Human RPA activates BLM’s bidirectional DNA unwinding from a nick
Figures
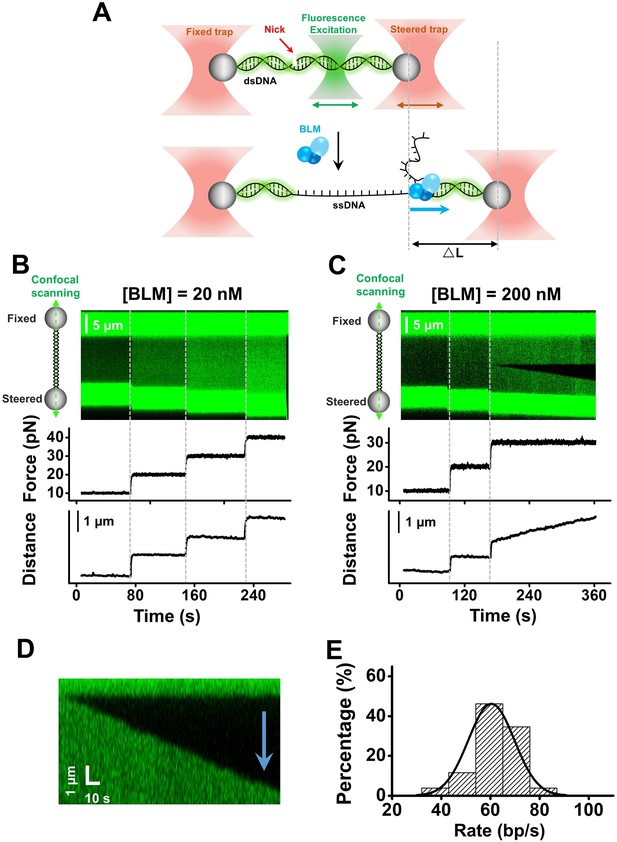
BLM unwinds dsDNA unidirectionally from a nick.
(A) A Schematic of the experimental configuration. Biotinylated λ phage DNA was suspended by two streptavidin coated beads manipulated by two optical traps. Meanwhile, confocal lasers repeatedly scanned along the DNA template. DNA length was expected to increase under a constant force when dsDNA was converted to ssDNA by BLM. (B, C) A representative kymograph of a tethered λ DNA as well as its corresponding force and DNA length as a function of time in the presence of 20 nM (B) or 200 nM (C) BLM. (D) Kymograph of an individual unwinding event showing the unidirectional DNA unwinding. The arrow indicates the direction of the unwinding fork movement. (E) Distributions of the dsDNA unwinding rates in the presence of 200 nM BLM under 30 pN.
-
Figure 1—source data 1
The dsDNA unwinding rates of 200 nM BLM under 30 pN.
- https://cdn.elifesciences.org/articles/54098/elife-54098-fig1-data1-v2.xlsx
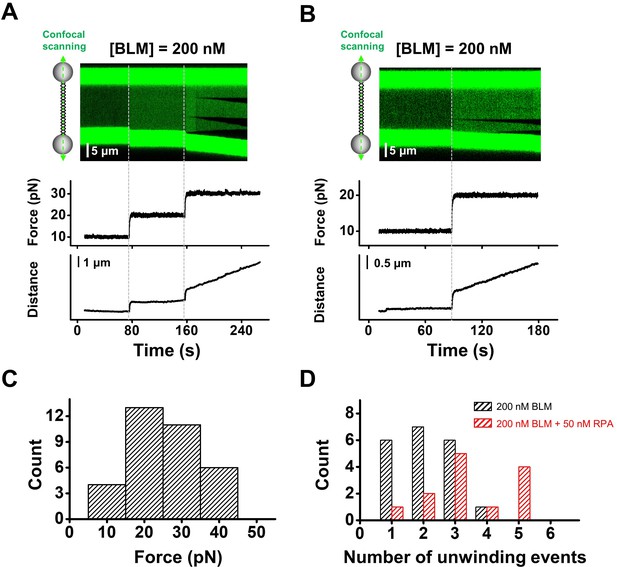
DNA unwinding in the presence of 200 nM BLM.
(A, B) Representative traces of BLM unwinding λ DNA in the presence of 200 nM BLM. Three individual unwinding events were detected once the force was increased to 30 pN in A, and two were monitored under 20 pN in B. (C) The forces required to monitor DNA unwinding by BLM range from 10 pN to 40 pN. (D) Number of unwinding events on a single λ DNA with or without RPA.
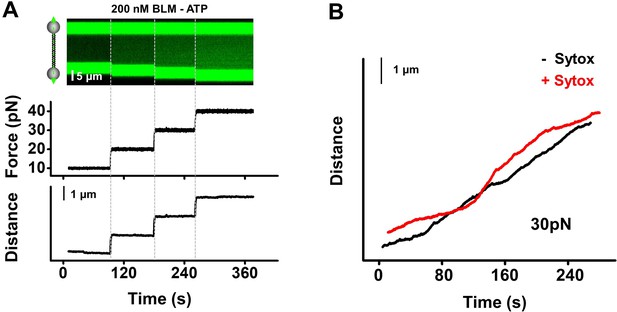
Control experiments verified BLM’s unwinding activity.
(A) To confirm that the observed unwinding was a result of BLM’s helicase activity, we conducted the unwinding experiment in the absence of ATP. In the presence of 200 nM BLM, we started the experiment at 10 pN force for ~1 min and increased it by 10 pN each time. There was no change of either fluorescent or DNA length signal under 10–40 pN (n = 13), indicating that the unwinding signals we observed were indeed due to BLM’s helicase activity. (B) BLM-mediated DNA length increases were comparable in the presence and absence of Sytox, indicating that the fluorescence dye does not affect BLM’s unwinding activity.
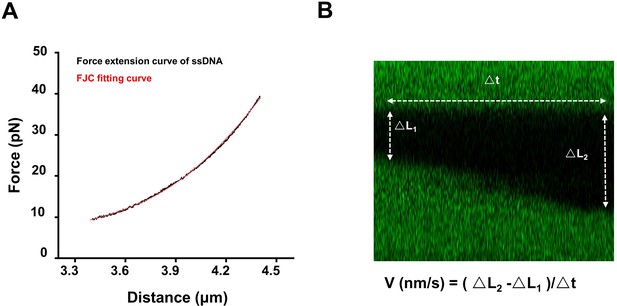
Elasticity parameters of ssDNA and DNA unwinding rate calculation.
(A) Elasticity parameters of ssDNA were necessary for data conversion. They were obtained from the DNA force-extension measurements. Force-extension curve of this ssDNA (8358 nt) in reaction buffer containing 50 nM Sytox was shown in black. The force-extension relation of ssDNA was described as extensible freely jointed-chain (FJC) model. The fitting result was shown in red. The contour length was 4.07 ± 0.12 nm and stretch module was 297.6 ± 11.8 pN (n = 6). (B) Kymographs were used to calculate DNA unwinding rate by BLM from a nick. It was obtained from fitting to the linear region of the increase of the unwinding fork versus time. The velocity in nm/s was further converted to bp/s based on the elasticity parameters.
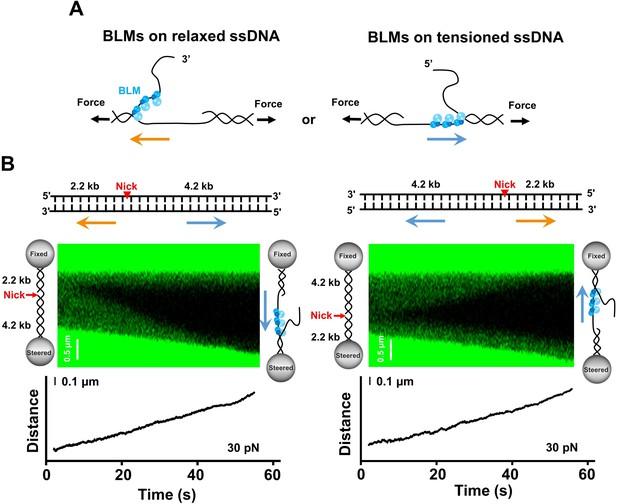
BLM unwinds DNA template containing a single nick.
(A) Two models of BLM unwinding dsDNA from a nick. BLM initiates unidirectional dsDNA unwinding from a nick by translocating either on the tensioned ssDNA or on the relaxed ssDNA. The unwinding polarities are opposite in these two scenarios. (B) The 6.4 kbp dsDNA template unwinding in the presence of 200 nM BLM. The 6.4 kbp dsDNA contains a nick located at 2.2 kbp and 4.2 kbp from the 5’ and 3’ termini, respectively. Representative kymographs and DNA length signals showed the 4.2 kbp dsDNA segments were always unwound under 30 pN. The arrows indicate the directions of the unwinding fork movement.
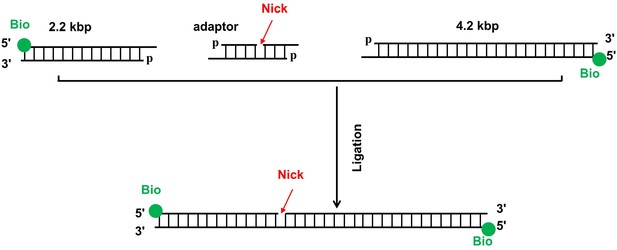
Construction of the 6.4 kbp DNA template.
The 6.4 kbp nicked DNA template containing a single nick consists of two DNA segments (2.2 kpb and 4.2 kbp) connected by an adaptor. The two DNA segments were PCR amplified from the plasmid pBR322 using a biotin-labeled primer. The resulting DNA fragments were digested with BstXI to create an overhang. The adaptor was a product by annealing three oligonucleotides where a nick was automatically generated due to the missing of the 5’ phosphate of one oligonucleotide. The final product was produced by ligating the two DNA segments with the adaptor at 1:1:1 ratio using T4 ligase.
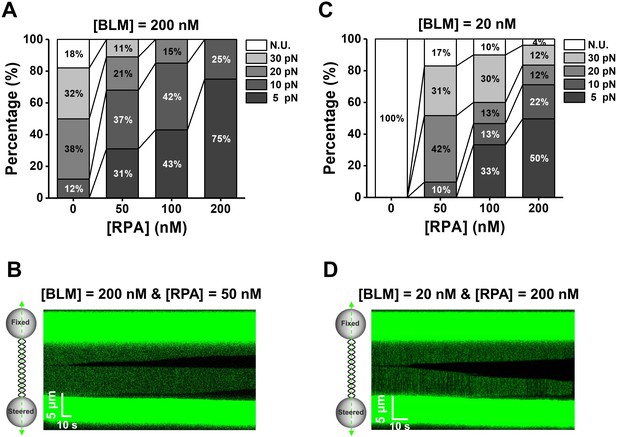
BLM unwinds dsDNA in the presence of RPA.
(A, C) Distributions of the required forces to monitor DNA unwinding in the presence of 200 nM (A) or 20 nM (C) BLM and RPA with indicated concentrations. N.U. represents no unwinding. (B, D) Kymographs of tethered λ phage DNA showing DNA unwinding in the presence of 50 nM RPA and 200 nM BLM (B) or 200 nM RPA and 20 nM (D) BLM under 5 pN.
-
Figure 3—source data 1
The required forces for BLM/RPA-mediated DNA unwinding.
- https://cdn.elifesciences.org/articles/54098/elife-54098-fig3-data1-v2.xlsx
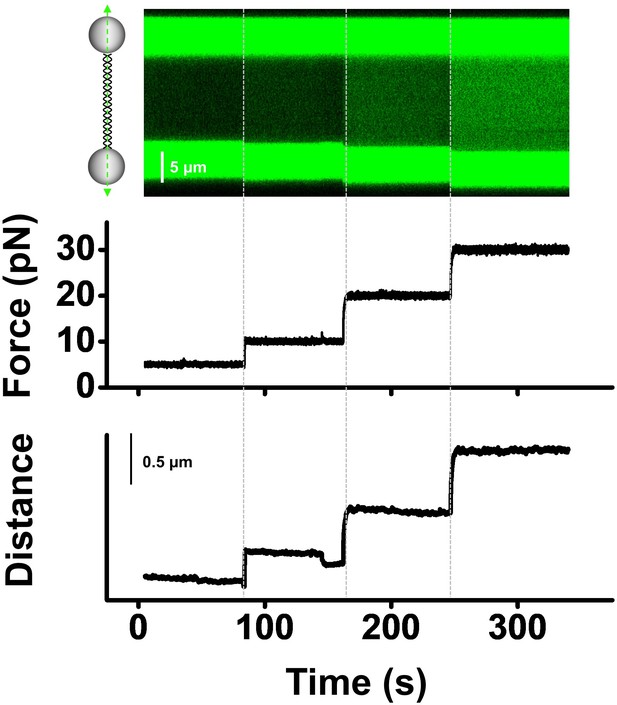
RPA alone was incapable of unwinding DNA.
To confirm that the observed unwinding in the presence of BLM and RPA was a result of BLM’s helicase activity, we conducted the unwinding experiment in the absence of ATP. In the presence of 200 nM BLM and 200 nM RPA, we started the experiment at 10 pN force for ~1 min and increased to 10/20/30 pN orderly. There was no change of either fluorescent or DNA length signal (n = 10), indicating that the unwinding signals we observed were indeed due to BLM’s helicase activity.
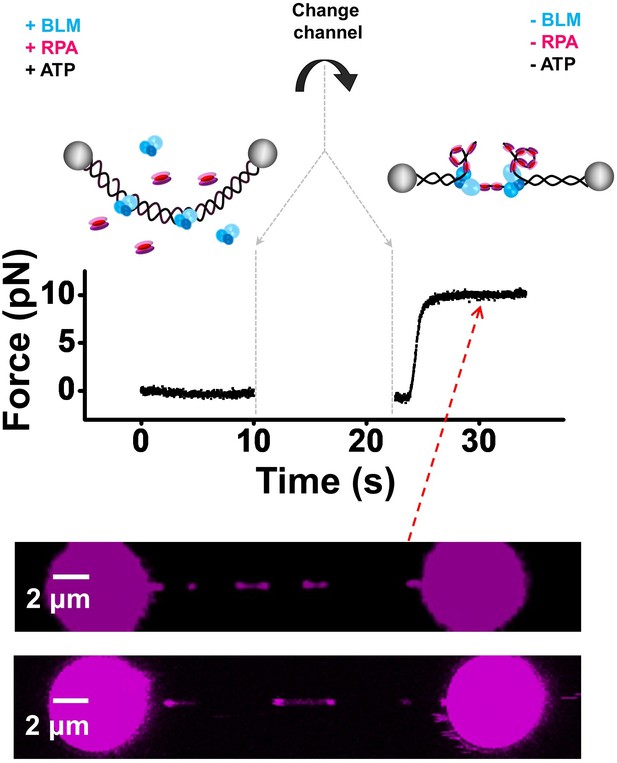
BLM and RPA unwind nicked DNA in the absence of external force.
To determine whether BLM and RPA can unwind nicked DNA in the absence of force, we designed a new DNA unwinding assay. We first incubated a relaxed λ DNA tether (under zero force) in a channel containing 200 nM WT BLM and 400 nM eGFP-RPA for ~10 s. The relaxed DNA tether was then transported to a buffer channel wherein DNA unwinding could not occur due to the absence of BLM, RPA and ATP. In this channel, we stretched the DNA tether to 10 pN and examined the fluorescence signal along the DNA template before RPA dissociates. As shown above, the bidirectional unwinding DNA intermediates were detected (n = 10), indicating RPA and BLM can indeed unwind nicked DNA in the first channel in which no external force exists.
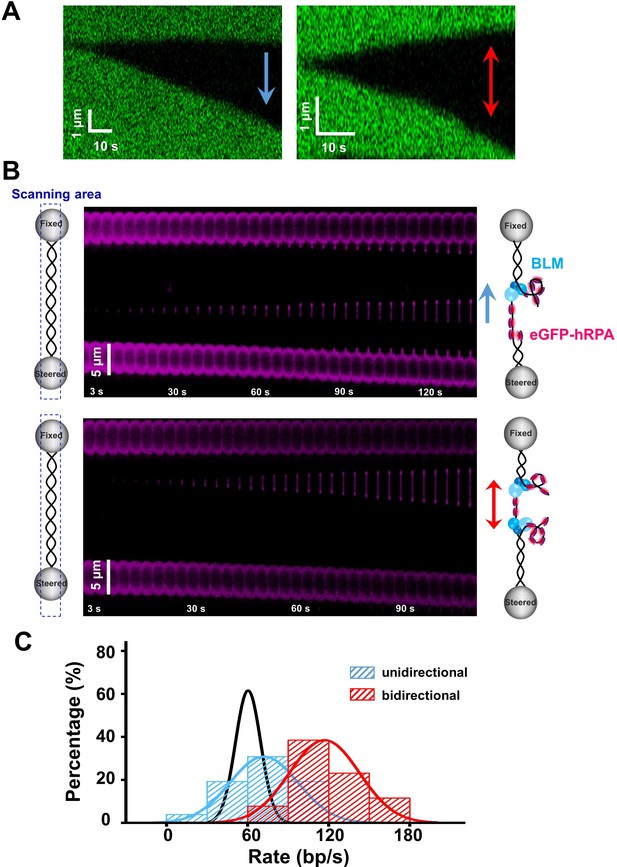
BLM’s RPA-activated bidirectional unwinding from a nick.
(A) Kymographs of individual unwinding events of a tethered λ phage DNA in the presence of 200 nM BLM and 50 nM RPA under 30 pN showing unidirectional and bidirectional DNA unwinding. The arrows indicate unwinding directions. (B) Representative kymographs of unidirectional (up) and bidirectional (down) DNA unwinding in the presence of 200 nM WT BLM and 50 nM eGFP-RPA. To illustrate the coiled ssDNAs, confocal laser scanned a rectangle area instead of only the DNA template track. The arrows indicate the directions of the unwinding fork movement. (C) Distributions of the uni- and bi- directional dsDNA unwinding rates in the presence of 200 nM BLM and 50 nM RPA under 30 pN. p-value<0.01 calculated by Student’s t test. These rate distributions were compared with that in the presence of 200 nM BLM alone (black).
-
Figure 4—source data 1
The uni- and bi- directional dsDNA unwinding rates.
- https://cdn.elifesciences.org/articles/54098/elife-54098-fig4-data1-v2.xlsx
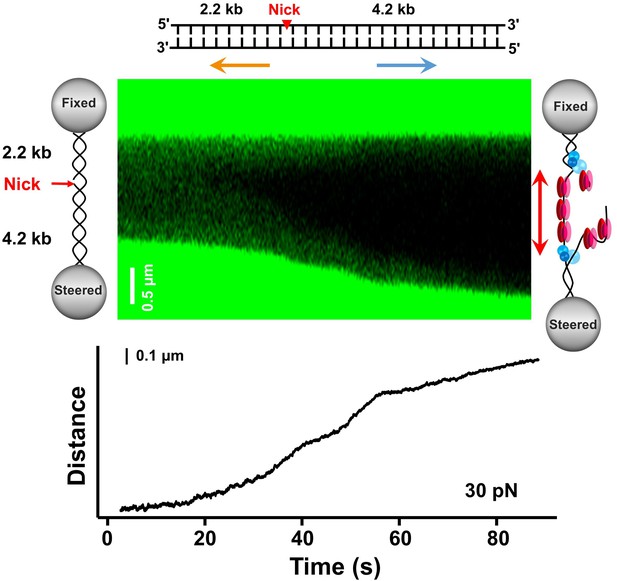
RPA supports BLM’s bidirectional unwinding on the 6.4 kbp nicked DNA template.
A kymograph of an individual unwinding event on the 6.4 kbp nicked DNA template shows bidirectional DNA unwinding (50 nM RPA and 200 nM WT BLM). The red arrows indicate the directions of the unwinding fork movement.
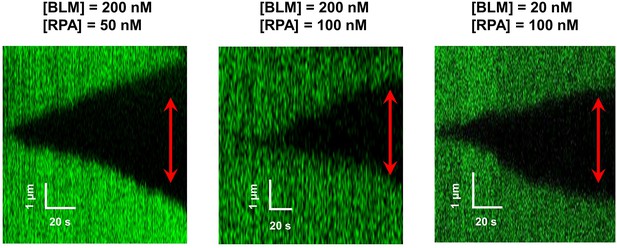
RPA supports BLM’s bidirectional unwinding under low forces.
Under 5 pN, bidirectional unwinding events were also observable when RPA and BLM were present. We showed representative kymographs of bidirectional DNA unwinding events at 5 pN under different conditions. The red arrows indicate the directions of the unwinding fork movement.
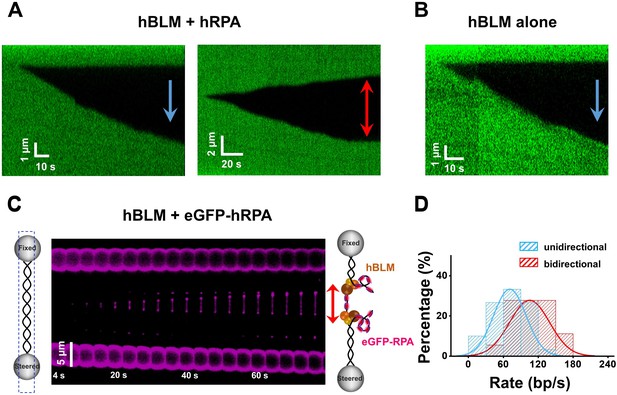
hBLM unwinds dsDNA in the presence and absence of hRPA.
(A) Kymographs of individual unwinding events showing the unidirectional unwinding (left) and bidirectional unwinding (right) in the presence of 200 nM hBLM and 100 nM hRPA under 30 pN. The arrow indicates the direction of the unwinding fork movement. In this experimental condition, 18 out of 48 unwinding events showed bidirectional DNA unwinding and the rest showed unidirectional DNA unwinding. (B) Kymograph of an individual unwinding event showing the unidirectional unwinding in the presence of 200 nM hBLM alone under 30 pN. Only unidirectional DNA unwinding was detected with hBLM alone (n = 15). (C) Kymograph of bidirectional DNA unwinding in the presence of 200 nM hBLM and 100 nM eGFP-hRPA. To illustrate the coiled ssDNAs, confocal laser scanned a rectangle area instead of only the DNA template track. The arrows indicate the directions of the unwinding fork movement. (D) Distributions of the uni- and bi- directional dsDNA unwinding rates in the presence of of 200 nM hBLM and 100 nM hRPA under 30 pN. p-value<0.01 calculated from Student’s t test.
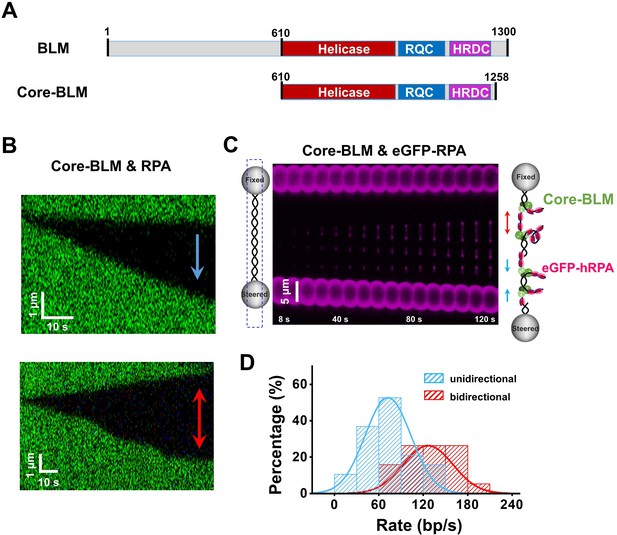
RPA supports core-BLM’s bidirectional unwinding from a nick.
(A) Schematic representations of WT BLM and core-BLM. Helicase, RQC and HRDC domains are shown in different colors. (B) Kymograph of an individual unwinding event in the presence of 20 nM core-BLM and 50 nM RPA under 35 pN showing unidirectional (up) and bidirectional (down) DNA unwinding. The arrows indicate the directions of the unwinding fork movement. (C) A representative kymograph of a single DNA tether unwound in the presence of 20 nM core-BLM and 50 nM eGFP-RPA. To demonstrate the coiled ssDNAs, confocal laser scanned a rectangle area instead of the DNA template track only. (D) Distributions of the uni- and bi- directional dsDNA unwinding rates in the presence of 20 nM BLM and 50 nM RPA under 35 pN. p-value<0.01 calculated by Student’s t test.
-
Figure 5—source data 1
The uni- and bi- directional dsDNA unwinding rates.
- https://cdn.elifesciences.org/articles/54098/elife-54098-fig5-data1-v2.xlsx
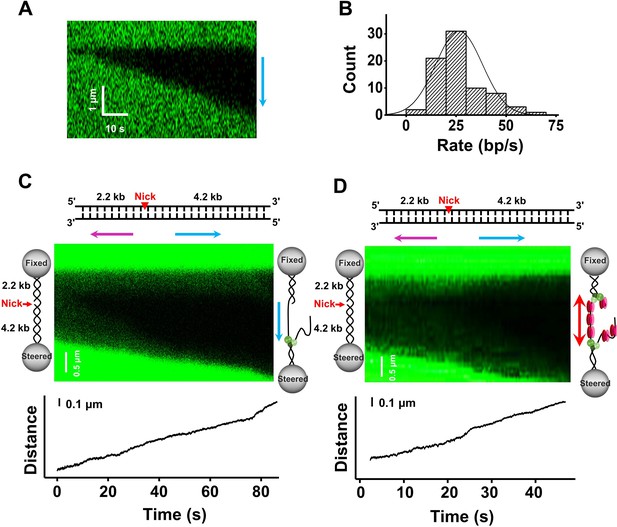
Core-BLM unwinds dsDNA in the presence and absence of RPA.
(A) Kymograph of an individual unwinding event showing the unidirectional unwinding in the presence of 200 nM core-BLM under 30 pN. The arrow indicates the direction of the unwinding fork movement. In all examined unwinding events (n = 48), DNA unwinding occurred unidirectionally. (B) Distribution of the dsDNA unwinding rates in the presence of 200 nM core-BLM under 30 pN. (C) The 6.4 kbp dsDNA template unwinding in the presence of 200 nM core-BLM. A representative kymograph and DNA length signal showed the 4.2 kbp dsDNA segment was unwound under 35 pN. In all examined traces (n = 27), only the 4.2 kbp dsDNA segment was unwound, indicating that core-BLM unwinds unidirectionally by translocating on the tensioned ssDNA. (D) A kymograph of an individual unwinding event in the presence of 50 nM RPA and 200 nM core-BLM showing bidirectional unwinding. The arrows indicate the directions of the unwinding fork movement.
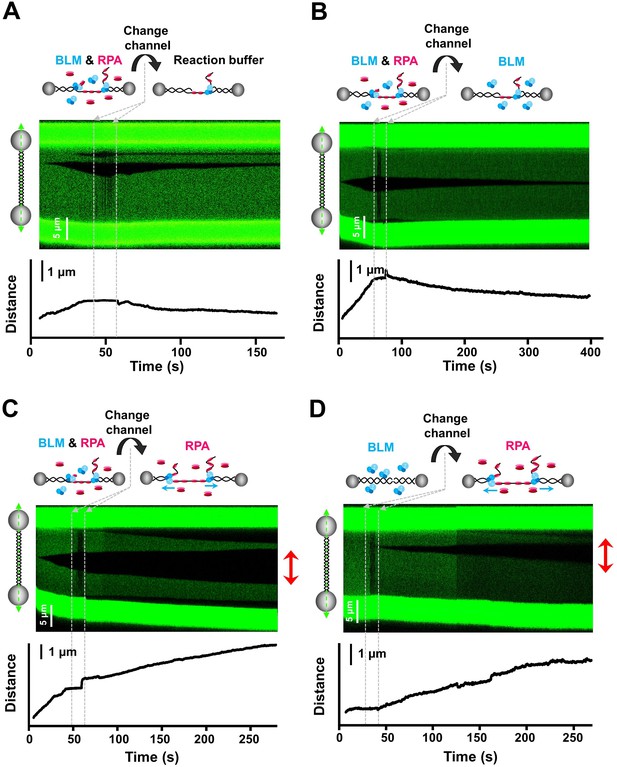
Free RPAs are indispensable for stimulating BLM-mediated DNA unwinding.
(A–C) Kymographs and DNA length vs. time traces of DNA tethers showing dsDNA unwinding initiating in a channel containing 20 nM BLM and 50 nM RPA under 30 pN, followed by quickly transporting to a channel containing ATP reaction buffer (A), 20 nM BLM (B) or 50 nM RPA (C) only. Red arrows indicate bidirectional DNA unwinding. (D) Kymograph and DNA length vs. time of a DNA tether showing the DNA template incubated in a channel containing 20 nM BLM under 30 pN, followed by transporting to a channel containing 50 nM RPA. Red arrows indicate bidirectional DNA unwinding.
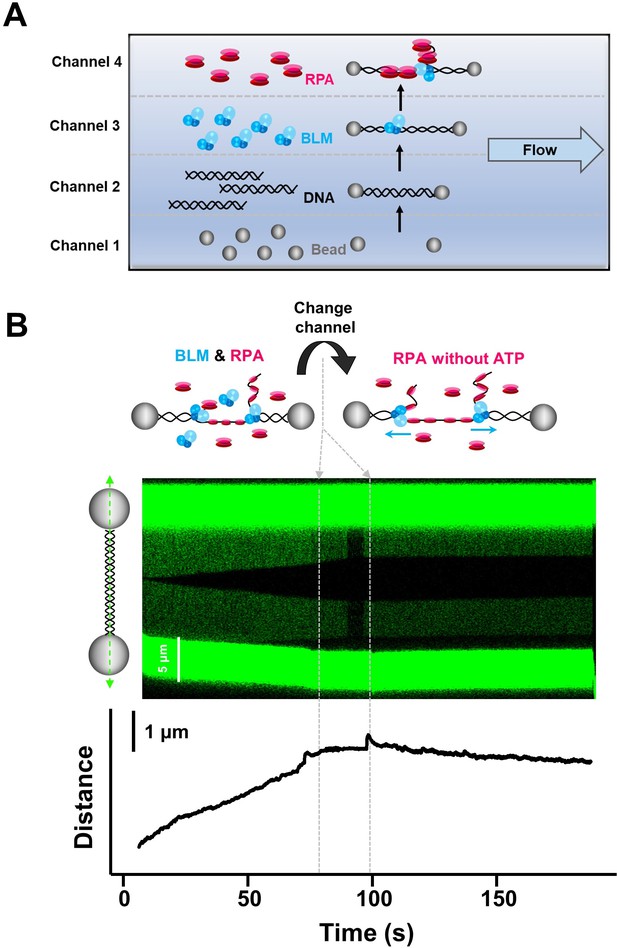
The micro-flow cell assay confirmed the observe DNA unwinding requires BLM’s unwinding activity.
(A) Schematic of the 4-channel micro-flow cell. Two beads were optically trapped in channel 1. A single DNA molecule was caught between the two beads and a DNA tether was formed in channel 2. The DNA molecule was incubated with the studied proteins in channels 3 and 4 and the unwinding activity was monitored. The buffer conditions as well as proteins in channels 3 and 4 were specified in the main text for each experiment. (B) Kymograph and DNA length vs. time trace of DNA tether showing dsDNA unwinding initiating in a channel containing 20 nM BLM and 50 nM RPA under 35 pN, followed by transporting to a channel containing 50 nM RPA and 0 mM ATP. In the following RPA channel, DNA unwinding was immediately terminated for all examined traces (n = 8).
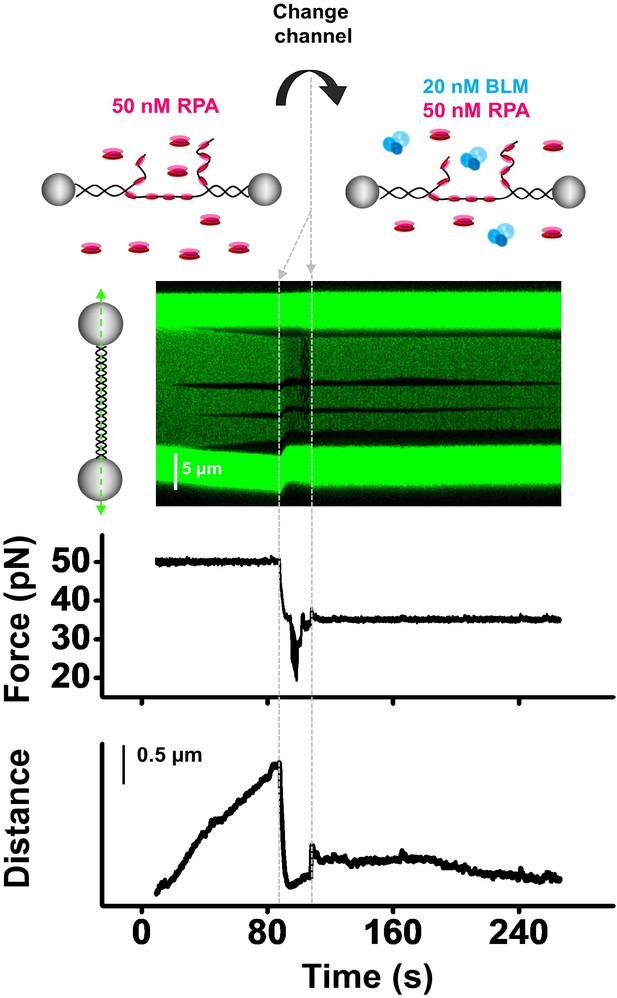
RPA cannot recruit BLM to the DNA unwinding fork.
To examine whether RPA can recruit WT BLM to the unwinding fork, we conducted the experiments by first placing the tether in the channel containing 50 nM RPA without ATP under 50 pN where RPA spontaneously bound to and denatured dsDNA as indicated by both DNA length and fluorescence signals. After that, we moved the tethered DNA to a channel containing 20 nM BLM and 50 nM RPA and reduced the force to 35 pN. In this channel, up to 83% of the unwinding events showed no DNA unwinding (n = 33).
Tables
Reagent type (species) or resource | Designation | Source or reference | Identifiers | Additional information |
---|---|---|---|---|
Gene (Gallus gallus) | gBLM | NCBI | RRID:SCR:006472 | NP_001007088.2 |
Gene (Homo sapiens) | hBLM | NCBI | RRID:SCR:006472 | NP_000048.1 |
Strain, strain background (Escherichia coli) | 2566 | This paper | Competent cell | |
Strain, strain background (Escherichia coli) | BL21(DE3) | This Paper | Competent cell | |
Recombinant DNA reagent | Lambda DNA | Thermo Fisher Scientific | SD0021 | |
Recombinant DNA reagent | pBR322 (plasmid) | Takara | RRID: Addgene_10877 | |
Recombinant DNA reagent | pTWIN1 (plasmid) | New England BioLabs | N6951S | Expression of core BLM in E. coli |
Recombinant DNA reagent | pET21a-sumo (plasmid) | This paper | Expression of BLM in E. coli | |
Recombinant DNA reagent | p11d-tRPA (plasmid) | Vector Builder | Addgene:102613 | Expression of human RPA in E. coli |
Peptide, recombinant protein | T4 DNA ligase | New England BioLabs | M0202L | |
Peptide, recombinant protein | Klenow Fragment, exo- | Thermo Fisher Scientific | EP0421 | |
Peptide, recombinant protein | Nde I | New England BioLabs | R0111S | |
Peptide, recombinant protein | Sal I | New England BioLabs | R3138S | |
Peptide, recombinant protein | BstX I | New England BioLabs | R0113L | |
Chemical compound, drug | Biotin-dATP | Invitrogen | Invitrogen: 19524016 | |
Chemical compound, drug | Biotin-dCTP | Invitrogen | Invitrogen: 19518018 | |
Chemical compound, drug | IPTG | Thermo Fisher Scientific | 15529019 | |
Commercial assay, kit | Streptavidin Coated Polystyrene Particles (1.76 µm) | Spherotech | AG02 | |
Commercial assay, kit | Streptavidin Coated Polystyrene Particles (4.42 µm) | Spherotech | AL01 | |
Commercial assay, kit | DNA Purification | Sangon Biotech | B518141 | |
Commercial assay, kit | SP Sepharose Fast Flow | GE Healthcare | 17072904 | |
Commercial assay, kit | Q Sepharose Fast Flow | GE Healthcare | 17051004 | |
Commercial assay, kit | Sytox orange Nucleic Acid Stain | Thermo Fisher Scientific | S11368 | 5 mM |
Software, algorithm | MATLAB, Data analysis | MathWorks | RRID: SCR:001622 |