Presynaptic PTPσ regulates postsynaptic NMDA receptor function through direct adhesion-independent mechanisms
Figures
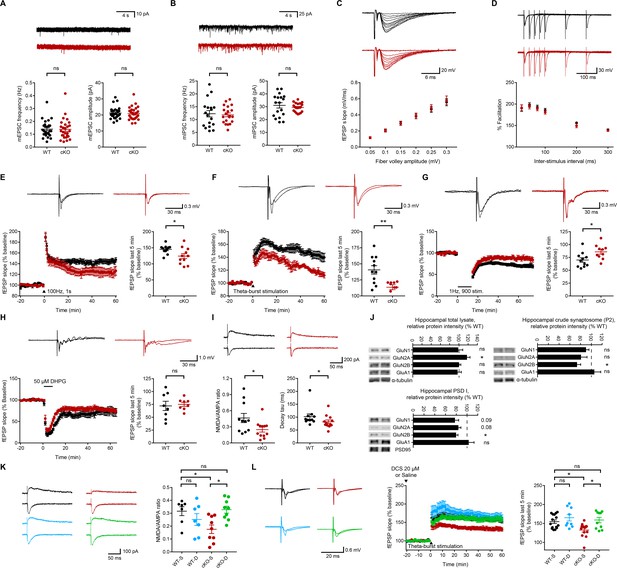
Normal spontaneous and evoked basal synaptic transmission but suppressed NMDAR-dependent synaptic transmission and plasticity in the Emx1-Cre;Ptprsfl/fl hippocampus.
(A) Normal mEPSCs in CA1 pyramidal neurons from Emx1-Cre;Ptprsfl/fl mice (P18–22). (n = 27 cells from six mice [WT] and 28, 7 [cKO], ns, not significant, Mann-Whitney test). (B) Normal mIPSCs in CA1 pyramidal neurons from Emx1-Cre;Ptprsfl/fl mice (P18–22). (n = 18, 4 [WT] and 20,4 [cKO], ns, not significant,Student’s t-test [amplitude], Welch’s correction [frequency]). (C) Normal evoked basal excitatory transmission at Schaffer collateral-CA1 pyramidal cell (SC-CA1) synapses in Emx1-Cre;Ptprsfl/fl mice (P26-30), as shown by fEPSP slopes plotted against fiber volley amplitude. (n = 14 slices from 5 mice and 14, 6 [WT, cKO], ns, not significant, repeated measures two-way ANOVA). (D) Normal paired-pulse ratio at SC-CA1 synapses of Emx1-Cre;Ptprsfl/fl mice (P26–30), as shown by percent facilitation plotted against inter-pulse intervals. (n = 17, 5 [WT] and 15, 5 [cKO], ns, not significant, repeated-measures/RM two-way ANOVA). (E) Suppressed HFS-LTP at Emx1-Cre;Ptprsfl/fl SC-CA1 synapses (P26-32). (n = 11, 6 [WT] and 11, 6 [cKO], *p<0.05, Student’s t-test). (F) Suppressed TBS-LTP at Emx1-Cre;Ptprsfl/fl SC-CA1 synapses (P26-32). (n = 11, 4 [WT] and 9, 4 [cKO], **p<0.01, Student’s t-test). (G) Suppressed LFS-LTD at Emx1-Cre;Ptprsfl/fl SC-CA1 synapses (P16-19). (n = 11, 6 [WT] and 10, 5 [cKO], *p<0.05, Student’s t-test). (H) Normal mGluR-LTD induced by DHPG (50 μM) at Emx1-Cre;Ptprsfl/fl SC-CA1 synapses (2–3 weeks). (n = 8, 7 [WT] and 8, 6 [cKO], ns, not significant, Student’s t-test). (I) Decreases in the ratio of NMDAR-EPSCs and AMPAR-EPSCs and the decay tau of NMDAR-EPSCs at Emx1-Cre;Ptprsfl/fl SC-CA1 synapses (P18-23). (n = 11 cells from five mice [WT] and 12, 5 [cKO], *p<0.05, Student’s t-test [NMDA/AMPA ratio], Mann-Whitney test [decay tau]). (J) Decreased levels of the GluN2B, but not GluN1 or GluN2A, subunit of NMDARs in crude synaptosomal (P2) and PSD I fractions, but not in total lysates, of the Emx1-Cre;Ptprsfl/fl hippocampus (3 weeks), compared with those in WT mice. α-tubulin was blotted for controls. GluA1, AMPAR subunit. (n = 7 mice (WT/cKO total lysates, 6,4 [WT and cKO P2], n = 3, 3 [WT and cKO PSD], *p<0.05, one sample t-test). (K) D-cycloserine (20 μM) rescues the NMDA/AMPA ratio at Emx1-Cre;Ptprsfl/fl SC-CA1 synapses (P19–22). (n = 7 cells from four mice [WT-V/vehicle], 7, 3 [WT-D/D-cycloserine], 9, 4 [cKO-V], 9, 4 [cKO-D], *p<0.05, ns, not significant, two-way ANOVA with Sidak’s test). (L) D-cycloserine (20 μM) rescues TBS-LTP at at Emx1-Cre;Ptprsfl/fl SC-CA1 synapses (P28–32), without affecting TBS-LTP at WT synapses. (n = 15 slices from six mice [WT-V], 13, 5 [cKO-V], 9, 3 [WT-D], 10, 3 [cKO-D], *p<0.05, ns, not significant, two-way ANOVA with Sidak’s test).

Generation and basic characterization of global Ptprs-mutant mice and Emx1-Cre;Ptprsfl/fl mice.
(A) Conditional KO strategy for the production of Emx1-Cre;Ptprsfl/fl cKO mice. (B) PCR genotyping of Ptprsfl/fl and Emx1-Cre alleles. (C) Reduced levels of PTPσ proteins in hippocampal lysates of global Ptprs-mutant mice (gHT/Ptprs+l–, heterozygotes; gKO/Ptprs–l–, homozygotes) and Emx1-Cre;Ptprsfl/fl cKO mice at P21, compared with WT and Ptprsfl/fl mice, as revealed by immunoblot analyses using anti-PTPσ antibodies directed against N-terminal (Ig1+two domains) and C-terminal (last 30 aa residues) regions of the PTPσ protein. A non-specific band recognized by the C-terminal antibody is indicated by an asterisk. (D) Reduced body weights of global Ptprs-mutant mice, but largely normal body weights of Emx1-Cre;Ptprsfl/fl mice, during the first three postnatal weeks. (n = 37/10 mice for WT/gKO, 22/23 mice for WT/cKO, ***p<0.001, two-way ANOVA with Sidak’s test). (E) Abnormal walking patterns in Ptprs-mutant mice, but normal walking patterns of Emx1-Cre;Ptprsfl/fl mice, as revealed by monitoring the walking of mice with painted feet (blue, fore; red, hind) on paper. (F) Normal gross morphology of the brain of Emx1-Cre;Ptprsfl/fl mice (8 weeks), as shown by DAPI staining. Scale bar, 2 mm. (G) Distribution patterns of PTPσ–β-galactosidase fusion proteins, as revealed by X-gal staining of coronal brain slices from WT mice targeted with the β-geo + Ptprs flox vector (8 weeks). Scale bar, 2 mm.
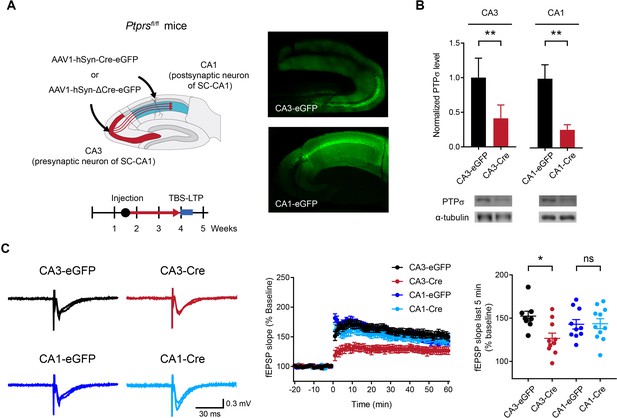
Deletion of presynaptic, but not postsynaptic, PTPσ suppresses TBS-LTP at SC-CA1 synapses.
(A) Diagram depicting deletion of presynaptic PTPσ in the CA3 region or postsynaptic PTPσ in the CA1 region, induced by local injection of AAV1-hSyn-Cre-eGFP (Cre recombinase fused to EGFP); mice injected with AAV1-hSyn-ΔCre-eGFP (lacking Cre) served as controls. TBS-LTP experiments were conducted ~2.5 weeks later. Local expression of Cre recombinase was further confirmed by EGFP fluorescence in controls co-injected with AAV1-hSyn-eGFP (right) and by reduced PTPσ protein levels (panel B). (B) Decreased PTPσ protein levels in CA3 and CA1 regions of Ptprsfl/fl mice injected with AAV1-hSyn-Cre-eGFP (CA3/CA1-Cre) compared with those in Ptprsfl/fl mice injected with AAV1-hSyn-ΔCre-eGFP (CA3/CA1-EGFP). (n = 5 mice [CA3-eGFP], 5 [CA3-Cre], 4 [CA1-eGFP] and 3 [CA1-Cre], *p<0.05, **p<0.01, one-sample t-test). (C) Suppressed TBS-LTP at SC-CA1 synapses of Ptprsfl/fl mice (4 weeks) induced by knocking out PTPσ in the CA3 region (but not CA1 region) by local injection of AAV1-hSyn-Cre-eGFP, compared with TBS-LTP in control Ptprsfl/fl mice injected with AAV-hSyn-ΔCre-eGFP. (n = 8 slices from five mice [CA3-eGFP], 10, 4 [CA3-Cre], 10, 4 [CA1-eGFP] and 11, 5 [CA1-Cre], *p<0.05, ns, not significant, two-way ANOVA with Sidak’s test).
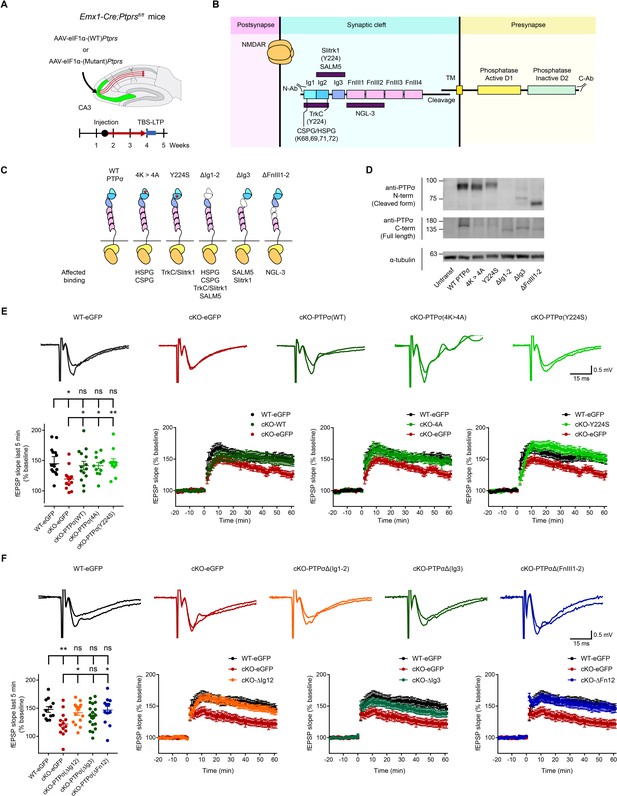
Re-expression of presynaptic PTP σ rescues postsynaptic LTP in the hippocampus through mechanisms independent of the extracellular region of PTPσ.
(A) Diagram depicting re-expression of WT and mutant PTPσ proteins in the CA3 region of the hippocampus by local injection of AAV(php.eB)-eIF1a-Ptprs (WT and mutants), followed by measurement of TBS-LTP. (B) Diagram depicting the domain structures of PTPσ and extracellular and cytoplasmic regions/domains involved in protein-protein interactions or tyrosine phosphatase activity. The first three Ig domains are important for trans-synaptic adhesions with Slitrk1, TrkC, CSPG/HSPG or SALM5, and the residues Y224 and K68/K69/K71/K72 are important for Slitrk1 and CSPG/HSPG interactions, respectively. (C) Specific PTPσ mutants used in our experiments, with point mutations or small deletions in the extracellular domains. All binding partners of PTPσ affected by the mutations/deletions are also indicated. (D) Expression levels and sizes of PTPσ mutants, revealed by immunoblot analysis of HEK293T cell lysates using two independent PTPσ antibodies targeting the N-terminal region (~Ig1-2) and C-terminus (last 30 residues) that can detect all PTPσ mutants, except for PTPσ-ΔIg12, which is not detected by the N-terminal antibody. (E) Rescue of TBS-LTP at SC-CA1 synapses by re-expression of WT PTPσ as well as mutant PTPσ (lacking CSPG/HSPG and Slitrk1 interactions) in the CA3 region of Emx1-Cre;Ptprsfl/fl mice (P28–32) through local injection of AAV-eIF1a-Ptprs-WT/mut. In control experiments, AAV-hSyn-eGFP was injected into the CA3 region of both Ptprsfl/fl (WT) and Emx1-Cre;Ptprsfl/fl mice. (n = 14 slices from four mice [WT-eGFP], 13, 5 [cKO-eGFP], 14, 6 [cKO-PTPσ-WT], 11, 4 [cKO-PTPσ−4A], and 11, 4 [cKO-PTPσ-Y224S], *p<0.05, **p<0.01, ns, not significant, one-way ANOVA with Dunnett’s test). (F) Full and partial rescue of TBS-LTP at SC-CA1 synapses by re-expression of mutant PTPσ lacking Ig1+2 (ΔIg12), Ig3 (ΔIg3), or FNIII1+2 (ΔFN12) domains in the CA3 region of Emx1-Cre;Ptprsfl/fl mice (P28–32) by local injection of AAV-eEF1-Ptprs. Note that expression of PTPσ-ΔIg3 induces a partial rescue. Control virus (AAV-eIF1a-eGFP) was injected into the CA3 region of both Ptprsfl/fl (control) and Emx1-Cre;Ptprsfl/fl mice. (n = 12 slices from four mice [WT-eGFP], 13, 4 [cKO-eGFP], 16, 5 [cKO-PTPσ-ΔIg12], 20, 6 [cKO-PTPσ-ΔIg3], and 13, 5 [cKO-PTPσ-ΔFn12], *p<0.05, **p<0.01, ns, not significant, one-way ANOVA with Dunnett’s test).

PTPσ adhesions with known postsynaptic partners, and identification of the amino acid residues involved in the interactions.
(A) PTPσ adhesions with known postsynaptic partners based on reported complex structures and molecular modeling of the SALM5-PTPσ complex. (B) Amino acid residues involved in the interactions between PTPσ and PTPσ-binding postsynaptic adhesion molecules based on reported or modeled complex structures. Note that IL1RAPL1 and IL-1RAcP have been shown to interact with PTPδ but not PTPσ (Yamagata et al., 2015; Yoshida et al., 2012; Yoshida et al., 2011).
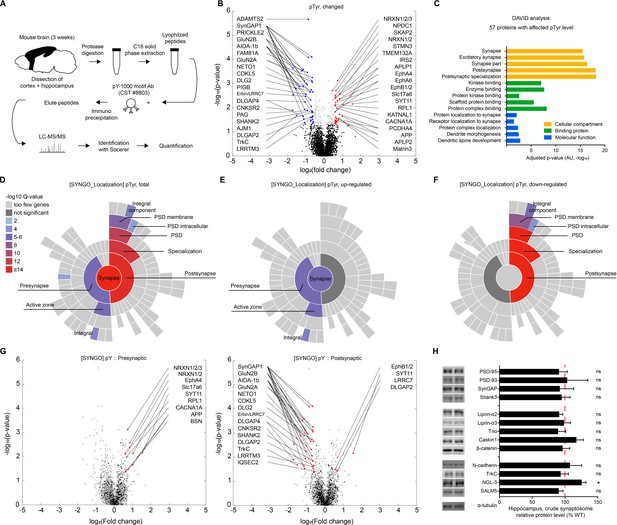
Proteins with altered pTyr levels in the Emx1-Cre;Ptprsfl/fl cortex and hippocampus.
(A) Diagram showing procedures for the identification of proteins with altered pTyr levels. (B) A volcano plot showing proteins with significantly up- or downregulated pTyr levels (fold change >1.5, p<0.05) in the cortex and hippocampus of Emx1-Cre;Ptprsfl/fl (P21). Samples from three WT or KO mice were pooled for each analysis. Note that a single protein can be linked to multiple dots (different pTyr motifs); conversely, the same peptide can belong to two different proteins (e.g., Erbin and LRRC7). (C) Functional analysis (DAVID GO analysis) of proteins from Emx1-Cre;Ptprsfl/fl mice with significantly altered pTyr levels. Note that synapse-related GO terms are strongly enriched. (D–F) Sunburst plots from SynGO analyses of specific pre- and postsynaptic localizations of proteins with altered pTyr levels (total, upregulated, and downregulated) from the Emx1-Cre;Ptprsfl/fl cortex and hippocampus. Note that upregulated proteins tend to be those that localize to presynaptic sites, whereas downregulated proteins tend to be those that localize to postsynaptic sites. (G) Volcano plots showing that presynaptic proteins, determined by SynGO analyses, showed mainly increased pTyr levels, whereas postsynaptic proteins showed mainly decreased pTyr levels. (H) Largely normal synaptic levels of PTPσ-related proteins in the hippocampus of Emx1-Cre;Ptprsfl/fl mice (3 weeks), as shown by immunoblot analyses of crude synaptosomes. (n = 6 mice for WT and cKO and for some, 6, five mice for WT and cKO, *p<0.05, ns, not significant, one-sample t-test).
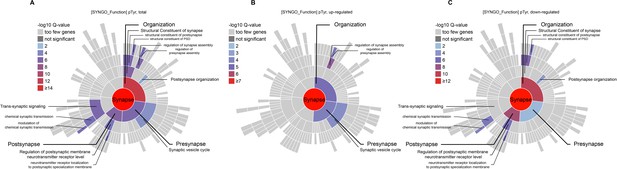
SynGO analysis of the functions of proteins with altered pTyr Levels in the Emx1-Cre;Ptprsfl/fl cortex and hippocampus.
(A–C) Sunburst plots from SynGO analyses of the function of proteins with altered pTyr levels (total, upregulated, and downregulated) from the Emx1-Cre;Ptprsfl/fl cortex and hippocampus. Note that proteins with upregulated pTyr proteins are strongly associated with ‘organization’ and ‘presynapse’ functions, whereas those with downregulated pTyr levels are associated with ‘organization’ and ‘postsynapse’ functions.
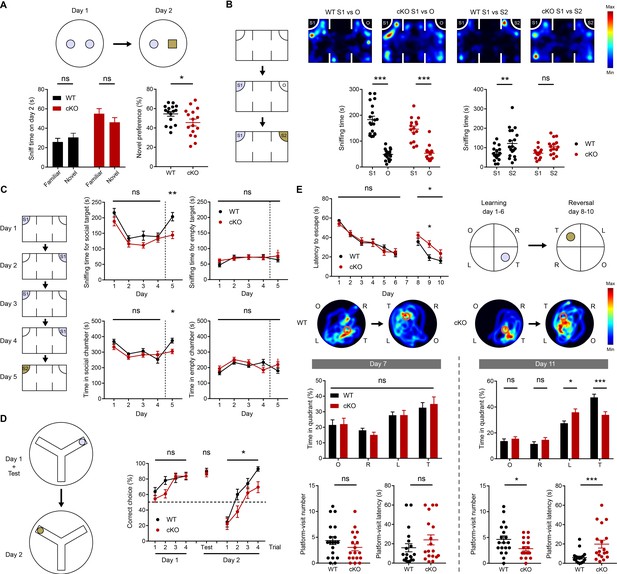
Suppressed novelty recognition in Emx1-Cre;Ptprsfl/fl mice.
(A) Impaired novel-object recognition in Emx1-Cre;Ptprsfl/fl mice (2–3 months). (n = 16 mice for WT and cKO, *p<0.05, ns, not significant, Student’s t-test). (B) Normal social approach, but suppressed social-novelty recognition, in Emx1-Cre;Ptprsfl/fl mice (2–3 months) in the three-chamber test. S1, first/initial social stranger; O, object; S2, second/new social stranger. (n = 19 [WT] and 15 [cKO], **p<0.01, ***p<0.001, ns, not significant, RM two-way ANOVA with Sidak’s test). (C) Normal social recognition and habituation, but suppressed social-novelty recognition, in Emx1-Cre;Ptprsfl/fl mice (2–3 months) in a modified three-chamber test, in which the subject mouse was exposed to the initial stranger mouse for four consecutive days and introduced to a new stranger mouse on day 5. (D) Suppressed reward-arm recognition in the reversal, but not initial, phase of the Y-maze in Emx1-Cre;Ptprsfl/fl mice (2–3 months). (n = 21 [WT] and 22 mice [cKO] for initial session, n = 17, 19 for reversal session, *p<0.05, ns, not significant, RM two-way ANOVA [initial/reversal] and Mann-Whitney test [test on day 2]). (E) Impaired learning and memory in the reversal, but not initial, phase of the Morris water maze in Emx1-Cre;Ptprsfl/fl mice (3–4 months). (n = 19 [WT] and 18 [cKO], *p<0.05, ***p<0.001, ns, not significant, RM two-way ANOVA with Sidak’s test [latency to escape], Student’s t-test [platform visit number] and Mann-Whitney test [platform visit latency]).
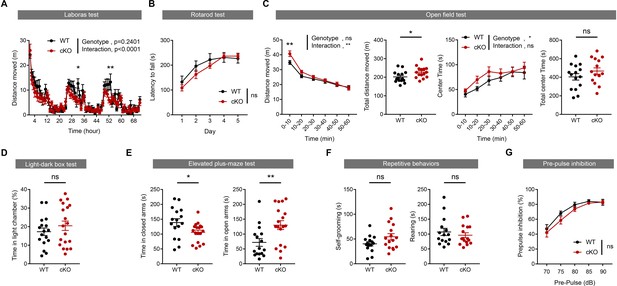
Emx1-Cre;Ptprsfl/fl mice show moderately changed locomotion and anxiety-like behavior, but normal motor coordination, repetitive behavior, and prepulse inhibition.
(A) Moderately decreased locomotor activity in Emx1-Cre;Ptprsfl/fl mice (2 months) in Laboras cages, representing a familiar environment, as indicated by distance moved. (n = 10 mice [WT] and 15 mice [cKO], *p<0.05, **p<0.01, RM two-way ANOVA with Sidak’s test). (B) Normal motor coordination and learning in Emx1-Cre;Ptprsfl/fl mice (2 months) in the rota-rod test. (n = 12 mice [WT] and 13 [cKO], ns, not significant, RM two-way ANOVA). (C) Moderately increased locomotor activity in Emx1-Cre;Ptprsfl/fl mice (2 months) in the open-field test (a novel environment), as indicated by distance moved. Note that the time spent in the center region of the open-field arena is moderately increased, as supported by the genotype difference, although not by the total center time, suggesting modereatley increased anxiolytic-like behavior. (n = 23 [WT] and 23 [cKO], *p<0.05, **p<0.01, ns, not significant, RM two-way ANOVA with Sidak’s test [distance moved, center time], Student’s t-test [total distance moved, total time spent in center]). (D) Normal anxiety-like behaviors in Emx1-Cre;Ptprsfl/fl mice (2 months) in the light-dark chamber test. (n = 17 [WT] and 19 [cKO], ns, not significant, Mann-Whitney test). (E) Anxiolytic-like behaviors in Emx1-Cre;Ptprsfl/fl mice (2 months) in the elevated plus-maze, as supported by decreased close-arm time and increased open-arm time. (n = 16 [WT] and 16 [cKO], *p<0.05, **p<0.01, ns, not significant, Student’s t-test, Mann-Whitney test [time in open arms]). (F) Normal self-grooming and rearing in Emx1-Cre;Ptprsfl/fl mice (2 months) in home cages. (n = 15 [WT] and 14 [cKO], in rearing, 15, 16 mice for WT, cKO, ns, not significant, Student’s t-test). (G) Normal prepulse inhibition in Emx1-Cre;Ptprsfl/fl mice (3 months). (n = 9 [WT] and 13 [cKO], ns, not significant, two-way ANOVA).
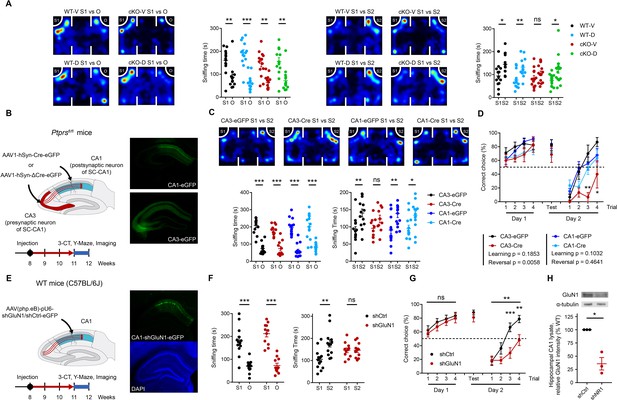
Presynaptic PTPσ-dependent regulation of postsynaptic NMDARs is important for novelty recognition in Emx1-Cre;Ptprsfl/fl mice.
(A) D-cycloserine (DCS) treatment (20 mg/kg; i.p.) rescues social-novelty recognition in Emx1-Cre;Ptprsfl/fl mice (2–3 months) without affecting social approach. Note that DCS has no effect on social approach or novelty recognition in WT mice. (n = 13, 14, 14, 14 mice for WT-V/vehicle, WT-D/D-cycloserine, cKO-V, cKO-D, *p<0.05, **p<0.01, ***p<0.001, ns, not significant, two-way ANOVA with Sidak’s test). (B and C) Impaired social-novelty recognition in Ptprsfl/fl mice injected with AAV1-hSyn-Cre-eGFP in the CA3 region, but not the CA1 region, in the three-chamber test compared with control Ptprsfl/fl mice injected with AAV1-hSyn-ΔCre-eGFP in CA3 or CA1 regions. Note that social approach (left panel) is unaffected by either CA3- or CA1-specific PTPσ KO. EGFP fluorescence indicates virus injection sites in CA3/CA1 regions. (n = 13, 11, 15, 14 mice for CA3-EGFP, CA3-Cre, CA1-EGFP and CA1-Cre, respectively, *p<0.05, **p<0.01, ***p<0.001, ns, not significant, two-way ANOVA with Sidak’s test). (D) Impaired recognition of novel, but not initial, reward-arm location in Ptprsfl/fl mice injected with AAV1-hSyn-Cre-eGFP in the CA3, but not CA1, region in the Y-maze test (3 months), compared with control Ptprsfl/fl mice injected with AAV1-hSyn-ΔCre-eGFP. (n = 9, 7, 16, 17 mice for CA3-EGFP, CA3-Cre, CA1-EGFP and CA1-Cre, respectively, during learning phase, n = 6, 3, 13, 11 mice for reversal phase, two-way ANOVA with Sidak’s test) (E and F) Knockdown of the GluN1 subunit of NMDARs in the CA1 region of WT mice (C57/BL6J) by injection of AAV(php.eB)-pU6-shGluN1 suppresses social-novelty recognition but not social approach, a finding that contrasts with the normal social-novelty recognition observed in control WT mice injected with AAV(php.eB)-pU6-shCtrl (scrambled control). (n = 13, 11 mice for shCtrl and shGluN1, respectively, **p<0.01, ***p<0.001, ns, not significant, two-way ANOVA with Sidak’s test). (G) GluN1 knockdown in the CA1 region of WT mice (C57/BL6J) by injection of AAV(php.eB)-pU6-shGluN1 suppresses novel, but not initial, reward-arm recognition compared with control WT mice injected with AAV(php.eB)-pU6-shCtrl. (n = 17 [shGluN1-initial], 19 [shCtrl-initial], 15 [shGluN1-reversal], 15 [shCtrl-reversal], **p<0.01, ***p<0.001, ns, not significant, RM two-way ANOVA with Sidak’s test). (H) Validation of AAV(php.eB)-pU6-shGluN1/shCtrl viruses by immunoblot analysis of the GluN1 protein from the injected hippocampus. GluN1 levels were normalized to α-tubulin levels. (n = 3, three mice for ShCtrl and shGluN1, *p<0.05, one sample t-test).
Tables
Reagent type (species) or resource | Designation | Source or reference | Identifiers | Additional information |
---|---|---|---|---|
Gene (Mus musculus) | Ptprs | 19280 in ncbi | For iteration of next studies. | |
Strain, strain background (Mus musculus; C57BL/6J) | Emx1-Cre;Ptprsfl/fl;Ptprs–l– mice | ES-Cell from KOMP: Ptrpstm1a(KOMP)Mbp | RRID: MGI_5797751 | gKO/cKO mice used in this study |
Genetic reagent (Mus musculus) | Emx1-Cre | JAX | #005628 | Cre-expressing line used in this study |
Transfected virus (Mus musculus) | pAAV1-hSyn-Cre-eGFP | Addgene | #105540 | Experimental virus for Cre injection (Figure 2 and Figure 6) |
Transfected virus (Mus musculus) | pAAV1-hSyn-ΔCre-eGFP | Addgene | #105539 | Control virus for Cre injection (Figure 2 and Figure 6) |
Transfected construct (Mus musculus) | pAAV-nEFCas9 | Addgene | #87115 | Vector for WT/Mut Ptprs expression (Figure 3). |
Transfected construct (Mus musculus) | Ptprs WT gene | Li et al., 2015 | Ptprs(meA-/meB-) | Detailed sequence is added in Supplementary file 1. This used for mutagenesis and WT Ptprs rescue injection |
Transfected construct (Mus musculus) | pAV-pU6-shGluN1#1-GFP | Vigene | #SH836303 | Experimental virus for Cre injection (Figure 6) |
Transfected construct (Mus musculus) | pAV-pU6-shscrambled-GFP | Vigene | #SH836303 | Control virus for Cre injection (Figure 6) |
Comparative phosphor-proteomic analysis | PhosphoSCAN service | Cell Signalling Technology | Phospho-tyrosine(pY-1000) | Used in Figure 4 |
Antibody | anti-PTPσ (Guinea Pig polyclonal) | This paper | #2135 for N-term epitope, #2138 for C-term epitope | WB(1:500) |
Antibody | anti-PSD93/SynGAP1/NGL3/SALM5/GluA1); (Rabbit,mouse polyclonal) | Home-made; used in previous studies from our group. | #1634(PSD93);#1682(SynGAP1);#2020(NGL3)#1943(SALM50;#1193(GluA1) | WB(1:500) |
Antibody | anti-tubulin (mouse monoclonal) | DSHB | 12G10 | WB(1:5000) |
Antibody | anti-GluN1(mouse))/GluN2A(rabbit)) monoclonal | Millipore | Mab363(GluN1);07-632(GluN2A) | WB(1:500) |
Antibody | anti-GluN2B(mouse)/PSD95(mouse) monoclonal | NeuromAb | 73-101(GluN2B);75-028(PSD95) | WB(1:500) |
Antibody | anti-Shank3(Rabbit;poly)/Synaptophysin(Mouse;mono) | Santa Cruz | H160(Shank3);D4(Synaptophysin) | WB(1:500) |
Antibody | anti-linprinα3/caskin1 (Rabbit polyclonal) | Synaptic Systems | 169 102(Liprin α3); 185 003(caskin1) | WB(1:500) |
Antibody | anti-liprinα2(Rabbit)/Trio(Mouse) polyclonal | Abcam | Ab155411(liprinα2);194365(Trio) | WB(1:500) |
Antibody | anti-βcatenin(Mouse monoclonal) | BD Science | 610154 | WB(1:500) |
Antibody | anti-N-Cadherin (Mouse monoclonal) | Thermo | 33–3900 | WB(1:500) |
Antibody | anti-TrkC (Rabbit monoclonal) | Cell Signaling Technology | 3376 | WB(1:500) |
Software, algorithm | GraphPad Prism 7.0 | GraphPad | Ver 7.0 | Used for all statistics used in the current study. |
Software, algorithm | DAVID analysis | David.ncifcrf.gov | DAVID analysis | Used for statistics in proteomics |
Additional files
-
Supplementary file 1
Amino acid sequences of PTPσ mutants used in the present study.
- https://cdn.elifesciences.org/articles/54224/elife-54224-supp1-v2.docx
-
Supplementary file 2
Details of the results of pTyr analysis.
- https://cdn.elifesciences.org/articles/54224/elife-54224-supp2-v2.xlsx
-
Supplementary file 3
Statistical details.
- https://cdn.elifesciences.org/articles/54224/elife-54224-supp3-v2.xlsx
-
Transparent reporting form
- https://cdn.elifesciences.org/articles/54224/elife-54224-transrepform-v2.docx