Late-life restoration of mitochondrial function reverses cardiac dysfunction in old mice
Figures
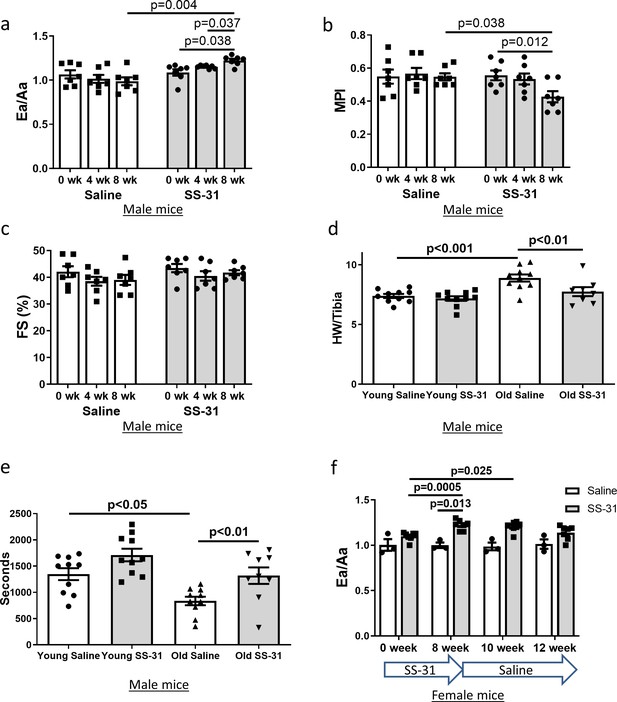
SS-31 treatment reverses cardiac aging phenotypes and improves exercise performance in old mice.
Doppler echocardiography showed that 8-week SS-31 treatment (a) improved diastolic function (increased Ea/Aa) and (b) enhanced myocardial performance (reduced myocardial performance index, MPI) of old male mice. (c) Fractional shortening (FS) was not altered by SS-31 treatment. (a–c) n = 7 male mice/group were analyzed by repeated measure ANOVA with Tukey’s multiple comparison test between time points and Sidak post hoc analysis between treatment groups. (d) 8- week SS-31 treatment reversed the age-related increase in normalized heart weight. n = 10 (for young saline, young SS-31 and old saline) and n = 8 (for old SS-31) male mice. Data were analyzed by one-way ANOVA with SNK post hoc analysis. (e) Treadmill running was impaired (reduced running time) in old control male mice but was rescued by SS-31 treatment. n = 10 (for young saline, young SS-31 and old saline) and n = 9 (for old SS-31) male mice, analyzed by one-way ANOVA with SNK post hoc analysis. (f) The improved diastolic function in old female mice (increased Ea/Aa) after 8 week of SS-31 treatment persisted for 2–4 weeks after cessation of treatment. n = 3 for saline control and n = 7 for SS-31 treatment, analyzed by repeated measure ANOVA with Tukey’s multiple comparison test between time points and Sidak post hoc analysis between treatment groups.
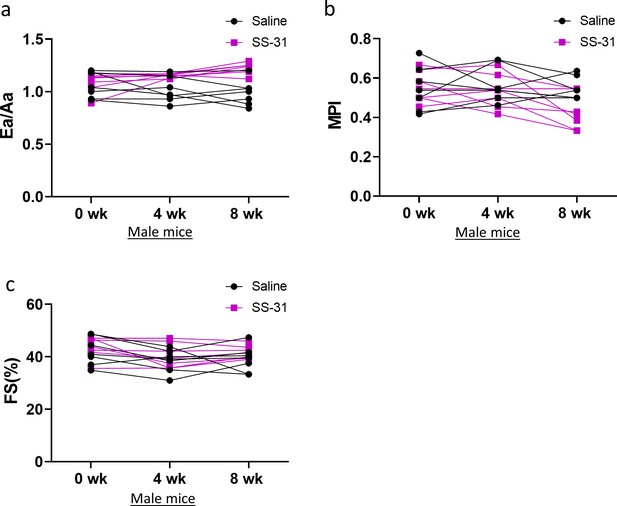
Individual trajectories of SS-31 responses in cardiac function of male mice.
8-week SS-31 treatment improved diastolic function and myocardial performance over time. Linear regression analyses treating each mouse in a group as a separate point revealed increased Ea/Aa (a) over time in old SS-31 treated (R = 0.45, p<0.001) but not old control mice (p=0.23) and reduced MPI (b) in old SS-31 treated (R = 0.30, p=0.011) but not old control mice (p=0.98). (c) FS remained unchanged in old SS-31 treated (p=0.26) and old control mice (p=0.42) during the 8-week treatment. N = 7 mice per group.
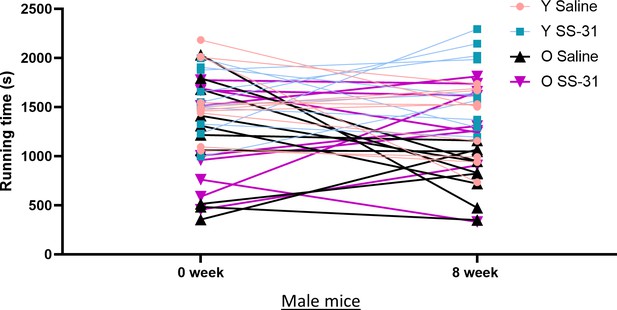
Individual trajectories of SS-31 responses in exercise performance of male mice.
Treadmill running time trended to decline in majority of old saline control mice (p=0.11) but not in old SS-31 treated mice (p=0.48); n = 10 for old control and n = 9 for old SS-31, analyzed by Wilcoxon signed-rank test with pairing of 0 week and 8 week data.

Individual trajectories of SS-31 responses in cardiac function of female mice.
8-week SS-31 treatment improved diastolic function in old female mice (p<0.001 between 0 week and 8 week by paired T-test); this improvement persisted 2 weeks after cessation of treatment (p=0.006 between 0 week and 10 week by paired T-test). At 4 weeks after treatment cessation, some mice displayed declined Ea/Aa, but overall, Ea/Aa was not significantly different from baseline (p=0.38 between 0 week and 12 week by paired T-test); n = 3 for saline control and n = 7 for SS-31 treatment.
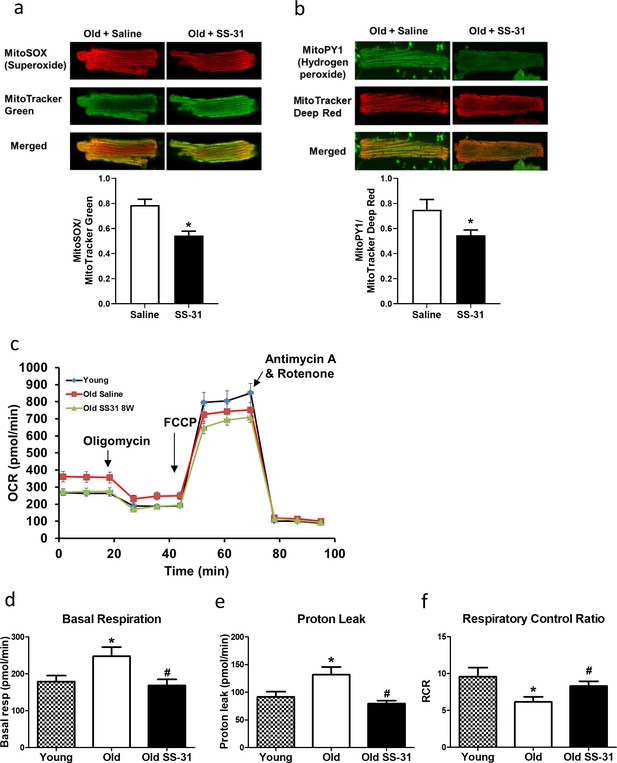
SS-31 treatment reduces ROS production and improves respiration in cardiomyocytes.
(a) SS-31 treated cardiomyocytes showed reduced mitochondrial superoxide, indicated by reduced MitoSox signal (normalized to mitochondrial content by the ratio to MitoTracker Green), compared to old controls. *p<0.05 vs old saline; n = 67 cells from three female mice for old saline and n = 71 cells from three female mice for old SS-31, compared by unpaired T-test. (b) SS-31 treated cardiomyocytes showed reduced hydrogen peroxide, indicated by reduced mitoPY1 signal (normalized to mitochondrial content using MitoTracker Deep Red), compared to old controls. *p<0.05 vs old saline; n = 31 cells from three female mice for old saline and n = 29 cells from three female mice for old SS-31, compared by unpaired T-test. Images for MitoSox and MitoPY1 measurements can found in Figure 2—source data 1 and Figure 2—source data 2. (c) Averaged traces of oxygen consumption rate (OCR, + / - SEM) of isolated cardiomyocytes from young, old, and old SS-31 treated male and female mice measured by the Seahorse XF Cell Mito Stress Test. Cardiomyocytes from old mice exhibited increased basal respiration (d) and proton leak (e) compared to that of young mice, and these age-related increases were reversed in cardiomyocytes from 8-week SS-31 treated old mice. (f) Old cardiomyocytes exhibited reduced respiratory control ratio (RCR) compared to young cardiomyocytes and this decrease was partially restored by 8-week SS-31 treatment. (d–f) *p<0.05 vs. young saline; #p<0.05 vs. old saline; n = 16 wells from four mice for young, n = 29 wells from six mice for old and n = 35 wells from six mice for old SS-31, analyzed by one-way ANOVA with SNK post hoc analysis.
-
Figure 2—source data 1
All image files for MitoSOX analysis in Figure 2a.
- https://cdn.elifesciences.org/articles/55513/elife-55513-fig2-data1-v2.zip
-
Figure 2—source data 2
All image files for MitoPY1 analysis in Figure 2b.
- https://cdn.elifesciences.org/articles/55513/elife-55513-fig2-data2-v2.zip
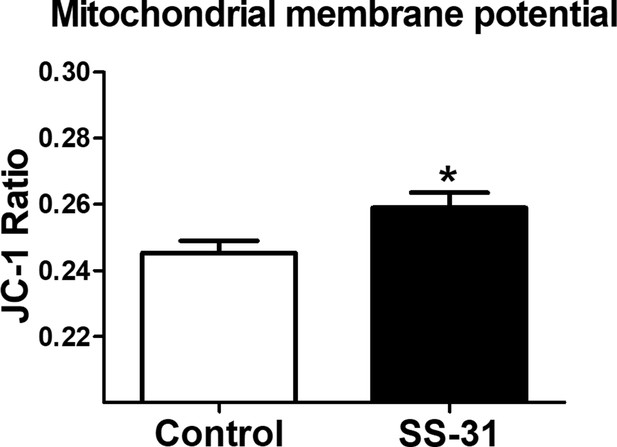
SS-31 treatment increases mitochondrial membrane potential in aged cardiomyocytes.
*p<0.05 vs old saline. n = 19 (Control) and 18 (SS-31) cells were analyzed by unpaired T-test.
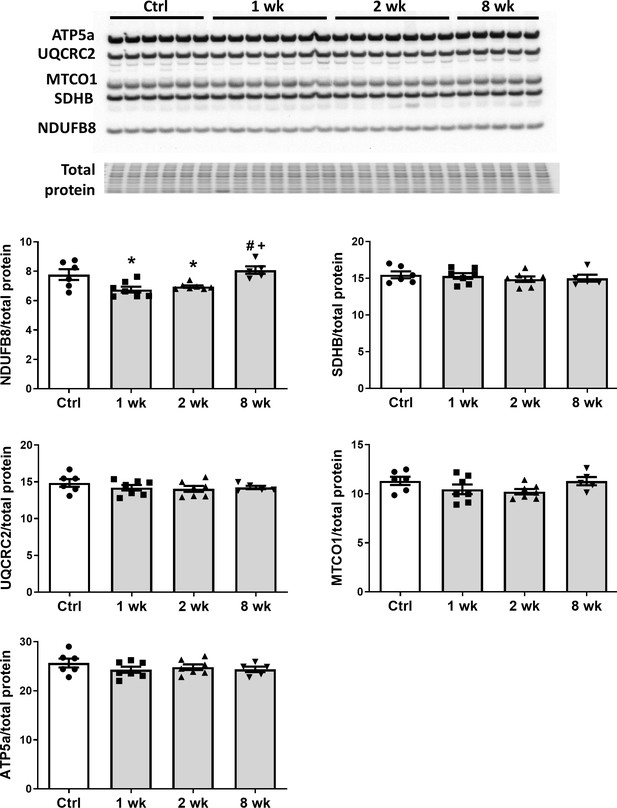
SS-31 treatment does not alter expression of subunits of oxidative phosphorylation complexes.
Immunoblotting using anti-OXPHOS antibody detected no differences in expression levels of OXPHOS subunits (NDUFB8, SDHB, UQCRC2, MTCO1, and ATP5A) in hearts of old male mice treated with SS-31 for 8 weeks. Only transient changes in NDUFB8 levels were detected at 1 and 2 weeks after SS-31 treatment. *p<0.05 vs Control; +p<0.05 vs 1-week SS-31; #p<0.05 vs 2-week SS-31 treatment; n = 6 for Control, n = 7 for 1 week and 2 week and n = 5 for 8 week, analyzed by one-way ANOVA with SNK post hoc analysis.
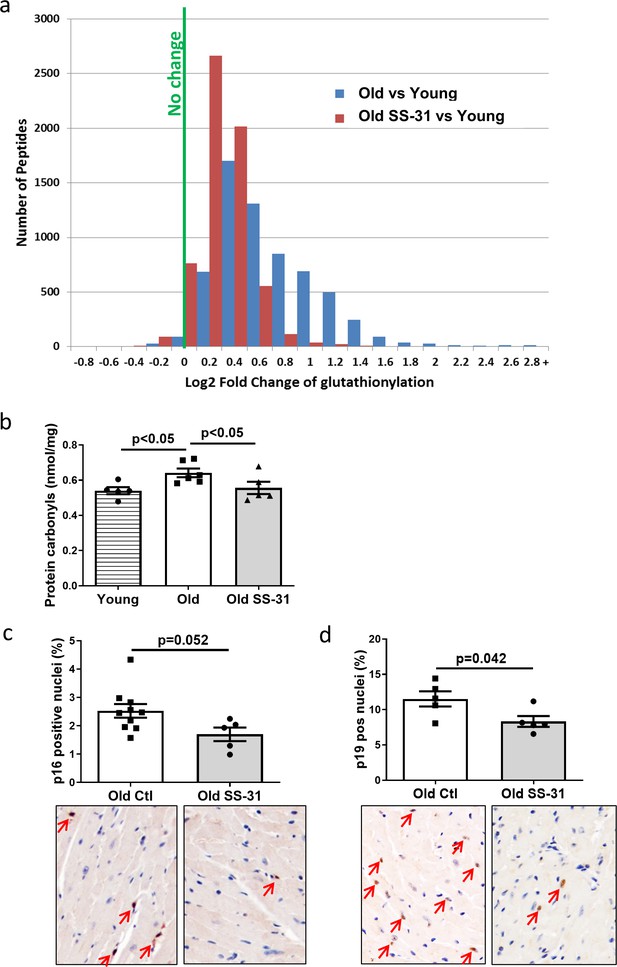
SS-31 treatment reduces protein oxidation and senescence in old hearts.
(a) A histogram of the distribution of changes in glutathionylation levels in peptides from old control and old SS-31 treated hearts; n = 3 female mice per group, analyzed as described in the Materials and method section. (b) Increased levels of protein carbonylation were detected in hearts of old control mice, but not old SS-31 treated mice, when compared to young control mice; n = 5 female mice per group, analyzed by one-way ANOVA with SNK post hoc analysis. (c–d) IHC staining of cellular senescence markers, p16 (c) and p19 (d), detected reduced p16 and p19 positive nuclei in old SS-31 treated heart compared to old control hearts; n = 5 male mice per group, analyzed by unpaired T-test. Images for p16 and p19 staining can be found in Figure 4—source data 1 and Figure 4—source data 2.
-
Figure 4—source data 1
All image files for p16 analysis in Figure 4c.
- https://cdn.elifesciences.org/articles/55513/elife-55513-fig4-data1-v2.zip
-
Figure 4—source data 2
All image files for p19 analysis in Figure 4d.
- https://cdn.elifesciences.org/articles/55513/elife-55513-fig4-data2-v2.zip
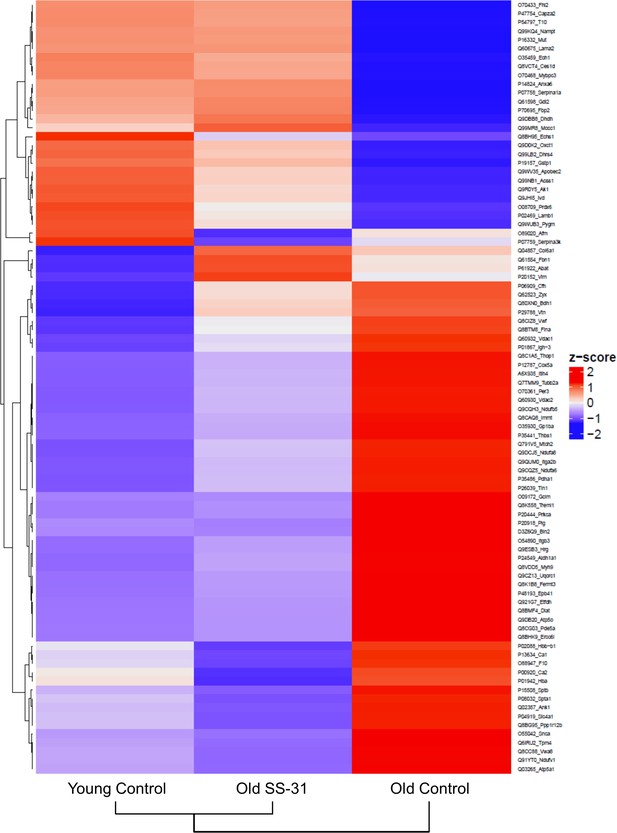
SS-31 treatment partially restores age-related proteomic remodeling.
A heatmap of z-scores the 88 proteins that were significantly altered by both aging (q < 0.05 for old control vs. young control) and SS-31 treatment (q < 0.05 for old SS-31 vs. old control); n = 9, 10, and eight male mice for young control, old control and old SS-31, respectively, analyzed as described in the method section. We computed the z-scores of the average log2 abundance values for each of the three groups, where we adjusted the data, by protein, to have a mean of zero and a standard deviation of 1. The heatmap was generated using the ComplexHeatmap (v.1.20.0) R package (Gu et al., 2016), where both the sample groups and the proteins were clustered via the hclust function with the ‘complete’ agglomeration method. Distance matrix for clustering were computed using ‘Euclidean’ distance. The resulting heatmap presents the proteins in rows and sample groups in columns, both of which were grouped according to the clustering results. Row labels on the right are the UniProt ID_Gene Name of each protein. The identities and fold changes of all protein identified are listed in Supplementary file 3.

SS-31 treatment induces modest changes in metabolome that partially attenuates the age-related changes.
A heat map of the relative levels of the 18 metabolites that were significantly (FDR < 0.05) different among treatment groups; n = 10 (for YCL, YSS, OCL) and n = 8 (for OSS) were male mice were analyzed as described in the method section.
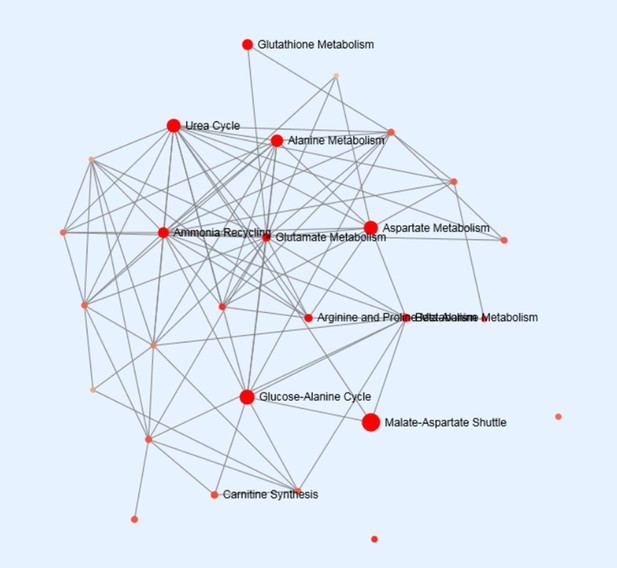
A network of metabolite set enrichment for the 11 metabolites that were significantly (p<0.05, by Tukey’s HSD) altered by aging.
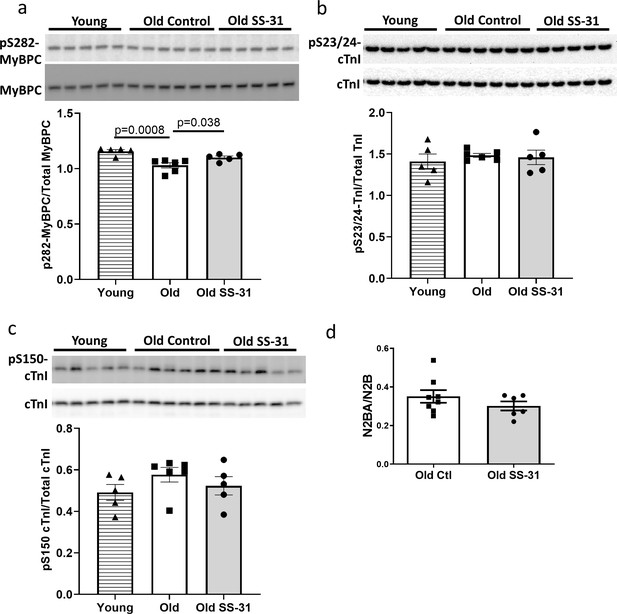
SS-31 rescues the age-related hypo-phosphorylation of MyBP-C.
(a) Old murine hearts displayed reduced levels of MyBP-C phosphorylation at Ser282, which is normalized by SS-31 treatment. (b–c) Aging and SS-31 treatment did not alter phosphorylation of cTnI at Ser23/24 (b) and Ser150 (c) in hearts For panel a-c, n = 5, 6, and five male mice for young control, old control and old SS-31, respectively, analyzed by one-way ANOVA Dunnett’s post hoc analysis for panel a-c. (d) Titin isoform ratio (N2BA/N2B ratio) did not change with SS-31 treatment; n = 8 and 6 female mice were used for old control and old SS-31, respectively, and were compared by unpaired T-test.
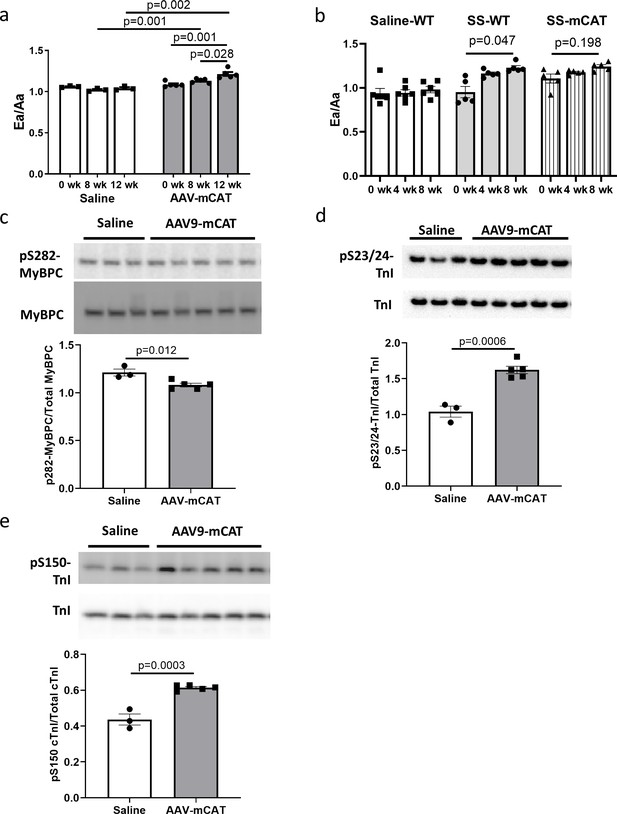
The cardiac benefit of SS-31 treatment is not additive to that of mCAT expression but the two interventions differentially regulate myofilament protein phosphorylation.
(a) Diastolic function (Ea/Aa) improved at both 8 and 12 weeks after AAV9-mCAT administration. n = 3 (saline) and n = 5 (AAV-mCAT) female mice were analyzed by repeated measure ANOVA with Tukey’s multiple comparison test between time points and Sidak post hoc analysis between treatment groups. (b) 8-week SS-31 improved diastolic function in old WT but did not further improve the function of old mCAT mice; n = 5 (for SS-WT and SS-mCAT) and n = 6 (for Saline-WT) mixed-sex mice were analyzed by repeated measure ANOVA with Tukey’s multiple comparison test between time points. (c) Late-life mCAT expression reduced Ser282 phosphorylation of MyBP-C. (d–e) Late-life mCAT expression increased phosphorylation of cTnI at Ser23/24 (d) and Ser150 (e). For panel c-e, n = 3 and 5 female mice were used for saline and AAV-mCAT, respectively, and were analyzed by unpaired T-test.
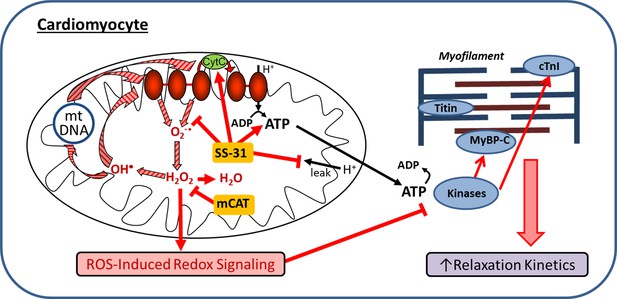
Schematic outline of results and interpretation.
While mCAT and SS-31 both inhibit electron transport chain produced ROS, they do so by different mechanisms. Both inhibit a ROS-mediated vicious cycle (ROS induced mtDNA and protein damage leads to greater ROS generation; striped arrows) and ROS-Induced redox signaling. However, by promoting electron transport, preventing proton leakage and augmenting ATP production, SS-31 also improves mitochondrial energetics. By improving mitochondrial energetics and reducing pathologic redox signaling, SS-31 promotes phosphorylation of cMyBP-C to enhance myofilament relaxation kinetics, while mCAT expression does so through promoting phosphorylation of cTnI.
Tables
Reagent type (species) or resource | Designation | Source or reference | Identifiers | Additional information |
---|---|---|---|---|
Strain, strain background (M. musculus; male and female) | C57BL/6J | National Institute of Aging Charles River colony | RRID:IMSR_JAX:000664 | |
Genetic reagent (M. musculus) | mCAT | Rabinovitch Lab; PMID:15879174 | RRID:IMSR_JAX:016197; Tg(CAG-OTC/CAT)4033Prab | Now available at The Jackson Lab |
Transfected construct (M. musculus) | AAV9-mCAT | Duan Lab, PMID:19690612 | AV.RSV.MCAT | Adeno-associated virus construct to transfect and express mCAT transgene |
Antibody | Anti-OXPHOS (Rabbit polyclonal) | Abcam | ab110413 RRID:AB_2629281 | (1:500) |
Antibody | Anti-Troponin I (Rabbit polyclonal) | Cell Signaling Technology | #4002 RRID:AB_2206278 | (1:1000) |
Antibody | Anti-pSer23/24-Troponin I (Rabbit polyclonal) | Cell Signaling Technology | #4004 RRID:AB_2206275 | (1:1000) |
Antibody | Anti-pSer150-Troponin I (Rabbit polyclonal) | ThermoFisher | PA5-35410 RRID:AB_2552720 | (1:1000) |
Antibody | Anti- cMyBP-C (Mouse monoclonal) | Santa Cruz SC-137237 | SC-137237 RRID:AB_2148327 | (1:1000) |
Antibody | Anti-pSer282-cMyBP-C (Rabbit polyclonal) | Enzo | ALX-215–057 R050 RRID:AB_2050502 | (1:2000) |
Antibody | anti-p19 (Rabbit polyclonal) | LSBio | LS-C49180 RRID:AB_1192824 | (1:300) |
Antibody | anti-p16 (Rabbit polyclonal) | Abcam | ab211542 | (1:300) |
Antibody | Donkey anti-Rabbit Secondary Antibody, HRP | ThermoFisher | A16035 RRID:AB_2534709 | (1:10000) |
Antibody | Goat anti-Mouse Secondary Antibody, HRP | ThermoFisher | A16072 RRID:AB_2534745 | (1:10000) |
Peptide, recombinant protein | SS-31 peptide (Elamipretide) | Stealth BioTherapeutics | 3 µg/g body weight/day | |
Commercial assay or kit | OxiSelect protein carbonyl ELISA kit | Cell Biolabs | STA-310 | |
Commercial assay or kit | ImmPRESS-VR Anti-Rabbit IgG HRP Polymer Detection Kit | Vector Laboratories | MP-6401–15 | |
Commercial assay or kit | Seahorse XF Cell Mito Stress Test Kit | Aligent/Seahorse Bioscience | 103015–100 | |
Commercial assay or kit | MitoSOX Red | ThermoFisher | M36008 | |
Commercial assay or kit | MitoPY1 | Fisher Scientific/Tocris Bioscience | 44-281-0 | |
Commercial assay or kit | MitoTracker Green | ThermoFisher | M7514 | |
Commercial assay or kit | MitoTracker Deep Red | ThermoFisher | M22426 | |
Commercial assay or kit | JC-1 Dye | ThermoFisher | T3168 | |
Commercial assay or kit | BCA protein assay | Thermo Scientific | 23225 | |
Commercial assay or kit | Pierce Reversible Protein Stain Kit for PVDF Membranes | Thermo Scientific | 24585 | |
Commercial assay or kit | SuperSignal West Pico PLUS Chemiluminescent Substrate | Thermo Scientific | 34580 | |
Software, algorithm | Graphpad Prism | Graphpad | RRID:SCR_002798 | |
Software, algorithm | AlphaView Software | ProteinSimple | ||
Software, algorithm | Metaboanalyst 4.0 | www.metaboanalyst.ca; PMID:29762782 | RRID:SCR_015539 | |
Software, algorithm | Topograph | MacCoss Lab software; PMID:22865922 | ||
Software, algorithm | ComplexHeatmap | https://github.com/jokergoo/ComplexHeatmap PMID:27207943 | RRID:SCR_017270 |
Additional files
-
Supplementary file 1
A dataset of relative abundances of all metabolites measured.
- https://cdn.elifesciences.org/articles/55513/elife-55513-supp1-v2.csv
-
Supplementary file 2
A table of all metabolites that showed significant differences in one or more comparisons.
- https://cdn.elifesciences.org/articles/55513/elife-55513-supp2-v2.xlsx
-
Supplementary file 3
A dataset of identities, fold changes and statistics of all proteins identified in the proteomic analysis.
- https://cdn.elifesciences.org/articles/55513/elife-55513-supp3-v2.csv
-
Transparent reporting form
- https://cdn.elifesciences.org/articles/55513/elife-55513-transrepform-v2.pdf