Long ascending propriospinal neurons provide flexible, context-specific control of interlimb coordination
Figures
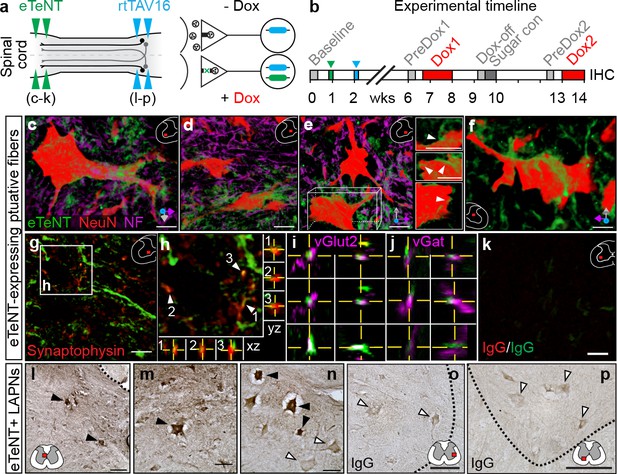
Histological detection of putatively silenced long ascending propriospinal neurons (LAPNs).
(a–b) Experimental design (see Materials and methods for details). (c–f) Volume rendered, high magnification images showing enhanced eTeNT.EGFP putative fibers (green) surrounding NeuN-stained neurons (red) and neurofilament-marked neural processes (magenta) in the cervical spinal cord (100x; x-y-z axis orientation shown in bottom right). Neuron in panel e is rotated about x-y-z axis to show eTeNT.EGFP fibers surrounding somata (inset panels right side). Neurofilament staining excluded in panel f for clarity (eTeNT.EGFP enshrouding cervical neuron). (g–h) eTeNT.EGFP signal co-localizes with synaptophysin (red). XZ-YZ orthogonal cross-sections through putative synapses shown in panel (h). eTeNT.EGFP signal co-localizes with the excitatory neurotransmitter marker vesicular glutamate transporter 2 (i, vGlut2; magenta) as well as the inhibitory neurotransmitter marker vesicular GABA transporter (j, vGat; magenta) (XZ-YZ orthogonal cross-sections shown). (k) Isotype controls revealed little-to-no immunoreactivity (IgG controls for synaptophysin and eTeNT.EGFP shown). (l–n) DAB enhancement of eTeNT.EGFP at the lumbar segments revealed dark immunoreactive neurons in the rostral lumbar segments (filled arrowheads) intermingled with DAB-negative neurons (open arrowheads). (o–p) Isotype control revealed little-to-no immunoreactivity. (c–k Scale bar = 25 µm; g,k scale bar = 10 µm; l,o,p Scale bar = 100 µm; m,n Scale bar = 50 µm).
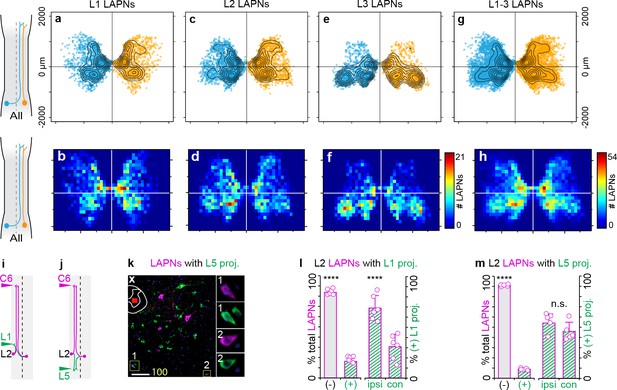
Long ascending propriospinal neurons (LAPNs) are a bilaterally distributed pathway throughout the rostral lumbar enlargement with modest local projections.
(a–i) Intrasegmental distribution profile of LAPNs. Scatter plot of (a) ipsilateral (n = 1,069) and (b) commissural-projecting LAPNs (n = 1,379) at spinal L1 with overlaid contour map (N = 11 animals; see methods for details). (c) Heatmap illustrating intrasegmental distribution of ipsilateral and commissural-projecting LAPNs at spinal L1 (n = 2448 neurons). Scatter-contour plots with corresponding heatmaps shown for spinal L2 (d-f, n = 3047 total LAPNs) and L3 (g–i, n = 2342 total LAPNs), respectively (L2: n = 1407 ipsi- and n = 1640 commissural LAPNs, N = 11 animals; L3: n = 1106 ipsi- and n = 1236 commissural LAPNs, N = 8 animals, see methods for details). (j,k) Experimental design to quantify L2 LAPNs with projections to rostral (L1) or caudal (L5) lumbar segments. (l) Representative image of spinal L2 LAPNs that are negative (left panel) and positive (right panel, insets 1–2) for resident projections in lumbar enlargement (maximum intensity projection of collapsed z-stack taken at 20x; magenta = LAPNs labeled with CTB Alexa647 (injected on the left side), green = neurons with L5 projections; scale bar = 100 µm). (m, left) The significant majority of L2 LAPNs lack local projections to spinal L1 (83.75 ± 2.88% negative vs 16.25 ± 2.88% positive; ***p<0.001; two-way analysis of variance (ANOVA) with Bonferroni post hoc). (m, right) Of the L2 LAPNs that have local projections to spinal L1, the significant majority are ipsilateral in nature (69.05 ± 12.25% ipsi- vs 30.95 ± 12.25% contralateral; ***p<0.001). (n, left) The significant majority of L2 LAPNs lack local projections to spinal L5 (91.05 ± 1.02% negative vs 8.95 ± 1.02% positive; ***p<0.001). (n, right) The few L2 LAPNs that project to spinal L5 do so with equal contributions of ipsilateral and commissural projections (54.07 ± 8.98% ipsi- vs 45.93 ± 8.98% commissural; p=0.99).
-
Figure 1—figure supplement 1—source data 1
contains the source data for CTB labeled cell body counts.
- https://cdn.elifesciences.org/articles/53565/elife-53565-fig1-figsupp1-data1-v2.xlsx
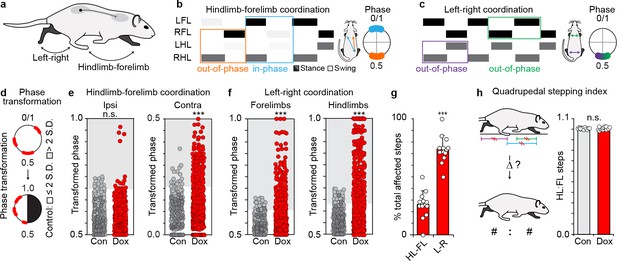
Silencing long ascending propriospinal neurons (LAPNs) disrupts intra-girdle movements during overground stepping.
(a–c) Representative swing-stance graphs of stepping behaviors observed at control time points. Left: orange = homolateral HL-FL movements (out-of-phase, 0.5), blue = diagonal HL-FL movements (in-phase, 0.0/1.0). Right: green = left -right forelimb, purple = left-right hindlimb, each out-of-phase. Insets = one complete stride cycle (right limb reference). (d) Circular 0–1 phase data are transformed into a linear scale (0.5–1.0 or 0.0–0.5). (e) Left: silencing LAPNs does not disrupt homolateral ("ipsi") HL-FL coordination (# steps beyond control variability: Control n = 19/480 [3.95%] vs Dox n = 17/600 [2.83%]; p=0.31, z = 1.01, Binomial Proportion Test; circles = individual step cycles; shaded region = values beyond control variability). Right: diagonal ("contra") HL-FL coordination is significantly disrupted (Control n = 17/480 [3.54%] vs Dox n = 98/600 [16.33%]; ***p<0.001, z = 7.47). (f) Silencing LAPNs significantly disrupts left-right forelimb and left-right hindlimb coordination, respectively (forelimbs: Control n = 26/480 [5.42%] vs Dox n = 135/600 [22.50%]; p<0.001, z = 8.57; hindlimbs: Control n = 26/480 [5.42%] vs Dox n = 177/600 [29.50%]; ***p<0.001, z = 11.31). (g) Silencing LAPNs disrupts left-right movements more than hindlimb-forelimb (% total altered steps: hindlimb-forelimb 26.20 ± 3.02% vs left-right 73.80 ± 3.37%; ***p<0.001, critical t = 2.17, paired t-test; bars = group mean± S.D.; circles=% total steps taken that are altered for individual animals). (h) The quadrupedal stepping index remained unchanged during silencing (Control: 100.78 ± 0.87 vs Dox: 100.76 ± 1.55; p=0.97, critical t = 2.17; paired t-test).
-
Figure 2—source data 1
Contains the source data for step ratio measures.
- https://cdn.elifesciences.org/articles/53565/elife-53565-fig2-data1-v2.xlsx
-
Figure 2—source data 2
Contains the source data for the magnitude of change of step ratio measures.
- https://cdn.elifesciences.org/articles/53565/elife-53565-fig2-data2-v2.xlsx
-
Figure 2—source data 3
Contains the source data for the interlimb coordination measures.
- https://cdn.elifesciences.org/articles/53565/elife-53565-fig2-data3-v2.xlsx
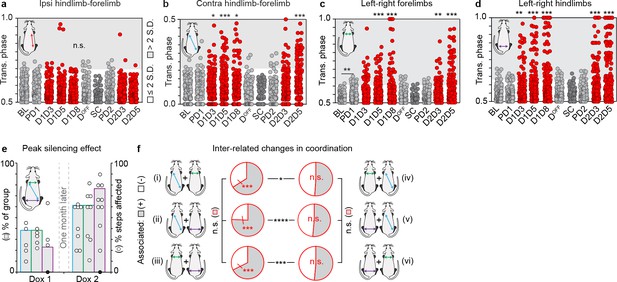
Silencing long ascending propriospinal neurons (LAPNs) disrupts interlimb coordination during overground locomotion.
(a–d) Individual time point comparisons for silencing-induced changes in interlimb coordination. Time points: BL = Baseline, PD1 = Pre-Dox1, D1D3 = Dox1 On-Day 3, D1D5 = Dox1 On-Day 5, D1D8 = Dox1 On-Day 8, DOFF = DoxOff, SC = sugar water (vehicle) control, PD2 = PreDox2, D2D3 = Dox2 On-Day 3, D2D5 = Dox2 On-Day 5. (a) Ipsilateral hindlimb-forelimb was not affected during LAPN silencing. (b) Silencing LAPNs significantly disrupted contralateral hindlimb-forelimb coordination as seen by the increase in the proportion of steps that deviated beyond normal control variability associated with overground walking (Pre-Dox1 [PD1, n = 5/120 step cycles] vs Dox1On-D3/5/8: *p<0.05 [n = 14/120], ***p<0.001 [n = 20/120], *p<0.05 [n = 15/120], Binomial Proportion Test). Re-silencing one month later reproduced the effect (Pre-Dox2 [n = 7/120] vs D2D5 [n = 35/120]: ***p<0.001). (c) Silencing LAPNs significantly disrupted left-right forelimb alternation (PD1 [n = 10/120] vs D1D5 [n = 29/120], ***p<0.001; vs D1D8 [n = 24/120], **p<0.01). Re-silencing one month later reproduced the effect (PD2 [n = 7/120] vs D2D3 [n = 23/120], **p<0.01; vs D2D5 [n = 41/120], ***p<0.001). (d) Silencing LAPNs significantly disrupted left-right hindlimb alternation (PD1 [n = 9/120] vs D1D3 [n = 26/120], **p<0.01; vs D1D5 [n = 29/120]/D1D8 [n = 35/120], both ***p<0.001). Re-silencing one month later reproduced the effect (PD2 [n = 6/120] vs D2D3 [n = 34/120]/D2D5 [n = 53/120], each ***p<0.001). Apart from the left-right forelimbs at Baseline vs Pre-Dox1 (n = 1/120 vs n = 10/120 steps), no significant differences were detected between control time points across all limb pairs analyzed. The vehicle control (sucrose water; "sugar control, SC") did not affect interlimb coordination. Group data shown (N = 13). Individual circles = individual steps (n = 84/time point, except sugar control where n = 50 steps). Circles plotted in shaded region denote steps with coordination values that deviated beyond control variability. (e) More animals showed peak disruption to interlimb coordination during Dox2 silencing vs Dox1. Bars denote % group that showed peak disruption to stepping at Dox1 vs Dox2. Circles denote peak % steps affected for each animal. Blue = contralateral hindlimb-forelimb, green = left -right forelimb, purple = left-right hindlimb coordination. Filled circle denotes an animal that showed no changes to hindlimb coordination during silencing. (f) Plots illustrating inter-related changes in coordination during LAPN silencing. The majority of locomotor bouts (one complete step cycle for all four limbs) with affected contralateral hindlimb-forelimb coordination were concomitant with changes in left-right coordination (panel i, gray denotes positive association, n = 68/103 steps [66.02%] vs white denoting no association 35/103 steps [33.98%], ***p<0.001 shown in red; z = 4.85). The reciprocal comparison (changes in left-right forelimb coordination are concomitant with changes in contralateral hindlimb-forelimb) did not show this relationship (panel iv, gray: n = 68/133 steps showing positive association vs white, n = 65/133 steps showing no association; p>0.5; z = 0.37). Similar results were found when comparing the inter-relationship between affected contralateral hindlimb-forelimb coordination and affected left-right hindlimb coordination (panel ii; gray: n = 78/103 steps showing positive association vs white, n = 23/103 steps showing no association; ***p<0.001 shown in red; z = 8.61). Once again, the reciprocal comparison showed no association (panel v; gray: n = 90/175 steps showing positive association vs white, n = 85/175 steps showing no association; p>0.5; z = 0.53). The majority of affected forelimb steps were concomitant with altered hindlimb steps (panel iii, gray: n = 90/133 steps showing positive association vs white, n = 43/133 steps showing no association; ***p<0.001 shown in red; z = 6.16). The reciprocal comparison did not show this association (panel vi, gray: n = 90/175 steps showing positive association vs white, n = 85/175 steps showing no association; p>0.5; z = 0.53).
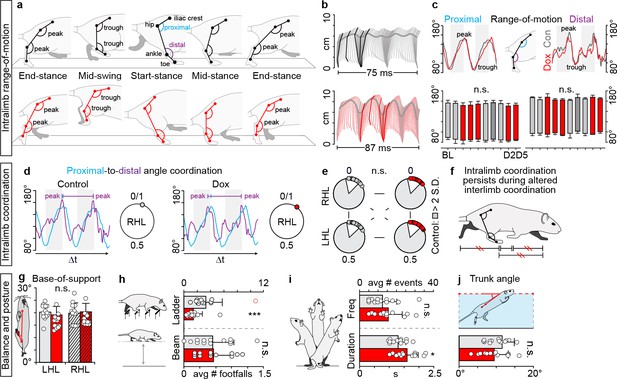
Intralimb coordination and postural control endures despite silencing-induced generalized interlimb discoordination.
(a) Three-segment (iliac crest-hip, hip-ankle, ankle-toe), two-angle model of intralimb coordination. Five phases of step cycle illustrated with corresponding hindlimb range-of-motion (peak-to-trough excursion of the proximal and distal angles) and intralimb kinematics (b). (c) Range-of-motion was not altered during silencing (right hindlimb shown, group average ± S.D. [Baseline to Dox2On-D5]; p>0.5, mixed model ANOVA, Bonferroni post hoc). (d) Representative example of proximal-to-distal temporal coordination for one stride cycle (temporal overlap in peak angular excursions). Intralimb coordination plotted on circular graph where 0 denotes in-phase coordination. (e) Silencing long ascending propriospinal neurons (LAPNs) did not disrupt the proximal-to-distal temporal relationship across the hindlimb segments (p>0.5 for all comparisons; Watson’s U (Orlovskiĭ et al., 1999) test). White inset = control variability. Individual circles = peak to-peak proximal or distal excursion for one stride cycle. (f) Summary schematic. (g) Silencing LAPNs did not affect hindlimb base-of-support during overground stepping (Baseline vs Dox1On-D5: left hindlimb, 20.23 ± 3.00° [n = 220 steps] vs 19.13 ± 3.38° [n = 223 steps], p=0.31; right hindlimb, 19.76 ± 4.19° [n = 227 steps] vs 20.37 ± 3.39° [n = 229 steps], p=0.62; paired t-tests). (h) The number of hindlimb foot falls on the ladder significantly decreased during silencing versus control (Control 3.33 ± 2.47 vs DoxOn1.43 ± 1.33, **p<0.01; excluding outlier [red circle] yielded similar results – see Materials and mmethods for details). No significant differences were detected on the beam (Control 0.55 ± 0.38 vs Dox 0.55 ± 0.32, p=0.96). (i) Frequency of spontaneously evoked rearing events remained unchanged during silencing (Control 7.62 ± 4.89 vs Dox 9.46 ± 4.74, p=0.29). There was a slight, but significant increase in the duration of the rearing events during silencing (Control 1.56 ± 0.31 s vs Dox 1.92 ± 0.47 s, p=0.045). (j) Trunk angle during swimming remained unchanged during silencing (Control 10.23 ± 2.87° vs Dox 9.49 ± 3.78°, p=0.54). Data shown from N = 13 animals. Circles = individual averages; bars = group average± S.D.
-
Figure 3—source data 1
Contains the source data for trunk angle measures.
- https://cdn.elifesciences.org/articles/53565/elife-53565-fig3-data1-v2.xlsx
-
Figure 3—source data 2
Contains the source data for intralimb range-of-motion.
- https://cdn.elifesciences.org/articles/53565/elife-53565-fig3-data2-v2.xlsx
-
Figure 3—source data 3
Contains the source data for intralimb coordination measures.
- https://cdn.elifesciences.org/articles/53565/elife-53565-fig3-data3-v2.xlsx
-
Figure 3—source data 4
Contains the source data for foot faults on the narrow beam.
- https://cdn.elifesciences.org/articles/53565/elife-53565-fig3-data4-v2.xlsx
-
Figure 3—source data 5
Contains the source data for hindlimb base-of-support.
- https://cdn.elifesciences.org/articles/53565/elife-53565-fig3-data5-v2.xlsx
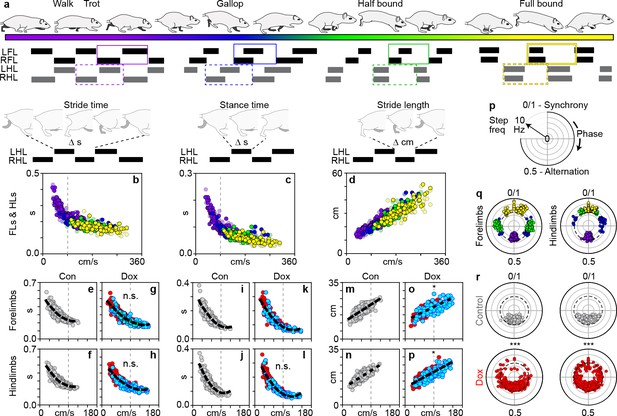
Silencing long ascending propriospinal neurons (LAPNs) disrupts interlimb coordination independent from the salient features of locomotion.
(a) Schematic illustrating the speed-dependent gaits with representative swing-stance graphs (purple = walk trot; blue = gallop; green = half-bound; yellow = full-bound). (b) Schematic illustrating stride time (duration of one stride) and its normal relationship with speed for the volitionally expressed gaits (N = 12 age-matched controls, see methods; circles = individual steps). This relationship persisted during silencing (forelimbs: c,d, Control: p<0.001, rS = −0.845, R2 = 0.714 [n = 480 steps] vs Dox: p<0.001, rS = −0.889, R2 = 0.790 [n = 600]) (hindlimbs: e,f, Control: p<0.001, rS = −0.864, R2 = 0.746 vs Dox: p<0.001, rS = −0.908, R2 = 0.824; steps with silencing-induced altered coordination shown in blue for clarity; altered step defined as step cycle with a phase relationship that deviates beyond control variability; dashed line = line of best fit). (g) Schematic illustrating stance time (duration of paw contact for one stride) and its normal relationship with speed. This relationship persisted during silencing (h,i, forelimbs, Control: p<0.001, rS = −0.905, R2 = 0.819 vs Dox: p<0.001, rS = −0.929, R2 = 0.863) (j,k, hindlimbs, Control: p<0.001, rS = −0.901, R2 = 0.812 vs Dox: p<0.001, rS = −0.946, R2 = 0.895). (l) Schematic illustrating stride length (distance traveled for one stride) and its normal relationship with speed. This relationship persisted during silencing (m,n, forelimbs, Control: p<0.001, rS = 0.784, R2 = 0.615 vs Dox: p<0.001, rS = 0.736, R2 = 0.582; o,p, hindlimbs, Control: p<0.001, rS = 0.801, R2 = 0.642 vs Dox: p<0.001, rS = 0.787, R2 = 0.619). There was a slight change in the slopes for the lines of best fit for stride length versus speed during silencing (n, *p<0.05; t = 2.18; p, *p<0.05, t = 2.42). (q) Phase-frequency plot illustrating phase change as a function of frequency. (r) Left-right forelimb and left-right hindlimb phase-frequency relationships for the speed-dependent gaits (dashed circle = 5 Hz transition zone from the walk-trot to gallop Gillis and Biewener, 2001; Muir and Whishaw, 2000). Silencing LAPNs functionally decoupled the left-right fore- and hindlimbs, respectively (s, left: forelimbs, Control vs Dox, ***p<0.001, U2 = 0.67, n1 = 123, n2 = 187, Watson’s U (Orlovskiĭ et al., 1999) test; (s), right: hindlimbs: Control vs Dox, ***p<0.001, U2 = 1.45, n1 = 131, n2 = 204; refer to Supplementary file 2; white inset denotes control variability, circles denote individual step cycles). The decoupled limb pairs stepped at Control-level frequencies (forelimbs, Control: 99.80% [n = 479/480] at ≤5 Hz; Dox: 92.50% [n = 555/600]; hindlimbs, Control: 100% [n = 480/480] at ≤5 Hz; Dox: 95.30% [n = 572/600]).
-
Figure 4—source data 1
Contains the source data for stereotypical gait measures.
- https://cdn.elifesciences.org/articles/53565/elife-53565-fig4-data1-v2.xlsx
-
Figure 4—source data 2
Contains the source data for phase/frequency relationship.
- https://cdn.elifesciences.org/articles/53565/elife-53565-fig4-data2-v2.xlsx
-
Figure 4—source data 3
Contains the source data for spatiotemporal relationship.
- https://cdn.elifesciences.org/articles/53565/elife-53565-fig4-data3-v2.xlsx
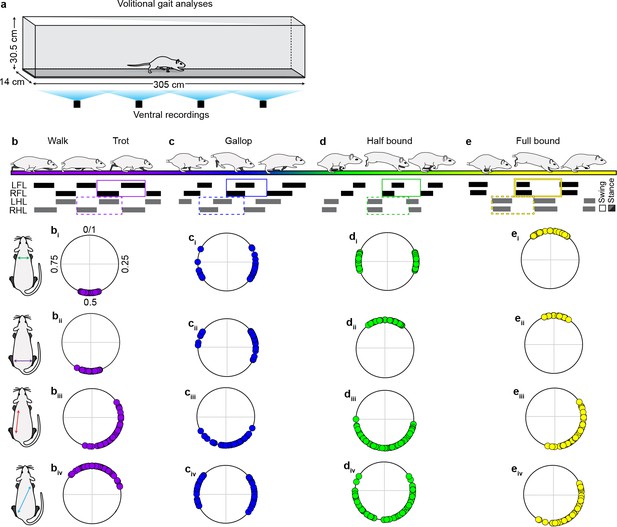
Stereotypic limb coupling patterns for speed-dependent, volitionally expressed locomotor gaits.
(a) Schematic illustrating custom-built long tank to analyze volitionally-expressed locomotor gaits (see methods for details). (b–e) Schematics and representative swing-stance graphs illustrating stereotypic locomotor gaits (purple = walk trot, blue = gallop, green = half -bound, yellow = full-bound). The corresponding polar plots denote the observed coupling patterns expressed for the various limb pairs across each gait (0/1 = synchrony; 0.5 = alternation) (walk-trot: n = 160 step cycles; gallop: n = 50 step cycles; half-bound: n = 108 step cycles; full-bound: n = 80 step cycles). Fewer gallop step cycles were analyzed due to the transient nature of this gait. Data collected from N = 12 age-matched controls across six time points (see Materials and mmethods for detail). Gaits were defined based on previously described criteria (Pocratsky et al., 2017). Source file: "Figure 4—source data 1."
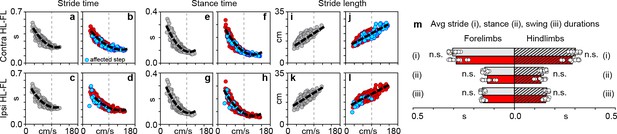
Salient features of locomotion remain unaffected during silencing-induced disruption to interlimb coordination.
(a–l) The fundamental relationship between speed and various speed-dependent spatiotemporal features of locomotion remained intact during silencing-induced changes to hindlimb-forelimb coordination (Control: n = 480 steps; Dox: n = 600 steps; blue circles = steps where interlimb coordination was significantly disrupted; dashed black line = line of best fit; dashed gray line = speed transition zone from walk-trot to gallop; Gillis and Biewener, 2001; Muir and Whishaw, 2000). (m) No differences were detected when comparing the average stride (i), stance (ii), and swing (iii) durations for Control vs Dox within and between the fore- and hindlimbs, respectively (multivariate ANOVA with speed as a co-variate followed by Sidák post hoc t-tests where appropriate). Group data are shown (N = 13). Bars = group average ± S.D. Circles = individual values.
-
Figure 4—figure supplement 2—source data 1
Contains the source data for FL-HL spatiotemporal measures.
- https://cdn.elifesciences.org/articles/53565/elife-53565-fig4-figsupp2-data1-v2.xlsx
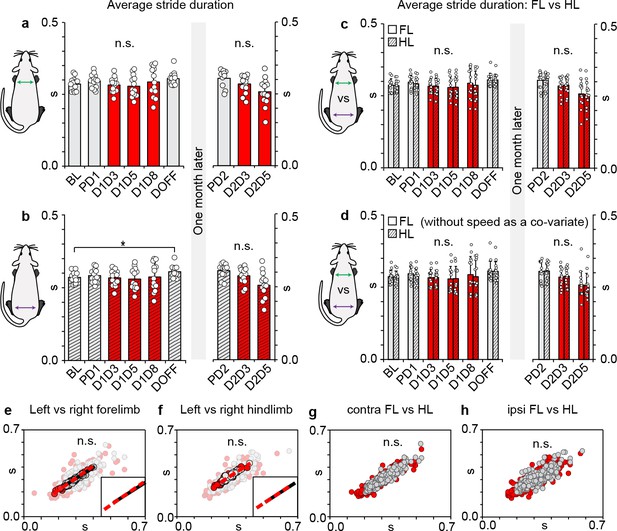
Rhythmic locomotor output within and between limb girdles remains coupled during long ascending propriospinal neuron (LAPN) silencing.
(a) Silencing LAPNs does not affect the underlying stride duration of the forelimbs (p>0.05; repeated measures ANOVA with speed as a co-variate followed by Sidák post hoc t-tests where appropriate) during overground locomotion. (b) A slight increase in the overall stride duration was detected in the hindlimbs when comparing Baseline to DoxOff nearly 6 weeks later (0.271 ± 0.004 s vs 0.298 ± 0.006 s, *p<0.05). (c,d) No differences were detected when comparing forelimb vs hindlimb stride durations within (e.g. comparing average stride duration of left versus right forelimb at Dox1On-D5) and between time points (e.g. comparing average stride duration of right hindlimb at Baseline vs Dox1On-D5) with or without controlling for the effects of speed (p>0.05; multivariate ANOVA with or without speed as a co-variate followed by Sidák post hoc t-tests where appropriate). Despite the disruption to intra- and inter-girdle temporal coordination, no differences in per-step stride durations were detected when comparing between left-right forelimbs (e), left-right hindlimbs (f), contralateral forelimb-hindlimbs (g), and ipsilateral hindlimb-forelimbs (h) for Control (n = 480 step cycles) vs Dox time points (n = 600 step cycles) (e–f, averaged datasets with lines of best fit for control [black] and DoxOn [red, dashed] overlaid onto raw; inset denotes similar slopes for lines of best fit for Control and DoxOn; g,h, averaged data excluded for clarity to demonstrate overall variability observed in raw datasets [circles = individual step cycles]; multivariate ANOVAs followed by Sidák post hoc t-tests performed on averaged datasets). Data shown in a-d are group means ± S.D. with overlaid circles denoting individual animal means.
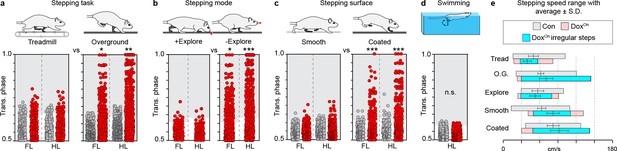
Silencing-induced disruption to interlimb coordination occurs in a task-specific, context-driven manner.
(a) Intra-girdle left-right coordination was affected to a greater extent during overground stepping as compared to treadmill during long ascending propriospinal neuron (LAPN) silencing (forelimbs, overground n = 135/600 [22.50%] vs treadmill n = 22/151 [17.05%], *p<0.05 [z = 2.38]; hindlimbs, overground n = 177/600 [29.50%] vs treadmill n = 28/151 [22.76%], **p<0.01 [z = 2.99]; Figure 5—figure supplement 1a–f; Supplementary file 3). (b) Silencing LAPNs does not affect interlimb coordination during exploratory-like stepping as compared to a more "directed" stepping mode ("going from A to B") (DoxOn forelimbs, non-exploratory overground n = 135/600 [22.50%] vs exploratory overground n = 13/95 [13.68%], *p<0.05 [z = 2.25]; DoxOn hindlimbs, non-exploratory overground n = 177/600 [29.50%] vs exploratory overground n = 7/95 [7.37%], **p<0.001 [z = 6.78]; Figure 5—figure supplement 1g–i; Supplementary file 3) (see Materials and methods for details). Silencing LAPNs does not affect intra-girdle left-right coordination while stepping on an uncoated plexiglass surface as compared to a Sylgard-coated base (N = 8 animals from a separate set of experiments; see methods for details; forelimbs, uncoated plexiglass n = 11/166 [6.63%] vs Sylgard-coated n = 39/170 [22.94%], ***p<0.001 [z = 4.34]; hindlimbs, uncoated plexiglass n = 12/166 [7.23%] vs Sylgard-coated n = 60/170 [35.29%], ***p<0.001 [z = 2.99]; Figure 5—figure supplement 1j–o; Supplementary file 3). (d) Silencing LAPNs did not affect left-right hindlimb alternation during swimming (n = 2/390 and 0/390 stroke cycles at Control and Dox, respectively; deviated beyond control variability; p>0.5 [z = 1.0]; Figure 5—figure supplement 1p; Supplementary file 3). Data shown in a,b, and d are from N = 13 animals. Data shown in c are from separate set of N = 8 animals. Circles = individual step or stroke cycles. Shaded region denotes variability beyond that observed at control time points for each condition described.
-
Figure 5—source data 1
Contains the source data for phase during exploratory walking.
- https://cdn.elifesciences.org/articles/53565/elife-53565-fig5-data1-v2.xlsx
-
Figure 5—source data 2
Contains the source data for phase during treadmill walking.
- https://cdn.elifesciences.org/articles/53565/elife-53565-fig5-data2-v2.xlsx
-
Figure 5—source data 3
Contains the source data for phase on different surfaces.
- https://cdn.elifesciences.org/articles/53565/elife-53565-fig5-data3-v2.xlsx
-
Figure 5—source data 4
Contains the source data for phase during swimming.
- https://cdn.elifesciences.org/articles/53565/elife-53565-fig5-data4-v2.xlsx
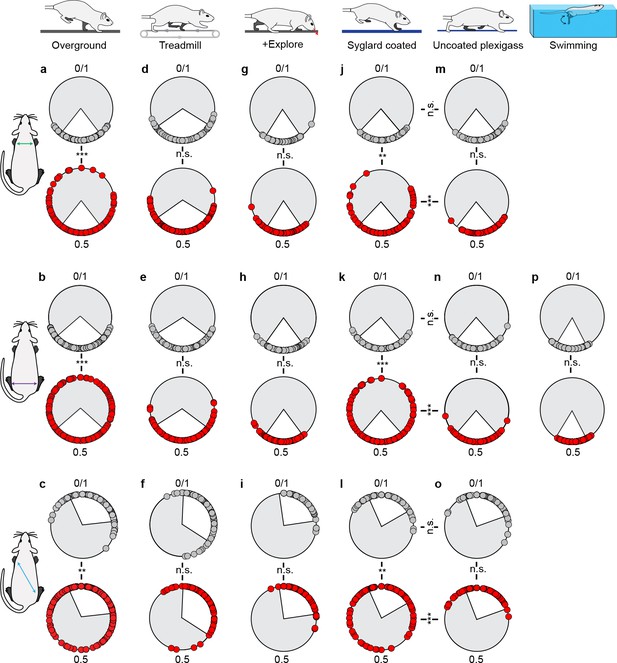
Interlimb coordination is disrupted in a context-driven manner.
Left-right forelimb (a,d,g,j,m), left-right hindlimb (b,e,h,k,n,p), and diagonal hindlimb-forelimb coordination (c,f,i,l,o) were assessed across the various behavioral contexts. Left-right forelimb and contralateral hindlimb-forelimb coordination analyses were excluded for the swim assessment as this task is primarily hindlimb-driven. Statistics are reported in Supplementary file 3. White inset denotes control variability. Circles denote individual step cycles. **p<0.01, ***p<0.001 (Watsons U2 test).
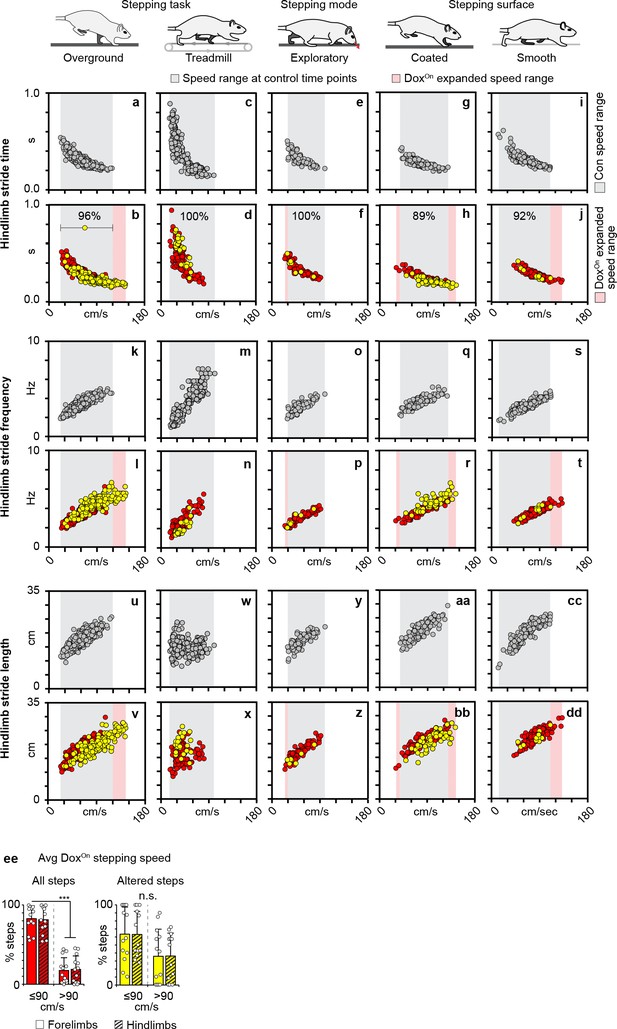
Silencing-induced changes to overground stepping occurred at a speed range which is shared across all behavioral contexts.
Hindlimb stride time vs speed (a–j), stride frequency vs speed (k–t), and stride length vs speed (u–dd) relationships observed at Control and DoxOn long ascending propriospinal neuron (LAPN) silencing across the various behavioral contexts. Circles = individual step cycles at control (gray) and DoxOn (red), yellow circles = irregular coordination observed during DoxOn, gray inset = speed range observed at control time points, pink inset = expanded speed range observed during DoxOn LAPN silencing. Preponderance of silencing-induced changes to hindlimb coordination fell within speed range observed at control time points (percentages shown in b), (d, f, h, j). Data shown in overground, treadmill, and exploratory stepping from N = 13 animals. Overground stepping: Control, n = 400 total steps at Baseline, Pre-Dox1, DoxOff, (Pre-Dox2) and DoxOn, n = 600 total steps at Dox1On-Day 3, D5, D8 and Dox2On-Day 3 and D 5. Treadmill stepping: Control, n = 277 total steps at Baseline, Pre-Dox1, DoxOff, and Pre-Dox2 and DoxOn, n = 150 total steps at Dox1On-Day four and Dox2On-Day 4. Volitional exploratory stepping: Control, n = 100 total steps at Baseline, Pre-Dox1, DoxOff, and Pre-Dox2 and DoxOn, n = 95 total steps at Dox1On-Day 3, D5, D8 and Dox2On-Day 3 and D 5. Stepping surface data generated from a separate set of N = 8 animals. Control, n = 251 and 240 total steps at Baseline, Pre-Dox1 and DoxOff for acrylic and Sylgard-coated stepping chambers, respectively. DoxOn, n = 166 and 170 total steps at Dox1On-Day five and D8 for smooth and coated stepping chambers, respectively. (ee) Instantaneous per-hindlimb step speed ranges observed across the various behavioral contexts at Control (gray) and DoxOn LAPN silencing (red). Yellow denotes speed range observed for the silencing-induced changes to hindlimb coordination. Group average and standard deviations overlaid onto bar plots. (ff, left panel) The significant majority of steps taken during silencing were ≤90 cm/s for forelimbs (solid bars; 82.70 ± 16.18% vs 17.30 ± 16.18%; ***p<0.001, paired t-test) and hindlimbs (striped bars; 81.30 ± 16.97% vs 18.70 ± 16.97%; ***p<0.001), respectively. (ff, right panel) The silencing-induced changes to interlimb coordination were not speed-dependent (percent of affected steps that occurred at ≤90 cm/s vs >90 cm/s: forelimbs, 63.90 ± 34.02% vs 36.10 ± 34.02%; p=0.17, paired t-test; hindlimbs, 63.59 ± 28.87% vs 36.41 ± 28.87%; p=0.12). Group data shown (N = 13). Bars = group average± S.D. Circles = individual values.
Videos
Conditionally silencing long ascending propriospinal neurons (LAPNs) disrupts interlimb coordination during overground stepping.
DoxOn videos shown from two independent experiments, three separate animals at Dox1On Day 8 of LAPN silencing. Videos shown from the same animal at 1x, 0.5x, and 0.25x speed.
Silencing long ascending propriospinal neurons (LAPNs) disrupts interlimb coordination during overground stepping but not during treadmill-based locomotion.
Videos shown from the same animal at 1x, 0.5x, and 0.25x speed during overground and treadmill stepping at Dox1OnDays 4 and 5.
Interlimb coordination is not affected during exploratory-like stepping behavior.
Videos shown from the same animal at the same DoxOn time point at 1x, 0.5x, and 0.25x speed.
Silencing long ascending propriospinal neurons (LAPNs) selectively disrupts interlimb coordination when animals are locomoting on a coated, but not smooth stepping surface.
Videos shown from the same animal at the Control and DoxOn time points at 1x and 0.5x speed.
Silencing long ascending propriospinal neurons (LAPNs) does not disrupt left-right hindlimb alternation during swimming.
Videos shown from the same animal at 1x, 0.5x, and 0.25x speed.
Tables
Reagent type (species) or resource | Designation | Source or reference | Identifiers | Additional information |
---|---|---|---|---|
Strain, strain background (female Sprague-Dawley rats) | Envigo | 200–220 g, approximately 10–12 weeks old | ||
Antibody (GFP) | Rabbit IgG | Abcam ab290 | 1:5000 | |
Antibody (NeuN) | Guinea pig IgG | Millipore ABN90P | 1:500 | |
Antibody (NeuN) | Mouse IgM | Millipore MAB377 | 1:500 | |
Antibody (neurofilament) | Mouse IgM | Sigma N5264 | 1:30,000 | |
Antibody (synaptophysin) | Mouse IgM | Millipore MAB5258-50UG | 1:10,000 | |
Antibody (vesicular glutamate transporter 2) | Guinea pig IgG | Millipore AB2251-I | 1:5000 | |
Antibody (vesicular GABA transporter) | Goat igG | Frontier Institute VGAT-Go-Af620 | 1:500 | |
Antibody (non-immune sera) | Rabbit IgG | Jackson ImmunoResearch #711-005-152 | 1:5000 | |
Antibody (secondary AlexaFluor 488) | Rabbit IgG | Jackson ImmunoResearch # 711-545-152 | 1:200 | |
Antibody (secondary AlexaFluor 594) | Guinea pig IgG | Jackson ImmunoResearch #706-585-148 | 1:200 | |
Antibody (secondary AlexaFluor 594) | Mouse IgG | Jackson ImmunoResearch # 715-585-150 | 1:200 | |
Antibody (secondary AlexaFluor 647) | Mouse IgG | Jackson ImmunoResearch # 715-605-151 | 1:200 | |
Antibody (secondary AlexaFluor 647) | Guinea pig IgG | Jackson ImmunoResearch # 706-546-148 | 1:200 | |
Antibody (secondary AlexaFluor 647) | Goat IgG | Jackson ImmunoResearch # 705-605-147 | 1:200 | |
HiRet-TRE-EGFP.eTeNT | Generous gift from Tadashi Isa | 1.6 × 107 vp/ml | ||
AAV2-CMV-rtTAV16 | Generous gift from Tadashi Isa | 4.8 × 1012 vp/ml | ||
HiRet-Cre | Generous gift from Zhigang He | 1.6 × 1012 vp/ml | ||
AAV2-CAG-FLEx-GFP | UNC Vector Core | 3.5 × 1012 vp/ml | ||
Chemical compound (Sylgard) | Sylgard-coated surface | Sylgard 184 Silicone Elastomer Kit, Dow Corning | ||
Chemical compound (cholera toxin B subunit conjugate) | CTB-488 | Invitrogen/Molecular Probes C-34775 | 1.5% solution in sterile saline | |
Chemical compound (cholera toxin B subunit conjugate) | CTB-594 | Invitrogen/Molecular Probes C-34777 | 1.5% solution in sterile saline | |
Chemical compound (cholera toxin B subunit conjugate) | CTB-647 | Invitrogen/Molecular Probes C-34778 | 1.5% solution in sterile saline |
Additional files
-
Supplementary file 1
The fundamental relationship between speed and the various speed-dependent locomotor parameters remains intact despite the silencing-induced disruption to interlimb coordination.
Time point comparisons reveal that silencing long ascending propriospinal neurons (LAPNs) did not affect the underlying foundation relationship between speed and stance duration, stride duration, and stride length at the fore- and hindlimbs, respectively. Running the same comparisons on the curated dataset of Dox-induced affected stepping yielded similar results (‘Dox-induced affected step’). Pearson correlation coefficients (r) and p values shown post-Bonferroni correction for multiple comparisons.
- https://cdn.elifesciences.org/articles/53565/elife-53565-supp1-v2.docx
-
Supplementary file 2
Silencing long ascending propriospinal neurons (LAPNs) functionally uncouples the intra-girdle left-right limb pairs during overground locomotion.
Interlimb coordination data were analyzed using Watson’s non-parametric two-sample U (Orlovskiĭ et al., 1999) test (Critical value of Watson’s U2 = 0.1869; Appendix D, TableD.44) (Zar, 1974)
- https://cdn.elifesciences.org/articles/53565/elife-53565-supp2-v2.docx
-
Supplementary file 3
Silencing long ascending propriospinal neurons (LAPNs) disrupts interlimb coordination in select behavioral contexts.
Interlimb coordination data were analyzed using Watson’s non-parametric two-sample U (Orlovskiĭ et al., 1999) test (Critical value of Watson’s U2 = 0.1869; Appendix D, TableD.44) (Zar, 1974)
- https://cdn.elifesciences.org/articles/53565/elife-53565-supp3-v2.docx
-
Transparent reporting form
- https://cdn.elifesciences.org/articles/53565/elife-53565-transrepform-v2.docx