Serotonin signaling by maternal neurons upon stress ensures progeny survival
Figures
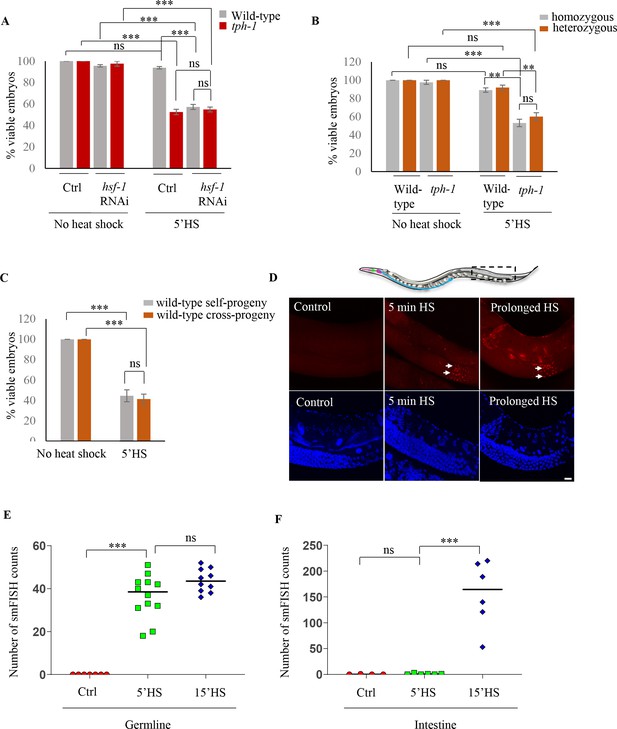
Maternal serotonin protects the viability of offspring following heat shock.
(A) Percent viable embryos from control (No heat-shock) and heat-shocked (5’HS) wild-type and tph-1 mutant animals under control (Ctrl) conditions and subjected to hsf-1 RNAi. Embryos were laid during a 2 hr interval by non-heat shocked animals, or animals that were heat-shocked for 5 min at 34°C and allowed to recover at 20°C for 2 hr. Wild-type animals (n = 28 experiments, embryos from 4 to 5 animals/experiment), tph-1 mutant animals (n = 28 experiments, embryos from 4 to 5 animals/experiment), wild-type hsf-1 RNAi-treated animals (n = 8 control and 24 heat-shock experiments, embryos from 4 to 5 animals/experiment), and tph-1 hsf-1 RNAi-treated animals (n = 3 control and 9 heat-shock experiments, embryos from 4 to 5 animals/experiment). Percent viable embryos from control non-heat shocked animals on OP50 are shown here and do not differ from those from animals on Control RNAi (n = 3–8 experiments; control wild-type = 99.5 ± 0.4, control tph-1 = 98.8 ± 0.6). (B) Percent viable homozygous or heterozygous embryos from control (No heat shock) and heat-shocked (5’HS) wild-type and tph-1 mutant animals. Wild-type and tph-1 mutant hermaphrodites were allowed to mate with wild-type males and once the hermaphrodites were laying cross- progeny, embryos laid during a 2 hr interval by non-heat-shocked animals, or animals that had recovered at 20°C for 2 hr post-5 minutes 34°C heat-shock were scored. n = 4 experiments, embryos from 4 to 5 animals/experiment. (C) Percent viable embryos that were either self- or cross-progeny, laid by control (No heat shock) wild-type animals, or wild-type animals heat-shocked for 5 min at 34°C (5’HS). Wild-type hermaphrodites were allowed to mate with wild-type males that had been heat-shocked for 5 min at 34°C, and once the hermaphrodites were laying cross-progeny, the hermaphrodites were heat-shocked for 5 min at 34°C, and viable embryos laid 0–2 hr post-heat shock were scored (n = 5 experiments, embryos from 4 to 5 animals/experiment). (D) Representative confocal image showing hsp70 (F44E5.4/.5) mRNA localization using smFISH in wild-type animals under control conditions and upon 5 min and 15 min (prolonged HS) exposure to 34°C (n = 3 experiments, 24 animals). Optical sections were projected on one plane. Top, red: hsp70 (F44E5.4/.5) mRNA. Bottom, blue: DAPI staining nuclei. Arrows indicate hsp mRNA in germline cells and arrowhead, in intestinal cells. Scale bar = 10 µm. (E) smFISH counts in the late pachytene area of the germline of control animals (Ctrl; mean count = 0 ± 0, n = 7), animals subjected to 5 min heat shock (5’HS; mean count = 36.4 ± 2.9, n = 12), and animals subjected to 15 min heat shock (15’HS; mean count = 43.8 ± 1.7, n = 10). (F) smFISH counts in the intestine of control animals (Ctrl; mean count = 0.25 ± 0.25, n = 4), animals subjected to 5 min heat shock (5’HS; mean count = 0.83 ± 0.48, n = 6), and animals subjected to 15 min heat shock (15’HS; mean count = 156.2 ± 26.2, n = 6). Data in A, B, C, show Mean ± Standard Error of the Mean. Data in E, F) show individual values. ***, p<0.001; **p<0.01, *p<0.05 (paired Student’s t-test). ns, non-significant.
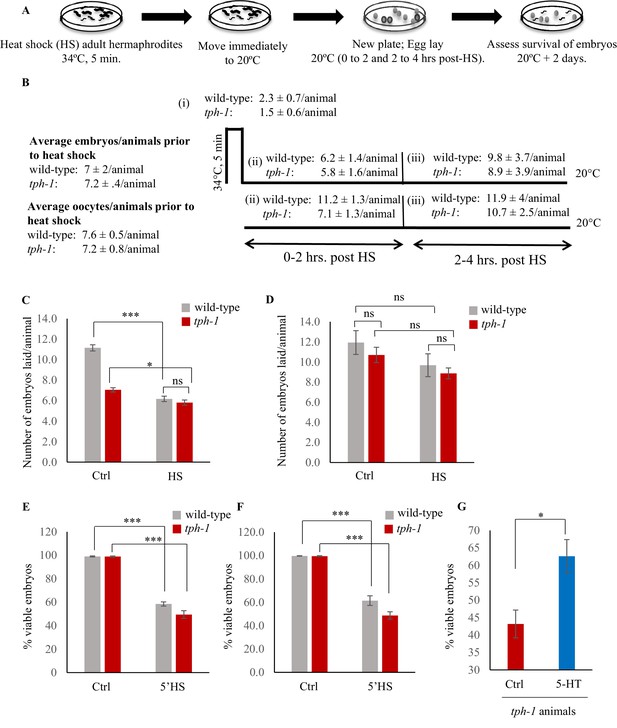
Characterizing the effects of heat on viability of embryos.
(A) Schematic design of the experiment. (B) The average numbers of embryos and oocytes present per animal in wild-type animals and tph-1 mutants prior to heat exposure, and embryos laid per animal under control conditions at 20°C, (i), upon the 5 min exposure to 34°C, (ii), during 0–2 hr post-heat shock, and (iii) during 2–4 hr post-heat shock are shown. (C) Mean numbers of embryos laid per animal by wild-type animals and tph-1 mutants under control conditions at 20°C, and during 0–2 hr post-heat shock (n = 18 experiments). (D) Mean numbers of embryos laid per animal by wild-type animals and tph-1 mutants under control conditions, and 2–4 hr post-heat shock. (n = 28 experiments). (E) Percent viable embryos when embryos laid by wild-type animals and tph-1 mutant animals during a 2 hr interval were exposed to 34°C for 5 min. (F) Percent viable embryos when embryos were present in utero in wild-type animals or tph-1 mutant animals exposed to 34°C for 5 min. This was determined by assaying the viability of embryos laid during a 2 hr interval immediately after the mothers were heat-shocked. (G) Percent viable embryos laid by tph-1 mutant animals upon treatment with exogenous 5-HT compared to those laid by untreated tph-1 mutant animals. In this experiment embryos were laid by tph-1 animals 2–4 hr after a 5-min heat shock. Data in C–G show Mean ± Standard Error of the Mean. *, p<0.05; **, p<0.01 ***, p<0.001, ns = non significant; (Paired Student’s t-test).
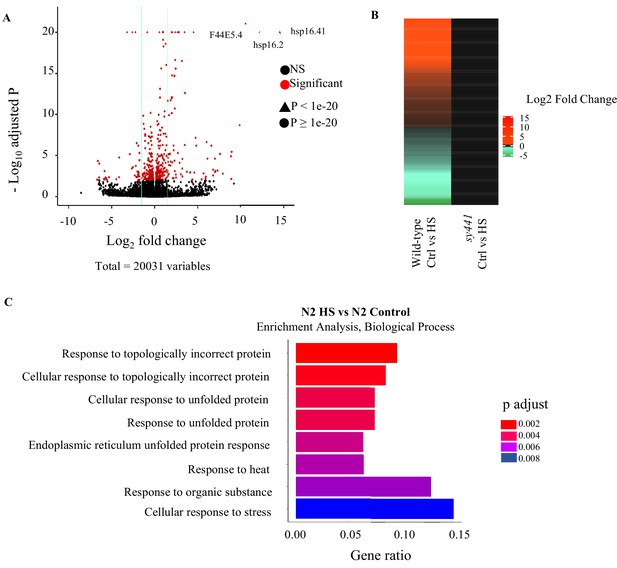
A brief (5 min) heat-shock induces HSF-1-dependent gene expression.
(A) Volcano plot from RNA-seq data showing differentially expressed transcripts (Log2 fold change) in wild-type animals following 5-min heat shock at 34°C compared to control, wild-type animals. Genes differentially expressed at 0.01 FDR are in red. (B) Heat map showing differential gene expression in hsf-1(sy441) I mutants (Log2 fold change) that lack functional HSF-1 upon heat shock, compared to differential gene expression in wild-type animals exposed to the same conditions. Heat shock: 5-min heat shock at 34°C. (C) Gene Ontology analysis (Biological Processes) of differentially expressed transcripts in wild-type animals following a 5-min heat shock at 34°C.
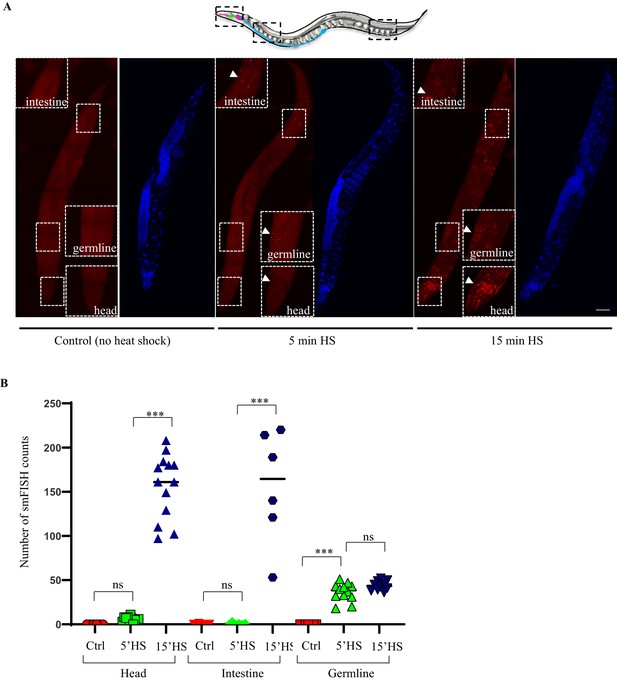
Characterization of hsp70 (F44E5.4/.5) gene expression upon heat shock.
(A) Representative micrographs showing projections of confocal images of wild-type animals processed for hsp70 (F44E5.4/.5) mRNA localization using smFISH. hsp70 (F44E5.4/.5) mRNA localization under control conditions and upon 5 min and 15 min exposure to 34°C (n = 5 experiments, 33 animals). Red.: hsp70 (F44E5.4/.5) mRNA. Blue: DAPI stained nuclei. Arrows indicate hsp mRNA. Insets correspond to specific regions within the animal. Scale bar = 10 µm. (B) smFISH counts in the head region, first two cells of the intestine, and the pachytene region of the germline of control animals (Ctrl), animals subjected to 5-min heat shock (5’HS), and animals subjected to 15-min heat shock (15’HS). Mean ± standard error of smFISH counts for head: Ctrl = 0 ± 0, n = 6, 5’HS = 5 ± 1.1, n = 11, 15’HS = 156.5 ± 10.2, n = 13. Mean ± standard error of smFISH counts for intestine: Ctrl = 0.25 ± 0.25, n = 4, 5’HS = 0.83 ± 0.48, n = 6, 15’HS = 156.2 ± 26.2, n = 6. Mean ± standard error of smFISH counts for germline: Ctrl = 0 ± 0, n = 6, 5’HS = 36.4 ± 2.9, n = 11, 15’HS = 43.8 ± 1.7, n = 10. Data in B shows individual counts/animal. ***, p<0.001; **p<0.01, *p<0.05 (paired Student’s t-test). ns, non-significant.
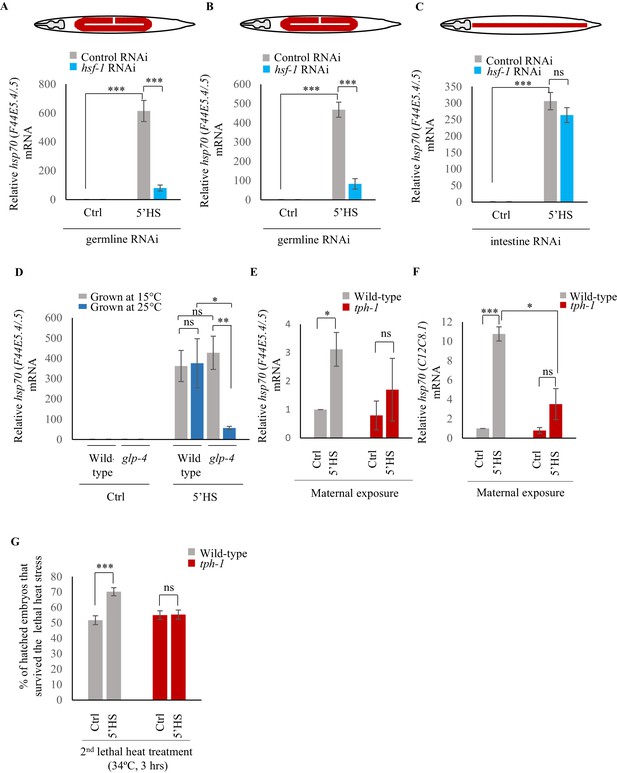
Maternal serotonin enables protective gene expression in the germline.
(A–C) Average hsp70 (F44E5.4/.5) mRNA levels in control, non heat-shocked (Ctrl) and heat-shocked (5’HS) animals subjected to tissue specific RNAi. mRNA levels were normalized to that in control non-heat shocked, wild-type animals. Heat Shock: 5 min at 34°C. (A) rrf-1 (pk1714) animals that predominantly undergo RNAi in germline tissue were subjected to control RNAi and hsf-1 RNAi (n = 4 experiments). (B) mkcSi13 [sun-1p::rde-1::sun-1 3′UTR + unc-119(+)] II; rde-1(mkc36) V animals that predominantly undergo RNAi in germline tissue subjected to control RNAi and hsf-1 RNAi (n = 3 experiments). (C) rde-1(ne219) V; kbIs7 predominantly undergo RNAi in intestinal tissue subjected to control RNAi and hsf-1 RNAi (n = 3 experiments). (D) Average hsp70 (F44E5.4/.5) mRNA levels in control non heat-shocked (Ctrl) and heat-shocked (5’HS) wild-type and glp-4 (bn2) I animals raised at 15°C (permissive temperature for glp-4) or 25°C (restrictive temperature for glp-4). n = 4 experiments. mRNA levels were normalized to that in control non-heat shocked animals of same genetic background, raised at the corresponding temperature. Heat Shock: 5 min at 34°C. (E) Average hsp70 (F44E5.4/.5) mRNA levels in embryos laid during a 2 hr interval by wild-type or tph-1 mutant animals that were not heat shocked (Ctrl), or heat-shocked for 5 min at 34°C and allowed to recover for 2 hr (5’HS). n = 3 experiments, embryos laid from 50 animals/experiment. mRNA levels were normalized to that in control non-heat shocked, wild-type embryos. (F) Average hsp70 (C12C8.1) mRNA levels in embryos laid during a 2 hr interval by wild-type or tph-1 mutant animals that were not-heat shocked (Ctrl), or heat-shocked for 5 min at 34°C and allowed to recover for 2 hr (5’HS). n = 3 experiments, embryos laid from 50 animals/experiment. mRNA levels were normalized to that in control non-heat shocked, wild-type embryos. (G) Percent larvae from non-heat shocked, or heat-shocked wild-type and tph-1 mutant animals that survive a subsequent heat exposure to 34°C. Maternal heat shock: 5 min at 34°C. Larval heat shock: 3 hr at 34°C. Note: larval heat exposure was titrated to achieve ~50% lethality amongst progeny of control, non-heat shocked animals. n = 5 experiments; larvae derived from 4 to 5 adult animals/experiment were scored. Data show Mean ± Standard Error of the Mean. ***, p<0.001; **p<0.01, *p<0.05 (paired Student’s t-test). ns, non-significant.
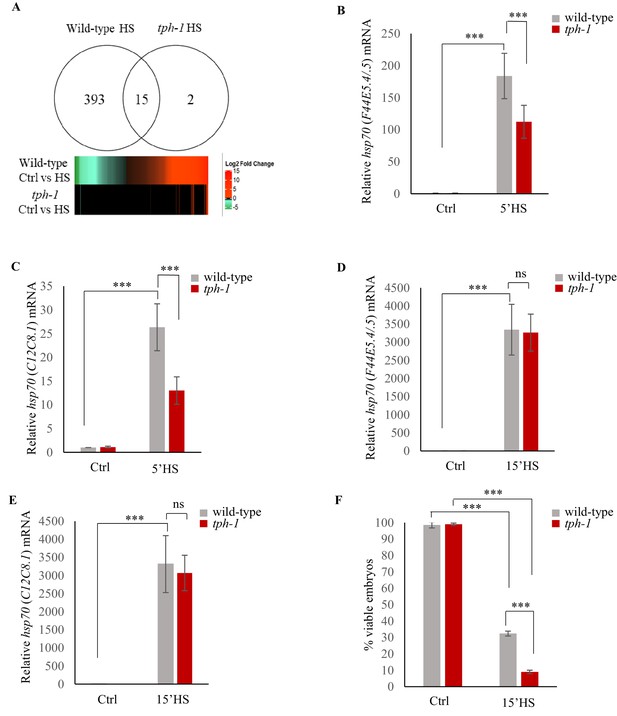
Serotonin accelerates the onset of gene expression upon heat shock.
(A) Top: Venn diagram showing overlap between genes differentially expressed in wild-type animals and tph-1 mutants (0.01 FDR) following 5 min heat-shock at 34°C. Numbers depict differentially induced genes in each strain. Data from RNA-seq experiments. Bottom: Heat map depicting expression levels (Log2 Fold change) of differentially expressed genes in wild-type and tph-1 mutants. (B–E) Average hsp70 mRNA in wild-type and tph-1 mutant animals following heat shock: (B) hsp70 (F44E5.4/.5) mRNA and (C) hsp70 (C12C8.1) mRNA levels in wild-type and tph-1 mutant animals under control conditions and following heat shock at 34°C for 5 min (n = 22 experiments). (D) hsp70 (F44E5.4/.5) mRNA and (E) hsp70 (C12C8.1) mRNA levels in wild-type and tph-1 mutant animals under control conditions and following heat shock at 34°C for 15 min (n = 5 experiments). (B–E) mRNA levels were normalized to that in control non-heat shocked, wild-type animals. (F) Percent viable embryos from control (Ctrl) and heat-shocked (15’HS), wild-type animals and tph-1 mutant animals. Embryos were laid during a 2 hr interval by non-heat shocked animals, or animals that were heat-shocked for 15 min at 34°C and allowed to recover at 20°C for 2 hr. n = 4 experiments, 4–5 animals/experiment. Data in B–F show Mean ± Standard Error of the Mean. ***, p<0.001 (paired Student’s t-test). ns, non-significant.
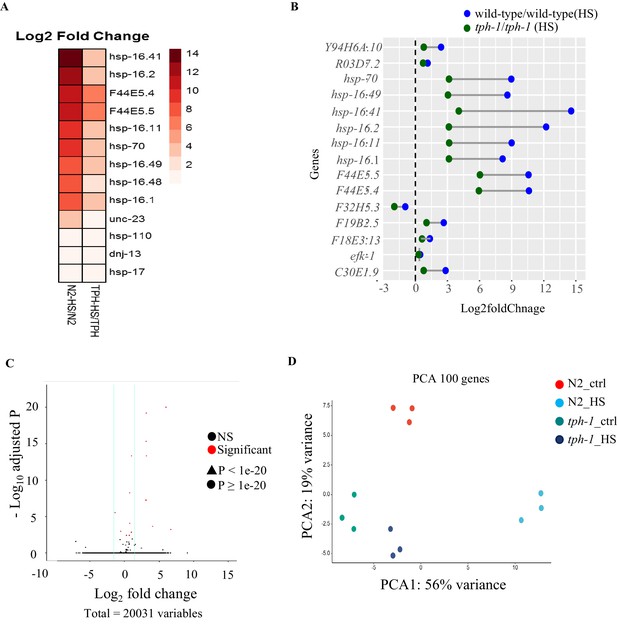
The lack of serotonin diminishes HSF-1-dependent gene expression upon 5 min heat-shock.
(A) Heat map depicting expression levels (Log2 fold change) of thirteen differentially expressed hsp genes in wild-type and tph-1 mutants. (B) Dumb-bell graph showing the expression levels of the 15 differentially expressed genes that are common between wild-type and tph-1 heat shock response. Heat shock: 5 min heat shock at 34°C. (C) Volcano plot from RNA-seq data showing differentially expressed transcripts (Log2 fold change) in tph-1 mutant animals following 5 min heat shock at 34°C compared to control, tph-1 animals. Compare with Figure 1—figure supplement 2A (D) Principal Component Analysis (PCA) depicting the separation of wild-type animals exposed for 5 min to 34°C from control non-heat shocked animals, and the lack of similar separation in tph-1 mutant animals.
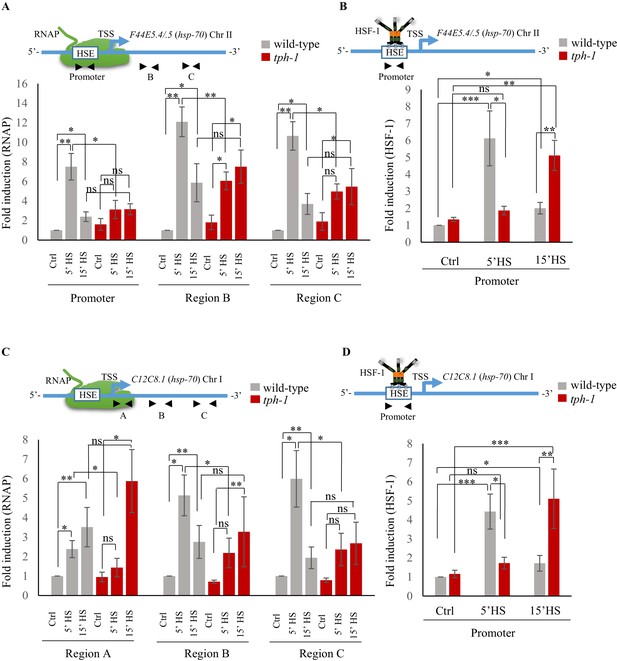
Serotonin accelerates the onset of RNAP and HSF-1 recruitment to target genes.
(A) Top: Schematic of hsp70 (F44E5.4/.5) gene regions within the Promoter (−390 to −241), middle of gene (Region B: +696 to +915) and toward 3’-UTR (Region C: +1827 to +1996) that were assayed for occupancy by RNAP. Bottom: RNAP occupancy at Promoter, Region B and Region C in wild-type animals and tph-1 mutants following exposure to 34°C for 5 min and 15 min (n = 8 experiments). (B) Top: Schematic of hsp70 (F44E5.4/.5) gene regions within the Promoter (−390 to −241) assayed for occupancy of HSF-1. This is the same Promoter region as in A. Bottom: HSF-1 occupancy at the Promoter in wild-type animals and tph-1 mutants following exposure to 34°C for 5 min and 15 min (n = 14 experiments). (C) Top: Schematic of hsp70 (C12C8.1) gene regions close to the beginning (Region A: +25 to +185), middle of gene (Region B: +475 to +583) and towards 3’-UTR (Region C:+1645 to +1835) assayed for occupancy by RNAP. Bottom: RNAP occupancy at Region A, Region B and Region C in wild-type animals and tph-1 mutants following exposure to 34°C for 5 min and 15 min (n = 8 experiments). (D) Top: Schematic of hsp70 (C12C8.1) gene region within the Promoter (−166 to −78) assayed for HSF-1 occupancy. Bottom: HSF-1 occupancy at the Promoter in wild-type animals and tph-1 mutants following exposure to 34°C for 5 min and 15 min (n = 14 experiments). Data show Mean ± Standard Error of the Mean. Data in all experiments are normalized to values from control (non-heat shocked) wild-type animals. Specificity and efficiency of pull-down under control conditions was ascertained (see Figure 4—figure supplement 2A, (B). *, p<0.05; **, p<0.01 ***, p<0.001; (ANOVA with Tukey’s correction). ns, non-significant.
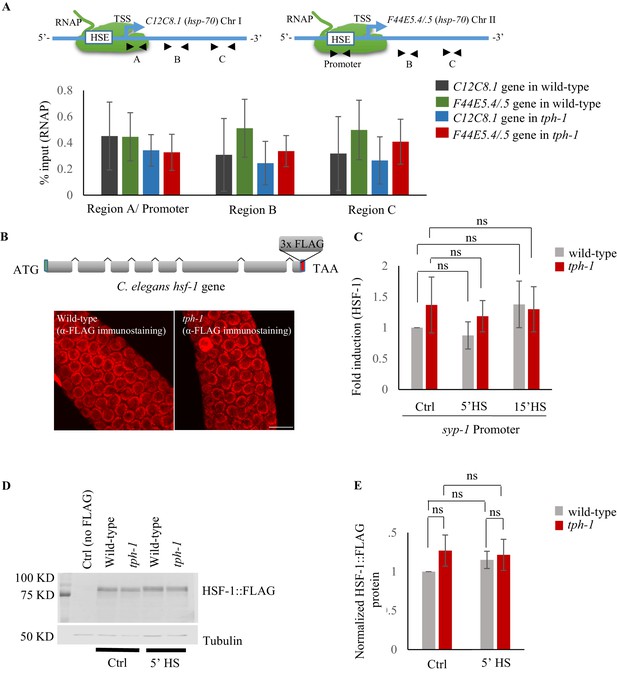
Characterization of RNAP and HSF-1 for ChIP-qPCR assays.
(A) Top: ChIP-qPCR to measure RNAP occupancy: Schematic of hsp70 (F44E5.4/.5) gene regions within the Promoter (−390 to −241), middle of gene (Region B: +696 to +915) and towards 3’-UTR (Region C: +1827 to +1996) assayed for occupancy by RNAP. Schematic of hsp70 (C12C8.1) gene regions close to the beginning (Region A: +25 to +185), middle of gene (Region B: +475 to +583) and towards 3’-UTR (Region C:+1645 to +1835) assayed for occupancy by RNAP. Bottom: RNAP occupancy at these regions in control non-heat shocked wild-type and tph-1 mutant animals. RNAP (% input) at the Region A/Promoter region in control wild-type animals does not significantly differ from that at Region B or Region (C) as would have been expected if RNAP was paused near the TSS (n = 8 experiments). Data show Mean ± Standard Error of the Mean. Comparisons are non-significant. ANOVA with Tukey’s correction. (B) Top: Schematic of hsf-1 tagged at the endogenous locus with 3X FLAG using CRISPR/Cas9. Bottom: Representative micrographs of confocal sections of the germline region showing HSF-1 immunostaining with anti-FLAG antibody in wild-type and tph-1 mutants (n = 5 experiments). Note HSF-1 is expressed in germline cells in both wild-type animals and tph-1 mutant animals. Scale bar = 10 µm. (C) Fold change in HSF-1 occupancy at the promoter of a control gene not having any HSF-1 binding sites (syp-1) in wild-type animals and tph-1 mutants following 5 and 15 min at 34°C. % input values were normalized to that in control wild-type animals (n = 14 experiments). (D) Representative western blot using anti-FLAG antibody showing the specificity of the FLAG antibody (lane 1: Ctrl are wild-type animals that do not express FLAG-tagged proteins), and HSF-1 levels in wild-type and tph-1 animals under control and heat shock conditions. Tubulin was used as a loading control. (E) Quantified HSF-1 levels in wild-type and tph-1 animals under control and heat shock conditions (n = 3 experiments). Protein levels are normalized to control wild-type animals. Note HSF-1 protein levels do not change. Data in A, C, E show Mean ± Standard Error of the Mean. (A and C), ANOVA with Tukey’s correction. (E) Paired Student’s t-test. ns, non-significant.
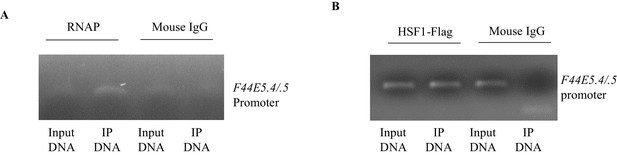
Validation of specificity and efficiency of antibodies used for ChIP assays.
(A–B) Representative agarose gels used to assess specificity and efficiency of immunoprecipitation (IP) and primer sequences used for ChIP-PCR. All amplified bands were the expected size and mouse control IgG did not yield detectable signals. Most importantly, we confirmed that the ChIP signal in control animals was detectable and real, to validate the calculation of normalized fold changes in our experiments. (A) IP from wild-type control animals using mouse antibody to total RNAP. Mouse IgG used as control. Primers at the Promoter region of hsp70 (F44E5.4/.5). (B) IP from wild-type control animals using mouse anti-FLAG antibody. Mouse IgG used as control. Primers at the Promoter region of hsp70 (F44E5.4/.5).
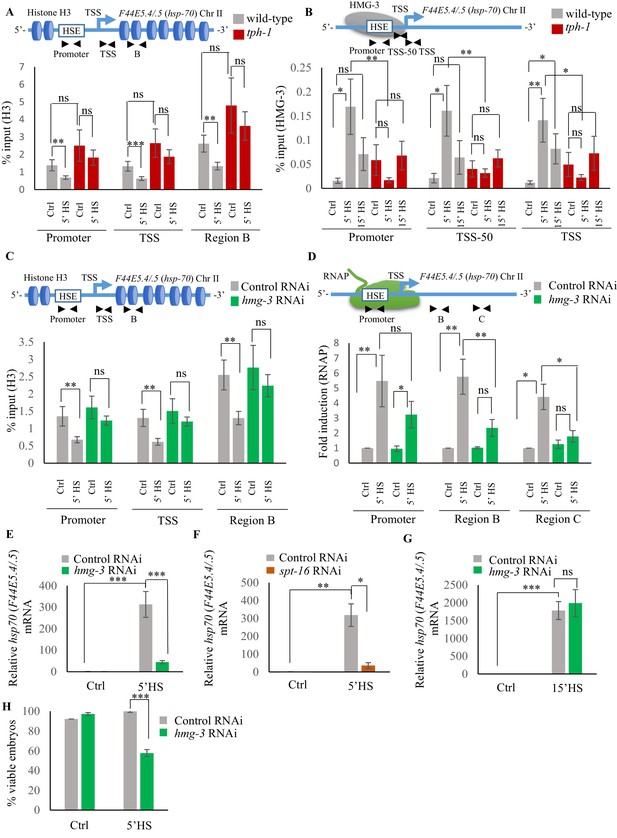
Serotonin enables FACT recruitment at hsp genes to displace nucleosomes and hasten the onset of transcription.
(A) Top: Schematic of hsp70 (F44E5.4/.5) gene regions within the Promoter (same as Figure 4A and B: −390 to −241), Transcription Start Site (TSS: −81 to +38) and gene body (same as Region B in Figure 4A: +696 to +915) assayed for histone H3 occupancy. Bottom: Occupancy of histone H3 (% input) at Promoter, TSS and Region B upon 5 min at 34°C (n = 9 experiments). (B) Top: Schematic of hsp70 (F44E5.4/.5) gene: regions in the Promoter (same as in Figure 4B: −390 to −241), region upstream of Transcription Start Site (TSS-50: −221 to −63) and Transcription Start Site (Same as A): TSS: −81 to +38) were assayed for HMG-3 occupancy. Bottom: HMG-3 occupancy (% input) across Promoter, TSS-50 and TSS following 5 and 15 min at 34°C (n = 13 experiments). (C) Top: Schematic of hsp70 (F44E5.4/.5) gene regions assayed for H3 occupancy (same as in A). Bottom: Occupancy of histone H3 in Promoter, TSS and in Region B of hsp70 (F44E5.4/.5) under control conditions and following 5 min at 34°C, in control-RNAi treated animals and hmg-3-RNAi treated animals (n = 9 experiments). (D) Top: Schematic of hsp70 (F44E5.4/.5) gene: same regions as in Figure 4A, that is Promoter (−390 to −241), Region B (+696 to +915) and Region C (+1827 to +1996) were assessed for RNAP occupancy in control-RNAi treated and hmg-3-RNAi treated animals. Bottom: Fold change in RNAP across Promoter, Region B and Region C following 5 min heat shock at 34°C (n = 5 experiments). % input values were normalized to that in control-RNAi treated animals at Promoter and Regions B and C. Specificity and efficiency of pull-down under control conditions were verified (see Figure 5—figure supplement 2A, (B). (E) hsp70 (F44E5.4/.5) mRNA levels in control-RNAi treated and hmg-3 -RNAi treated animals following a 5-min heat shock at 34°C (n = 6 experiments). (F) hsp70 (F44E5.4/.5) mRNA levels in control-RNAi treated and spt-16 -RNAi treated animals following a 5 min heat shock at 34°C (n = 4 experiments). (G) hsp70 (F44E5.4/.5) mRNA levels in control-RNAi treated and hmg-3 -RNAi treated animals following a 15 min heat shock at 34°C (n = 6 experiments) (H) Percent viable embryos (laid 2–4 hr post-5 min heat shock at 34°C) from control-RNAi treated and hmg-3-RNAi treated animals (n = 5 experiments, 4–5 animals/experiment). Data show Mean ± Standard Error of the Mean. *, p<0.05; **, p<0.01 ***, p<0.001; (A–D, ANOVA with Tukey’s correction; E–H) Paired Student’s t-test). ns, non-significant.
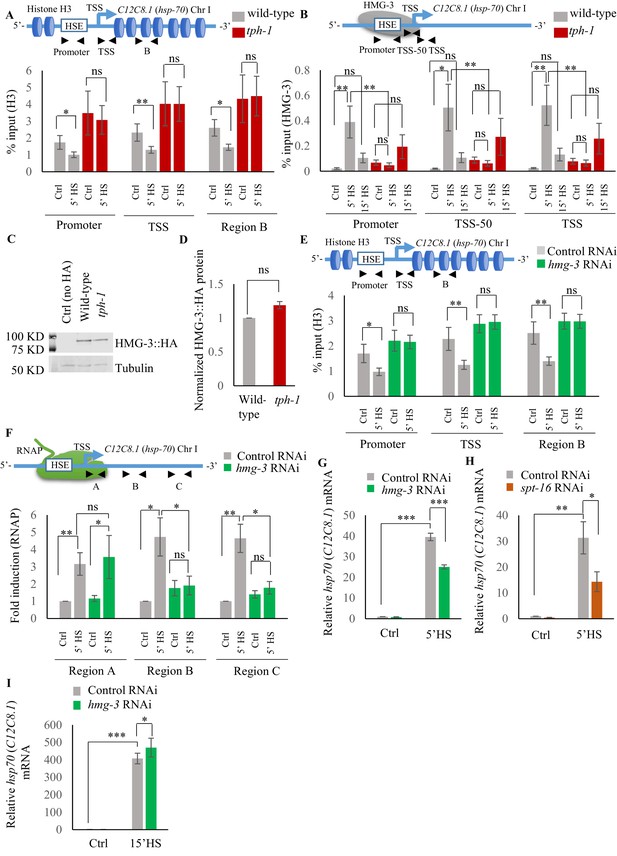
Characterizing H3 and FACT occupancy across hsp genes in wild-type and tph-1 mutant animals under control and heat shock conditions.
(A) Top: Schematic of hsp70 (C12C8.1) gene regions within the Promoter (same as in Figure 4D: −166 to −78), Transcription Start Site (TSS: +40 to +131) and gene body (Region B: +475 to +583) assayed for histone H3 occupancy. Bottom: Occupancy of histone H3 (% input) at the Promoter, TSS and Region B upon 5 min at 34°C (n = 9 experiments). (B) Top: Schematic of hsp70 (C12C8.1) gene regions within the Promoter (same as Figure 4D: −166 to –78), region upstream of Transcription Start Site (TSS-50: −77 to +42) and Transcription Start Site (TSS: +40 to +131) assayed for HMG-3 occupancy in wild-type and tph-1 mutant animals. Bottom: HMG-3 occupancy (% input) across Promoter, TSS-50 and TSS following 5 and 15 min at 34°C (n = 13 experiments). The specificity and efficiency of pull-down under control conditions was ascertained. (C) Representative western blot using anti-HA antibody showing the specificity of the HA antibody (lane 1: Ctrl are wild-type animals that do not express HA-tagged proteins), and HMG-3 protein levels in wild-type and tph-1 animals under control conditions. Tubulin was used as the internal control. (D) Quantified levels of HMG-3 in wild-type and tph-1 animals (n = 3 experiments). Protein levels were normalized to that in control animals. (E) Top: Schematic of hsp70 (C12C8.1) gene: same regions as in A were assayed for H3 occupancy. Bottom: Occupancy of histone H3 (% input) in Promoter, TSS and in Region B of hsp70 (C12C8.1) under control conditions and following 5 min at 34°C, in control-RNAi treated animals and hmg-3-RNAi treated animals (n = 9 experiments). (F) Top: Schematic of hsp70 (C12C8.1) gene: regions as in Figure 4C, that is close to the beginning (Region A: +25 to +185), middle of gene (Region B: +475 to +583) and towards 3’-UTR (Region C:+1645 to +1835) were assayed for occupancy by RNAP following hmg-3 knock-down by RNAi. Bottom: Fold change in RNAP at beginning, Region B and Region C following 5 min heat shock at 34°C (n = 5 experiments). % input values were normalized to that in control-RNAi treated animals at these regions. (G) hsp70 (C12C8.1) mRNA levels in control-RNAi treated and hmg-3 -RNAi treated animals following a 5 min heat shock at 34°C (n = 6 experiments). (H) hsp70 (C12C8.1) mRNA levels in control-RNAi treated and spt-16 -RNAi treated animals following a 5 min heat shock at 34°C (n = 4 experiments). (I) hsp70 (C12C8.1) mRNA levels in control-RNAi treated and hmg-3 -RNAi treated animals following a 15 min heat shock at 34°C (n = 4 experiments). Data show Mean ± Standard Error of the Mean. *, p<0.05; **, p<0.01 ***, p<0.001; (paired Students t-test). A, B, E, F: ANOVA with Tukey’s correction. (D) (G–I) paired Student’s t-test. ns, non-significant.
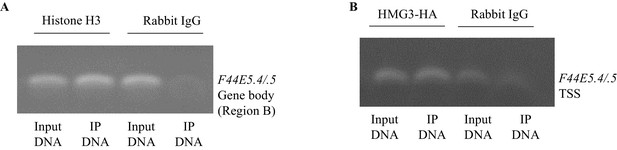
Validation of specificity and efficiency of antibodies used for ChIP assays.
(A–B) Representative agarose gels used to assess specificity and efficiency of immunoprecipitation (IP) and primer sequences used for ChIP-PCR. All amplified bands were the expected size and rabbit control IgG did not yield detectable signals. Most importantly, we confirmed that the ChIP signal in control animals was detectable and real, to validate the calculation of normalized fold changes in our experiments. (A) IP from wild-type control animals using rabbit antibody to histone H3. Rabbit IgG used as control. Primers in the Region B of hsp70 (F44E5.4/.5). (B) IP from wild-type control animals using rabbit anti-HA antibody. Rabbit IgG used as control. Primers at the TSS region of hsp70 (F44E5.4/.5).
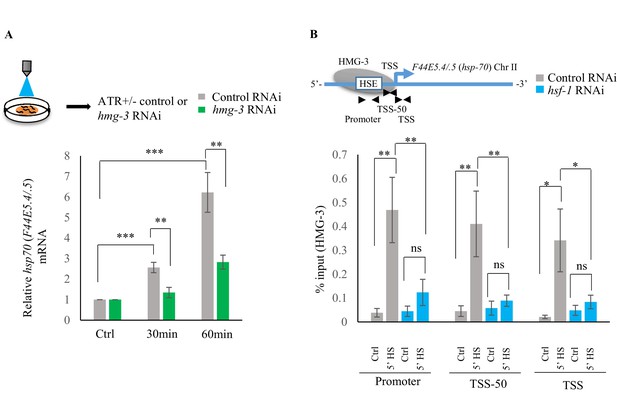
Serotonin release mediates FACT recruitment by HSF-1 to induce hsp expression.
(A) Top: Schematic of optogenetic activation of 5-HT release conducted by stimulating ADF and NSM neurons in control-RNAi treated and hmg-3-RNAi treated animals. Bottom: hsp70 (F44E5.4/.5) mRNA levels in control-RNAi treated and hmg-3-RNAi treated animals at different time points following optogenetic stimulation. mRNA levels were normalized to control-RNAi treated and hmg-3-RNAi treated unstimulated animals respectively (n = 6 experiments). (B) Top: Schematic of hsp70 (F44E5.4/.5) gene Promoter, TSS-50 and TSS to assess HMG-3 occupancy in control-RNAi treated and hsf-1-RNAi treated animals. Bottom: HMG-3 occupancy (% input) at Promoter, TSS-50 and TSS in control-RNAi and hsf-1 -RNAi treated animals following 5 min at 34°C (n = 9 experiments). Specificity and efficiency of pull-down under control conditions was ascertained. Data show Mean ± Standard Error of the Mean. *, p<0.05; **, p<0.01 ***, p<0.001. (A) Paired Student’s t-test. (B) ANOVA with Tukey’s correction). ns, non-significant.
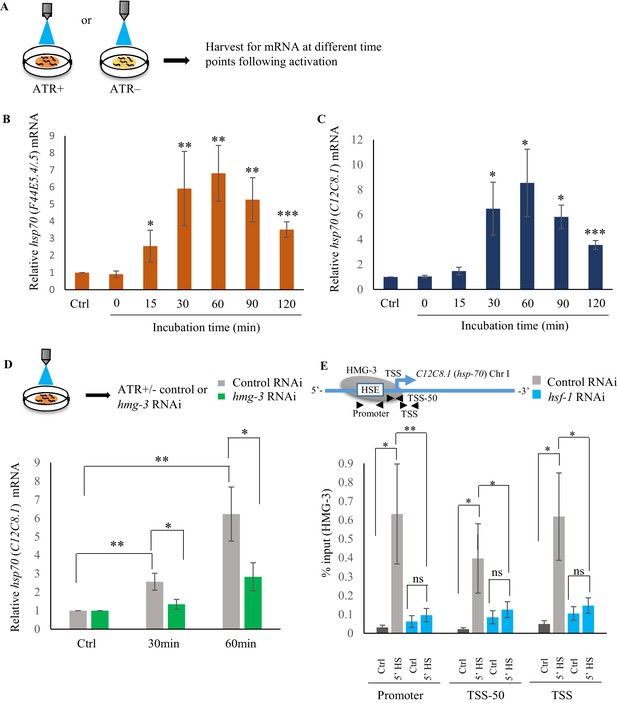
Serotonin-induced transcriptional activity of HSF-1 in C. elegans is FACT dependent.
(A) Schematic of optogenetic activation of 5-HT release by stimulating ADF and NSM neurons. (B, C) hsp70 (F44E5.4/.5) and hsp70 (C12C8.1) mRNA levels respectively, at different time points following optogenetic stimulation (n = 6 experiments). (D) Top: Schematic of optogenetic activation of 5-HT release by stimulating ADF and NSM neurons. Bottom: hsp70 (C12C8.1) mRNA levels in control-RNAi treated animals and animals treated with hmg-3-RNAi following optogenetic activation of 5-HT release. mRNA levels were normalized to control-RNAi treated and hmg-3-RNAi treated unstimulated animals (n = 6 experiments). (E) Top: Schematic of hsp70 (C12C8.1) gene showing Promoter, TSS-50 and TSS to assess HMG-3 occupancy in control-RNAi treated and hsf-1-RNAi treated animals. Bottom: HMG-3 occupancy (% input) across Promoter, TSS-50 and TSS in control-RNAi and hsf-1 -RNAi treated animals following 5 min at 34°C (n = 9 experiments). Specificity and efficiency of pull-down under control conditions was ascertained. Data show Mean ± Standard Error of the Mean. *, p<0.05; **, p<0.01 ***, p<0.001; (B–D) Paired Student’s t-test. (E) ANOVA with Tukey’s correction). ns, non-significant.
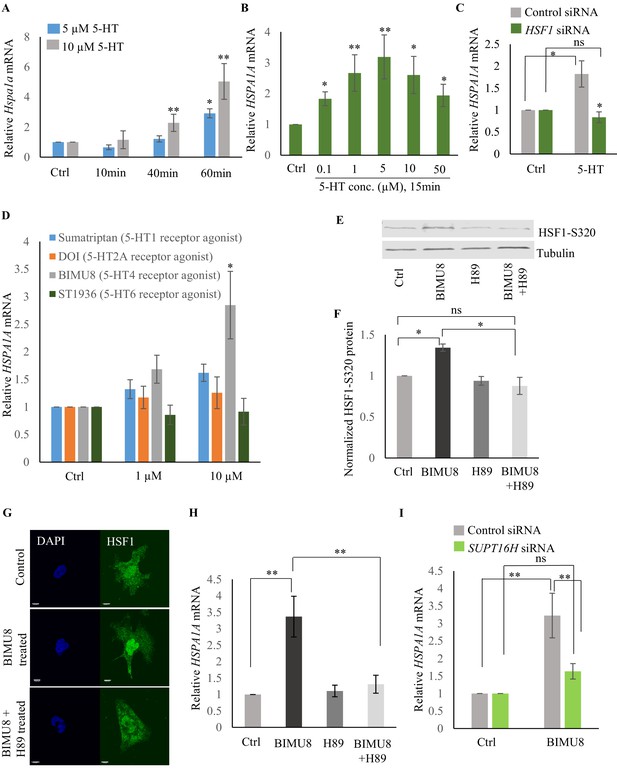
Serotonin activates PKA-mediated signal transduction to enable HSF1-FACT interaction in mammalian cells.
(A) Time and dose-dependent change in Hspa1a mRNA levels in control and 5-HT treated primary cortical neuronal cultures (n = 4 experiments). (B) Dose-dependent change in HSPA1A mRNA levels in control NT2 cells and NT2 cells treated with 5-HT for 15 min (n = 4 experiments). (C) HSPA1A mRNA levels in NT2 cells treated with 5 µM 5-HT for 15 min, transfected with control and HSF1 siRNA (n = 4 experiments). (D) HSPA1A mRNA levels in NT2 cells treated with two different doses of four 5-HT receptor agonists relative to control untreated cells (n = 5 experiments). NT2 cells were treated for 10 min. (E–F) Protein levels of S320 phospho-modified HSF1 in control NT2 cells and cells treated with 10 µM BIMU8 for 10 min, in the presence or absence of the PKA inhibitor, H89 (n = 4 experiments). (E) Representative western blot using an antibody that recognizes HSF1 phosphorylated at S320. Tubulin served as the internal control. (F) Quantitation of phospho-S320 levels (n = 4 experiments). (G) Representative micrographs showing projections of confocal images of HSF1 localization in control NT2 cells and cells treated with 10 µM BIMU8 for 10 min, in the presence or absence of the H89 (n = 2 experiments; 25 cells). Scale bar = 10 µm. (H) HSPA1A mRNA levels relative to control NT2 cells upon treatment with 10 µM BIMU8 for 10 min, in the presence or absence of H89 (n = 5 experiments). (I) HSPA1A mRNA levels in cells treated with 10 µM BIMU8 for 10 min, transfected with control and SUPT16H siRNA. mRNA levels and protein levels are normalized to control RNAi-treated or unstimulated cells (n = 5 experiments). Data in A-D, F, H, I show Mean ± Standard Error of the Mean. *, p<0.05; **, p<0.01 ***, p<0.001; (Paired Student’s t-test). ns, non-significant.
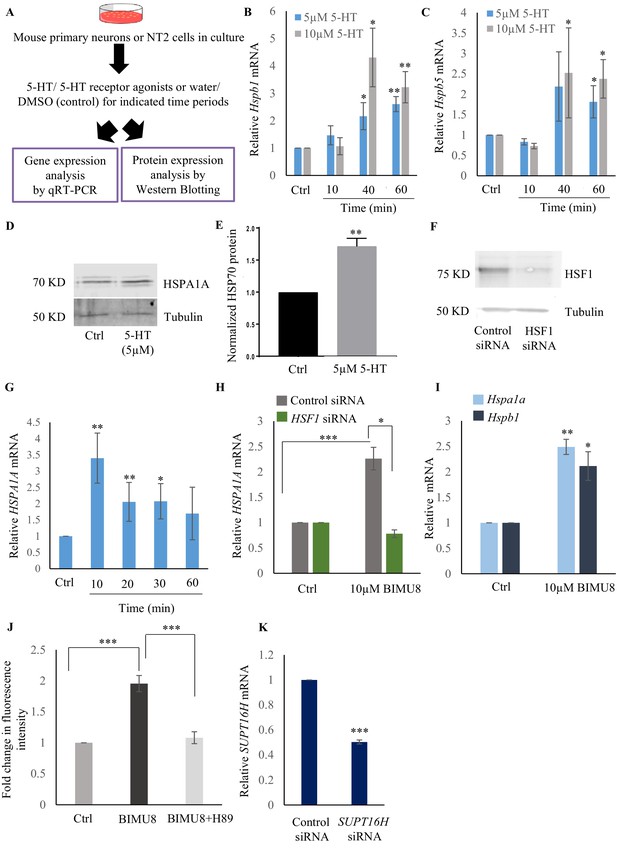
Serotonin activates a PKA-mediated signal transduction pathway to enable HSF1-FACT interaction in mammalian cells.
(A) Experimental setup for assessing whether 5-HT is a conserved signal that activates HSF1 in mammalian cells. (B, C) Time and dose-dependent change in Hspb1 and Hspb5 mRNA levels respectively, in control and 5-HT-treated primary cortical neuronal cultures (n = 4 experiments). (D) Representative western blot showing HSPA1A protein levels following 5-HT stimulation of NT2 cells (5 µM was applied for 24 hr to be able to assess protein accumulation; n = 4 experiments). (E) Quantitation of HSPA1A protein levels in control and 5-HT treated NT2 cells (n = 3 experiments). Tubulin was used as internal control. (F) Representative western blot showing HSF1 protein levels in control and HSF1 siRNA-treated NT2 cells to confirm siRNA knockdown of HSF1 (n = 2 experiments). (G) Temporal dynamics of HSPA1A mRNA levels in control and BIMU8-treated NT2 cells (n = 5 experiments). (H) HSPA1A mRNA levels in untreated and BIMU8-treated NT2 cells (10 µM for 10 min) transfected with control and HSF-1 siRNA (n = 4 experiments). (I) Hspa1 and Hspb1 mRNA levels in untreated and BIMU8-treated (10 µM for 10 min) primary cortical neuronal cultures (n = 3 experiments). (J) Quantitation of fluorescence intensity following immunostaining for HSF1 in the nuclei of untreated NT2 cells, NT2 cells treated with BIMU8 (10 µM for 10 min), and NT2 cells treated with BIMU8 and H89. Fluorescence intensity values (arbitrary units) were derived using ImageJ following background subtraction and normalized to that in control untreated cells. (n = 2 experiments; 25 cells each). (K) SUPT16H mRNA levels in control-siRNA and SUPT16H-siRNA treated NT2 cells to confirm siRNA knockdown of SUPT16H (n = 5 experiments). Data in B, C, E, G-K show Mean ± Standard Error of the Mean. Values were normalized to that in either control untreated cells, or control-siRNA treated cells. *, p<0.05; **, p<0.01 ***, p<0.001; (paired Students t-test).
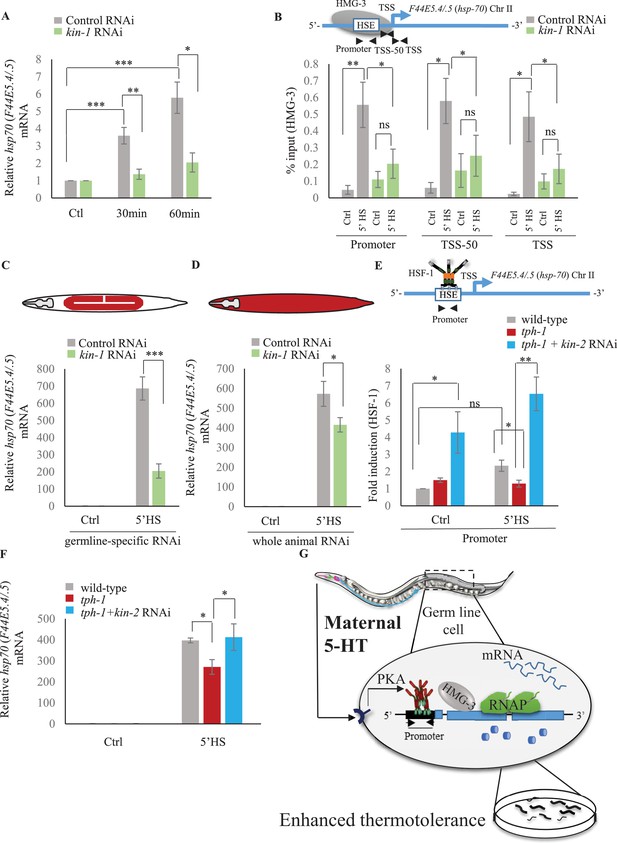
Serotonin-induced PKA-activation is a conserved signaling pathway that enables HSF-1 to recruit FACT in C. elegans.
(A) hsp70 (F44E5.4/.5) mRNA levels in control-RNAi treated and kin-1-RNAi treated animals at different time points following optogenetic stimulation of 5-HT release. mRNA levels are normalized to control RNAi and kin-1 RNAi treated unstimulated animals respectively (n = 8 experiments). (B) Top: Schematic of hsp70 (F44E5.4/.5) gene showing Promoter, TSS-50 and TSS region used to assess HMG-3 occupancy. Bottom: HMG-3 occupancy (% input) in wild-type animals subjected to control and kin-1 RNAi following 5 min at 34°C, across Promoter, TSS-50 and TSS regions (n = 9 experiments). Specificity and efficiency of pull-down under control conditions was ascertained. (C) hsp70 (F44E5.4/.5) mRNA levels in control and heat-shocked (mkcSi13 [sun-1p::rde-1::sun-1 3′UTR + unc-119(+)] II; rde-1(mkc36) V) animals that undergo germ-line specific RNAi following exposure to control RNAi or kin-1 RNAi. HS: 5 min at 34°C (n = 4 experiments). (D) hsp70 (F44E5.4/.5) mRNA levels in control and heat-shocked wild-type animals after they were subject to control and kin-1 RNAi-mediated knockdown. HS: 5 min at 34°C (n = 4 experiments). (E) Top: Schematic of hsp70 (F44E5.4/.5) promoter region assayed for HSF-1 occupancy. Bottom: HSF-1 occupancy in control and heat-shocked wild-type animals, tph-1 mutant animals and tph-1 mutant animals subjected to kin-2 -RNAi. HS: 5 min at 34°C (n = 5 experiments). % input values were normalized to that in control wild-type animals not subjected to heat shock. (F) hsp70 (F44E5.4/.5) mRNA levels in control and heat-shocked wild-type animals, tph-1 mutant animals and tph-1 mutant animals subjected to kin-2 -RNAi. HS: 5 min at 34°C (n = 5 experiments). Data show Mean ± Standard Error of the Mean. *, p<0.05; **, p<0.01 ***, p<0.001; ns, non-significant. (B, E) ANOVA with Tukey’s correction, A, C, D, F: paired Student’s t-test). (G) Working model showing how maternal 5-HT protects future progeny. 5-HT released by neurons permeates the animal through the coelomic fluid to act on the germline, activate the PKA signal transduction pathway in a tissue-autonomous manner and enable HSF-1 to recruit FACT, displace nucleosomes in the germline, and accelerate the onset of protective gene expression.
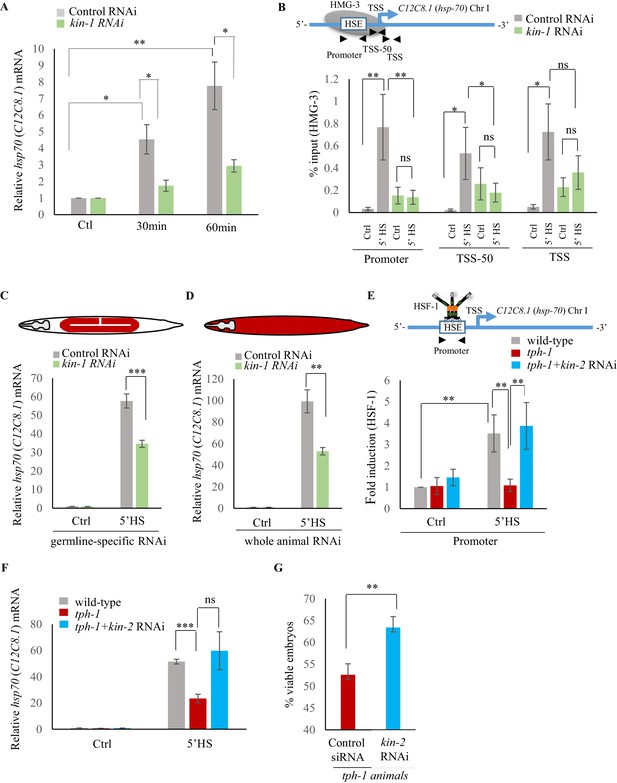
Serotonin-induced PKA-activation is a conserved pathway that enables HSF-1 to recruit FACT in C. elegans.
(A) hsp70 (C12C8.1) mRNA levels in control RNAi treated and kin-1 RNAi treated animals collected at different time points following optogenetic stimulation to release 5-HT. mRNA levels were normalized to control RNAi treated or kin-1 RNAi treated unstimulated animals (n = 8 experiments). (B) Top: Schematic of hsp70 (C12C8.1) gene showing Promoter, TSS-50 and TSS regions used to assess HMG-3 occupancy. Bottom: HMG-3 occupancy (% input) in wild-type animals subjected to control-RNAi and kin-1-RNAi following a 5-min heat shock treatment at 34°C (n = 9 experiments). (C) hsp70 (C12C8.1) mRNA levels in control and heat-shocked (mkcSi13 [sun-1p::rde-1::sun-1 3′UTR + unc-119(+)] II; rde-1(mkc36) V) animals that undergo germline specific RNAi; animals were subject to control and kin-1 RNAi-mediated knockdown. HS: 5 min at 34°C (n = 4 experiments). (D) hsp70 (C12C8.1) mRNA levels in control and heat-shocked wild-type animals after they were subject to control and kin-1 RNAi-mediated knockdown. HS: 5 min at 34°C (n = 4 experiments). (E) Top: Schematic of hsp70 (C12C8.1) promoter region assayed for HSF-1 occupancy. Bottom: HSF-1 occupancy at the hsp70 (C12C8.1) gene in control and heat-shocked wild-type animals, control and heat-shocked tph-1 mutant animals, and control and heat-shocked tph-1 mutant animals subjected to kin-2 -RNAi. HS: 5 min at 34°C (n = 5 experiments). % input values were normalized to that in control wild-type animals not subjected to heat shock. (F) hsp70 (C12C8.1) mRNA levels in control and heat-shocked wild-type animals, tph-1 mutant animals and tph-1 mutant animals subjected to kin-2 -RNAi. HS: 5 min at 34°C (n = 5 experiments). (G) Percent viable embryos laid 2–4 hr. post-heat shock by tph-1 animals and tph-1 animals subjected to kin-2 RNAi (n = 5 experiments). Data show Mean ± Standard Error of the Mean *, p<0.05; **, p<0.01 ***, p<0.001; (B, E) ANOVA with Tukey’s correction, A, C, D, F, G: paired Student’s t-test). ns, non-significant.
Tables
Reagent type (species) or resource | Designation | Source or reference | Identifiers | Additional information |
---|---|---|---|---|
Strain, strain background (Mus musculus) | Wild type C57BL/6 | Envigo | Cortical neuron preparations were made from P0 pups from timed-pregnant C57BL/6 mice | |
Genetic reagent (C. elegans) | N2 Wild type | Caenorhabditis Genetic Center | N2, C. elegans var Bristol | |
Genetic reagent (C. elegans) | hsf-1::3X flag I | Prahlad lab, University of Iowa | CRISPR insertion of 3X FLAG at C’ of hsf-1 | This paper (Materials and methods) |
Genetic reagent (C. elegans) | tph-1 (mg280) II | Caenorhabditis Genetic Center | Strain Name: MT15434 | |
Genetic reagent (C. elegans) | hsf-1(sy441)I | Caenorhabditis Genetic Center | Strain Name: PS3551 | |
Genetic reagent (C. elegans) | lite-1(ce314); ljIs102 [tph-1;;ChR2::YFP;unc-122::gfp] | Caenorhabditis Genetic Center | Strain Name: AQ2050 | |
Genetic reagent (C. elegans) | hmg-3(bar24[hmg-3::3xHA]) I | Gift from Dr. Baris Tursun, Max Delbrück Center (MDC) | Strain Name: BAT1560 | |
Genetic reagent (C. elegans) | glp-4(bn2) I | Caenorhabditis Genetic Center | Strain Name: SS104 | |
Genetic reagent (C. elegans) | rrf-1(pk1417) I | Caenorhabditis Genetic Center | Strain Name: NL2098 | |
Genetic reagent (C. elegans) | mkcSi13 II; rde-1(mkc36) V | Caenorhabditis Genetic Center | Strain Name: DCL569 | |
Genetic reagent (C. elegans) | rde-1(ne219) V; kbIs7 | Caenorhabditis Genetic Center | Strain Name: VP303 | |
Genetic reagent (C. elegans) | tph-1 (mg280) II; hsf-1::flag I | Prahlad lab, University of Iowa | Cross made with hsf-1::3Xflag (I) strain and tph-1 (II) | This paper (Materials and methods) |
Genetic reagent (C. elegans) | tph-1 (mg280) II; hmg-3(bar24[hmg-3::3xHA]) I | Prahlad lab, University of Iowa | Cross made with FLAG tagged train and tph-1 | This paper (Materials and methods) |
Cell line (Homo-sapiens) | NTERA-2 cl.D1 (NT2 cell line) | Originally from Stratagene | A gift from Dr. Christopher Stipp, University of Iowa (PMID:10806098) | |
Antibody | Mouse monoclonal anti-FLAG | Sigma | Catalog No. F1804 | IF (1:100), WB (1:1000) |
Antibody | Mouse monoclonal anti-RNA polymerase II | BioLegend | Catalog No. 664906 | ChIP (5 µl per sample) |
Antibody | Rabbit polyclonal anti-Histone H3 | Abcam | Catalog No. ab1791 | ChIP (2 µl per sample) |
Antibody | Rabbit polyclonal anti-HA | Abcam | Catalog No. ab9110 | ChIP (5 µl per sample), WB (1:1000) |
Antibody | Rabbit polyclonal anti-HSF1 | Cell Signaling Technology | Catalog No. 4356 | IF (1:100), WB (1:1000) |
Antibody | Rabbit monoclonal anti-HSF1(S320) | Abcam | Catalog No. ab76183 | WB (1:1000) |
Antibody | Mouse monoclonal anti-Hsp70 | Gift from Dr. Richard Morimoto, Northwestern University | Clone: 3A3 | WB (1:1000) |
Antibody | Mouse monoclonal anti-α-tubulin | DSHB, University of Iowa | Catalog No. AA4.3 | WB (1:1000) |
Antibody | Donkey anti-mouse Cy3 | Jackson ImmunoResearch Laboratories | Catalog No. 715-165-150 | IF (1:1000) |
Antibody | AlexaFluor 488-conjugated goat anti-rabbit IgG | Invitrogen | Catalog No. A-11008 | IF (1:500) |
Antibody | Sheep anti-mouse IgG | Rockland Immunochemicals | Catalog No. 610-631-002 | WB (1:10,000) |
Antibody | Goat anti-rabbit IgG | Invitrogen | Catalog No. A21109 | WB (1:10,000) |
Sequence-based reagent | siRNA targeting human HSF1 | Santa Cruz Biotechnology | Catalog No. sc-35611 | These are pools of 3–5 target-specific 19–25 nucleotide sequences designed by the company |
Sequenced-based reagent | siRNA targeting human SUPT16H | Santa Cruz Biotechnology | Catalog No sc-37875 | These are pools of 3–5 target-specific 19–25 nucleotide sequences designed by the company |
Sequenced-based reagent | Control siRNA | Santa Cruz Biotechnology | Catalog No sc-37007 | These are pools of 3–5 target-specific 19–25 nucleotide sequences designed by the company |
Commercial assay or kit | Mouse anti-FLAG M2 magnetic bead | Sigma | Catalog No. M-8823 | ChIP (15 µl per sample) |
Commercial assay or kit | Protein A/G magnetic bead | Pierce | Catalog No. 88802, Pierce | ChIP (15 µl per sample) |
Commercial assay or kit | iScript cDNA Synthesis Kit | Bio-Rad | Catalog No. 170–8891 | |
Commercial assay or kit | SYBR Green Master Mix | Roche | Catalog No. 04887352001 | |
Commercial assay or kit | Lipofectamine LTX Plus reagent | Thermo Fisher Scientific | Catalog No. 15338030 | |
Commercial assay or kit | ChIP DNA purification kit | Zymo Research | Catalog No. D5205 | |
Chemical compound, drug | 5-HT | Sigma | Catalog No. 85036 | |
Chemical compound, drug | Sumatriptan succinate (5-HT1 receptor agonist) | Sigma | Catalog No. S1198 | |
Chemical compound, drug | DOI hydrochloride (5-HT2A receptor agonist) | Sigma | Catalog No. D101 | |
Chemical compound, drug | BIMU8 hydrate (5-HT4 receptor agonist) | Sigma | Catalog No. B4063 | |
Chemical compound, drug | ST1936 (5-HT6 receptor agonist) | Sigma | Catalog No. SML0260 | |
Chemical compound, drug | PKA inhibitor H89 | Sigma | Catalog No. B1427 | |
Software, algorithm | ImageJ (FIJI) | NIH | Quantification of fluorescence intensity | |
Software, algorithm | LAS X | Leica Microsystems | Confocal imaging and analysis |
Additional files
-
Supplementary file 1
Genes differentially expressed (FDR < 0.01) in wild-type animals upon 5 min heat shock at 34°C.
Day one adult wild-type N2 animals were exposed to 34°C for 5 min. Animals were harvested immediately, and differential gene expression (FDR < 0.01) was calculated by comparing heat-shocked animal with control animals at 20°C.
- https://cdn.elifesciences.org/articles/55246/elife-55246-supp1-v2.xlsx
-
Supplementary file 2
No differential expression (FDR < 0.01) could be detected in hsf-1 mutant animals hsf-1(sy441) I upon 5 min heat shock at 34°C.
Day one adult hsf-1 (sy441) I animals were exposed to 34°C for 5 min. Animals were harvested immediately, and differential gene expression (FDR < 0.01) was calculated by comparing heat-shocked animal with control hsf-1 (sy441) I animals at 20°C. Note: no differential gene expression was detected.
- https://cdn.elifesciences.org/articles/55246/elife-55246-supp2-v2.xlsx
-
Supplementary file 3
Genes differentially expressed (FDR < 0.01) in tph-1 (mg280) II animals upon 5 min heat shock at 34°C.
Day one adult tph-1 (mg280) II animals were exposed to 34°C for 5 min. Animals were harvested immediately, and differential gene expression (FDR < 0.01) was calculated by comparing heat-shocked animal with control tph-1 (mg280) II animals at 20°C.
- https://cdn.elifesciences.org/articles/55246/elife-55246-supp3-v2.xlsx
-
Transparent reporting form
- https://cdn.elifesciences.org/articles/55246/elife-55246-transrepform-v2.docx