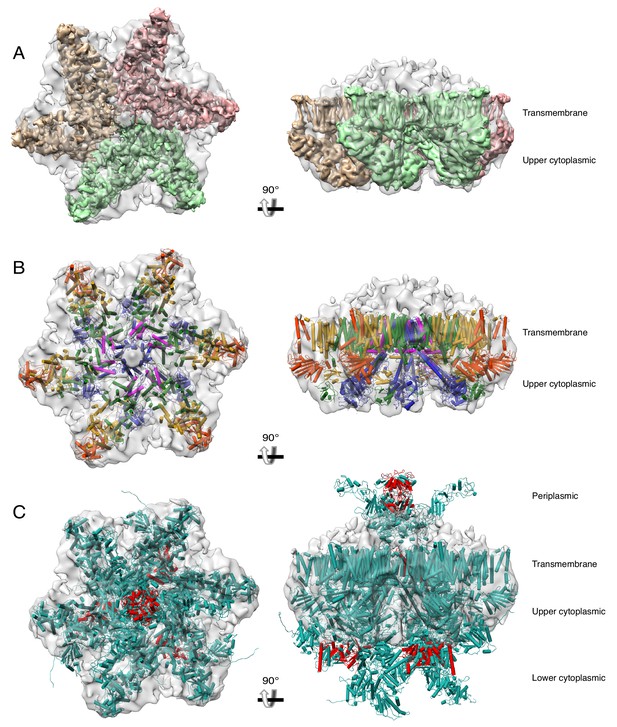
A hexameric model of the ESX-3 dimer.
(A) Fit of three copies of the transmembrane and upper cytoplasmic regions of the ESX-3 translocon complex into the ESX-5 hexameric structure (EMDB 3596). The ESX-3 translocon complex was filtered to 6 Å resolution to permit easy visualization of the EccC3 transmembrane helices. (B) The model of the ESX-3 translocon complex for the full transmembrane and upper cytoplasmic domains (including homology models of the EccC3 transmembrane helices accurate to 6 Å resolution) colored by protein. (C) The full model of the ESX-3 translocon complex including homology models for all three EccC3 ATPase domains and the periplasmic domain of EccB3 docked into the ESX-5 negative stain map. Red represents areas were atoms clash substantially.
Tables
Data collection and refinement statistics.
Collection parameters for initial test data set and void peak analysis on the Talos Arctica and final collection on Titan Krios microscopes. Refinement details for initial model, consensus map, and focused refinements.
Data Collection | ||||
---|---|---|---|---|
Collection | Initial Screening | Collection 1 | Collection 2 | Void peak |
Microscope | Talos Arctica | Titan Krios | Titan Krios | Talos Arctica |
Voltage (kV) | 200 | 300 | 300 | 200 |
Detector | Gatan K2 | Gatan K2 | Gatan K2 | Gatan K3 |
Pixel size (Å/pixel) | 1.14 | 0.82 | 0.82 | 0.9 |
Exposure Time (s) | 9 | 10 | 10 | 11.7 |
Electron dose (e-/Å2) | 63 | 80 | 67 | 58 |
Defocus range (µm) | 1.5-2.5 | 0.4-1.2 | 0.6-1.4 | 1.5-2.5 |
Number of micrographs | 2,499 | 2,705 | 4,632 | 1,215 |
Consensus Reconstruction | ||||
Data Set | Initial Screening | Collection 1 & 2 | Void peak | |
Software | Relion 2.1, cisTEM, and cryosparc | Relion 3.0 | cisTEM | |
# of particles, picked | 240,000 | 778,149 | 259,333 | |
# of particles post, Class2D | 138,000 | 554,901 | 640 | |
# of particles post, Class3D | 46,830 | 362,438 | NA | |
# of particles post, skip align Class3D | NA | 90,479 | NA | |
Symmetry | C1 | C1 | NA | |
Map sharpening B-factor (Å2) | NA | -160 | NA | |
Final resolution (Å) | 4.7 Å | 4.0 Å | NA | |
Focused Refinements | ||||
Location of focus | # of particles | Resolution | ||
Protomer i | 76,967 | 3.8 | ||
Protomer ii | 90,479 | 3.8 | ||
Symmetry expanded protomer | 52,067 | 3.7 | ||
Periplasmic EccB3 | 70,000 | 5.8 | ||
ATPase 1, 2, and 3 | 30,000 | 7 |
Reagent type (species) or resource | Designation | Source or reference | Identifiers | Additional Information |
---|---|---|---|---|
Biological sample Mycobacterium smegmatis | mc(2)155 | ATCC | 700084 | Wild type strain |
Biological sample Mycobacterium smegmatis | mc(2)155 with ideR::mγδ200 (KanR) | Dussurget et al., 1996, Provided by GM Rodriguez | ||
Biological sample Mycobacterium smegmatis | mc(2)155, MSMEG_0626-3C-EGFP | This paper | ||
Biological sample Mycobacterium smegmatis | mc(2)155 with ideR::mγδ200 (KanR), MSMEG_0626-3C-EGFP | This paper | ||
Recombinant DNA reagent | pKM444 | Murphy et al., 2018 | Addgene Plasmid #108319 | Plasmid encoding for Che9c phage RecT and Bxb1 phage Integrase |
Recombinant DNA reagent | pKM444 - zeo | This paper | Addition of zeocin resistance cassette to pKM444 plasmid | |
Recombinant DNA reagent | pKM468-3C-EGFP | This paper | Modified ORBIT tagging plasmid | |
Sequenced-based reagent | ORBIT targeting oligonucleotide | This paper | Oligo | 5’ TGTGCGTTCCACTGGTTCCCCGGCAACCACCTGCTGCACGTGAGCCAGCCGGACTACCTAGGTTTGTACCGTACACCACTGAGACCGCGGTGGTTGACCAGACAAACCCGCCGGATGACCCGCTTCCTGCGCGGCTTCATGTTCGACTGAACCCTTCACCGAGGTCCG 3’ |
Antibody | Anti-GFP stabilized antibody preparation | Roche | Cat. #: 11814460001 | Dilution 1:10000 |
Antibody | Anti-mouse IgG HRP-conjugated antibody | R&D Systems | Cat. #: HAF007 | Dilution 1:5000 |
Antibody | Goat anti-rabbit IgG antibody HRP | GenScript | Cat. #: A00098 | Dilution 1:5000 |
Antibody | Rabbit anti-GroEL | Sigma-Aldrich | Cat. #: G6532-.5ML | Dilution 1:5000 |
Peptide, recombinant protein | Human Rhinovirus (HRV) 3C Protease | Thermo Scientific Pierce | Cat. #: 88946 | |
Commercial assay or kit | Superose 6 Increase 10/300 GL | GE Healthcare | Cat. #: 29091596 | |
Chemical compound, detergent | DDM, n-Dodecyl-β-D-Maltopyranoside | Inalco | Cat. #: 1758-1350 | |
Chemical compound, detergent | GDN, glyco-diosgenin | Anatrace | Cat. #: GDN101 | |
Other | NativePAGE 3-12% Bis-Tris Protein Gels, 1.0 mm, 10-well | ThermoFisher Scientific | Cat. #: BN1001BOX | |
Commercial assay or kit | Pierce Silver Stain Kit | Pierce | Cat. #: PI24612 | |
Software, algorithm | SerialEM | Mastronarde, 2005 | https://bio3d.colorado.edu/SerialEM/ | |
Software, algorithm | MotionCor2 | Zheng et al., 2017 | http://msg.ucsf.edu/em/software/motioncor2.html | |
Software, algorithm | CTFfind4 | Rohou and Grigorieff, 2015 | https://grigoriefflab.umassmed.edu/ctffind4 | |
Software, algorithm | RELION | Scheres, 2012 | cam.ac.uk/relion/index.php/Download_%26_install | |
Software, algorithm | cisTEM | Grant et al., 2018 | https://cistem.org/ | |
Software, algorithm | cryosparc | Punjani et al., 2017 | https://cryosparc.com/docs/reference/install/ | |
Software, algorithm | pyem | Asarnow et al., 2019 | https://github.com/asarnow/pyem | |
Software, algorithm | coot | Emsley et al., 2010 | https://www2.mrc-lmb.cam. | |
Software, algorithm | raptorX | Källberg et al., 2012 | http://raptorx.uchicago.edu/ | |
Software, algorithm | phenix real space refine | Afonine et al., 2018 | https://www.phenix-online. | |
Software, algorithm | MDFF | Trabuco et al., 2009 | org/documentation/reference/refinement.html | |
Software, algorithm | namdinator | Kidmose et al., 2019 | https://namdinator.au.dk/ | |
Software, algorithm | pisa | Krissinel, 2015 | http://www.ccp4.ac.uk/pisa/ | |
Software, algorithm | chimera | Pettersen et al., 2004 | https://www.cgl.ucsf.edu/chimera/ | |
Software, algorithm | chimeraX | Goddard et al., 2018 | https://www.cgl.ucsf.edu/chimerax/ | |
Software, algorithm | DALI | Holm and Laakso, 2016 | http://ekhidna2.biocenter.helsinki.fi/dali/ |
Additional files
-
Supplementary file 1
Model Refinement Statistics.
- https://cdn.elifesciences.org/articles/52983/elife-52983-supp1-v2.docx
-
Supplementary file 2
Top Dali server hits.
- https://cdn.elifesciences.org/articles/52983/elife-52983-supp2-v2.xlsx
-
Supplementary file 3
Buried surface area.
- https://cdn.elifesciences.org/articles/52983/elife-52983-supp3-v2.docx
-
Transparent reporting form
- https://cdn.elifesciences.org/articles/52983/elife-52983-transrepform-v2.pdf