The biogenesis of CLEL peptides involves several processing events in consecutive compartments of the secretory pathway
Figures
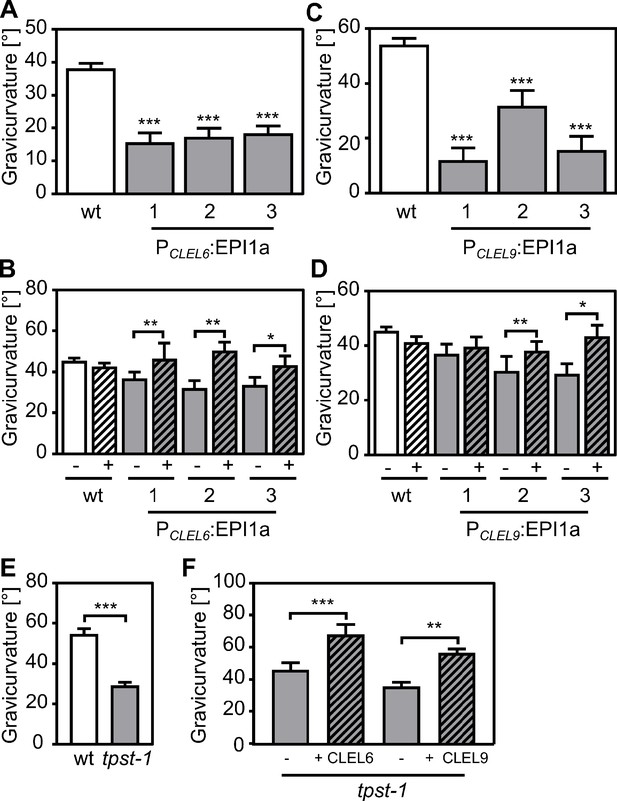
Reduced gravicurvature of PCLEL6:EPI1a, PCLEL9:EPI1a and tpst-1 seedlings is rescued by addition of mature CLEL6 or CLEL9 peptides.
Gravicurvature of (A) three independent PCLEL6:EPI1a and (C) PCLEL9:EPI1a lines (gray bars; promoter constructs are shown in Figure 1—figure supplement 1) is significantly reduced in comparison to the wild type (white bars). The same effect was observed when the EPI10 inhibitor was expressed under control of the CLEL6 or CLEL9 promoter (Figure 1—figure supplement 2). Gravicurvature of PCLEL6:EPI1a and PCLEL9:EPI1a lines is restored to wild-type levels by application of (B) 10 nM CLEL6 or (D) 300 nM CLEL9 (hatched bars). (E) tpst-1 gravicurvature in comparison to the wild-type control. (F) Gravicurvature of tpst-1 seedlings treated (hatched bars) with CLEL6 (10 nM) or CLEL9 (300 nM) as compared to the untreated tpst-1 control (gray bars). Seedlings were grown for five days in the dark on ½ MS medium with peptides added as indicated. Plates were rotated 90° and gravicurvature was assessed after two days as the angle of the hypocotyl with the horizontal. Panel A was modified from Stührwohldt et al. (2017). Data are shown for one representative of at least two independent experiments as the mean ± SE (n ≥ 15). *, **, and *** indicate significant differences at p<0.05, p<0.01, and p<0.001, respectively (two-tailed t test). The gravitropic response of roots was not affected in transgenic plants expressing the EPI inhibitors under control of CLEL promoters (Figure 1—figure supplement 3).
-
Figure 1—source data 1
Source data for hypocotyl gravitropic responses shown in Figure 1 and Figure 1—figure supplement 2.
- https://cdn.elifesciences.org/articles/55580/elife-55580-fig1-data1-v1.xlsx
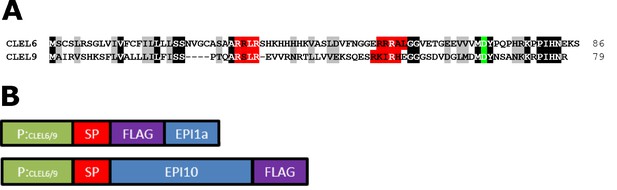
Sequence alignment of CLEL6 and CLEL9 and schematic representation of the promoter-EPI constructs.
(A) Sequence alignment of CLEL6 and CLEL9 precursors. Conserved pre-processing and processing sites are highlighted in red and green, respectively. (B) Schematic representation of expression constructs showing the CLEL6 and CLEL9 promoters in green. The open reading frames (ORFs) for EPI1a and EPI10 (blue) were codon-optimized and equipped with a plant signal peptide (SP, magenta) for efficient expression and secretion in planta. The EPI1a and EPI10 ORFs were extended with an N- or C-terminal FLAG tag (purple), respectively.
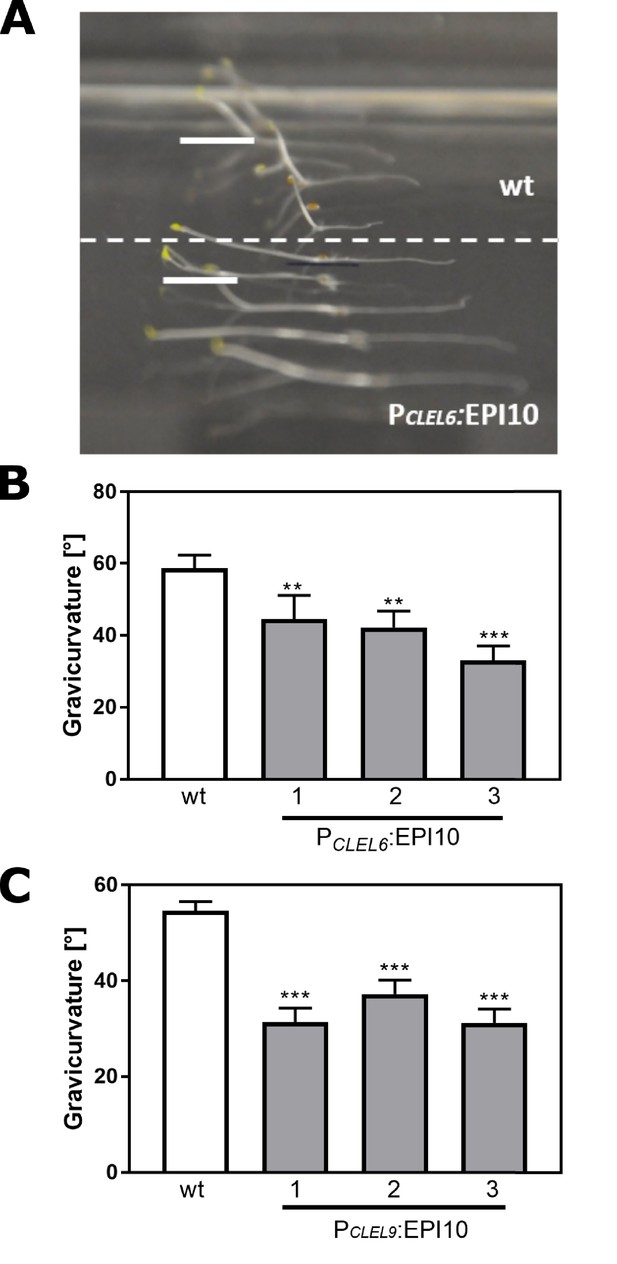
The gravitropic response of the hypocotyl is impaired in PCLEL6:EPI10 and PCLEL9:EPI10 transgenic lines.
Representative pictures of etiolated wild-type and PCLEL6:EPI10 seedlings differing in their gravitropic response are shown in (A). Seedlings were grown on slanted plates in the dark, rotated by 90 degrees, and after two more days the pictures were taken. (B) Gravitropic response of PCLEL6:EPI10 and wild-type seedlings assessed as the angle of the hypocotyl with the horizontal. (C) Gravitropic response of PCLEL9:EPI10 seedlings in comparison to the wild type. Representative data are shown for one out of three independent experiments as the mean ± SE (n ≥ 15). Asterisks indicate statistically significant differences at p<0.01 (**) and p<0.001 (***), respectively (two-tailed t test).
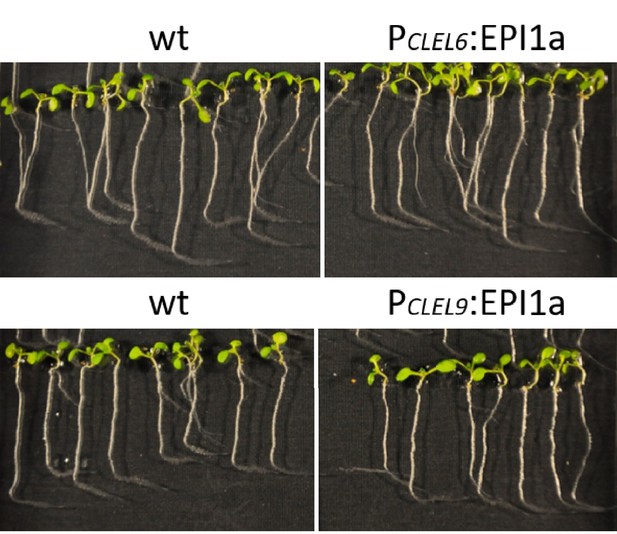
PCLEL6:EPI1a and PCLEL9:EPI1a lines are not affected in root gravitropism.
Seedlings of wildtype, PCLEL6:EPI1a and PCLEL9:EPI1a transgenic lines were grown for five days and were exposed to a 90° gravistimulus for two days. Representative pictures of two independent experiments are shown.
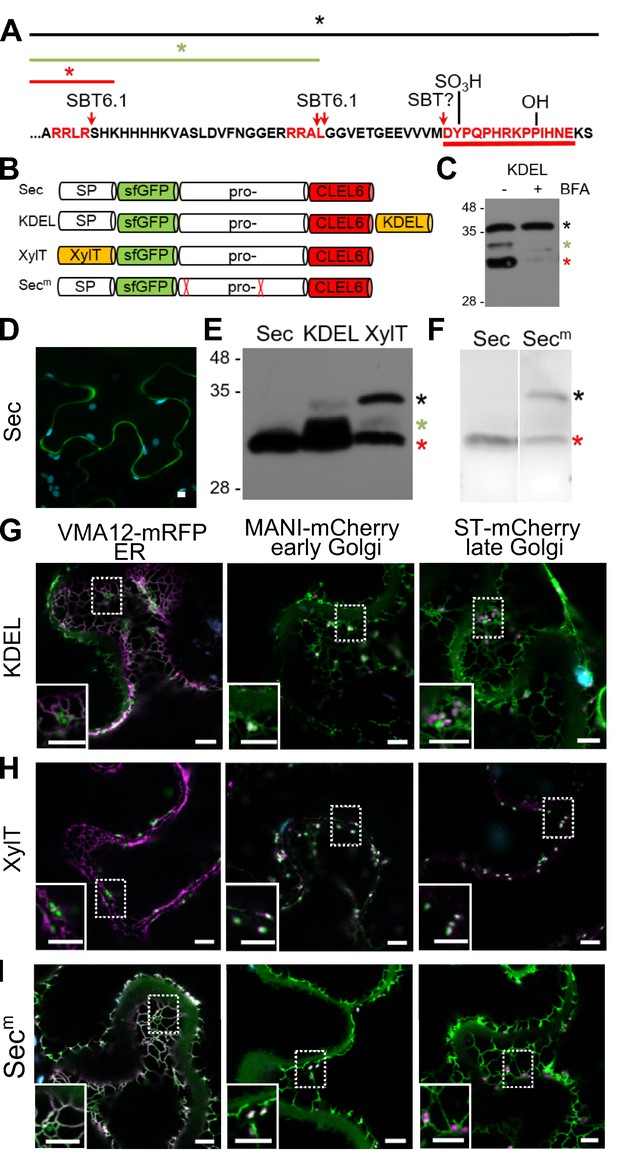
Pre-processing of CLEL6 by SBT6.1 in the cis-Golgi is required for peptide maturation and secretion.
(A) Sequence of the CLEL6 precursor without the signal peptide. Proteolytic processing sites and other post-translational modifications are indicated, mature CLEL6 peptide sequence underlined. Colored lines and asterisks represent the size of the processed forms of CLEL6 observed in panels C, E, and F. (B) Schematic representation of expression constructs used for subcellular localization studies. (C) Processing of the ER-restrained CLEL6 (KDEL) construct with (+) or without (-) BFA treatment, analyzed by anti-GFP immunoblotting. Different processed forms of the precursor are marked by colored asterisks as shown in panel A. (E, F) Immunoblot analysis of ER-restrained (KDEL) and Golgi-localized (XylT) precursor processing compared to the secreted form (Sec) and the precursor lacking the two SBT6.1 cleavage sites (Secm). Fully and partially processed precursors are indicated by the colored asterisks as defined in panel A. (D, G–I) Co-localization of the different fusion proteins with ER (VMA12-mRFP) and Golgi (ManI-mCherry and ST-mCherry) markers analyzed by fluorescence microscopy. Pictures show an overlay of the green (500–550 nm) and red (610–670 nm) fluorescence channels. The dotted areas are shown in higher magnification in the insets. Scale bars represent 5 µm.
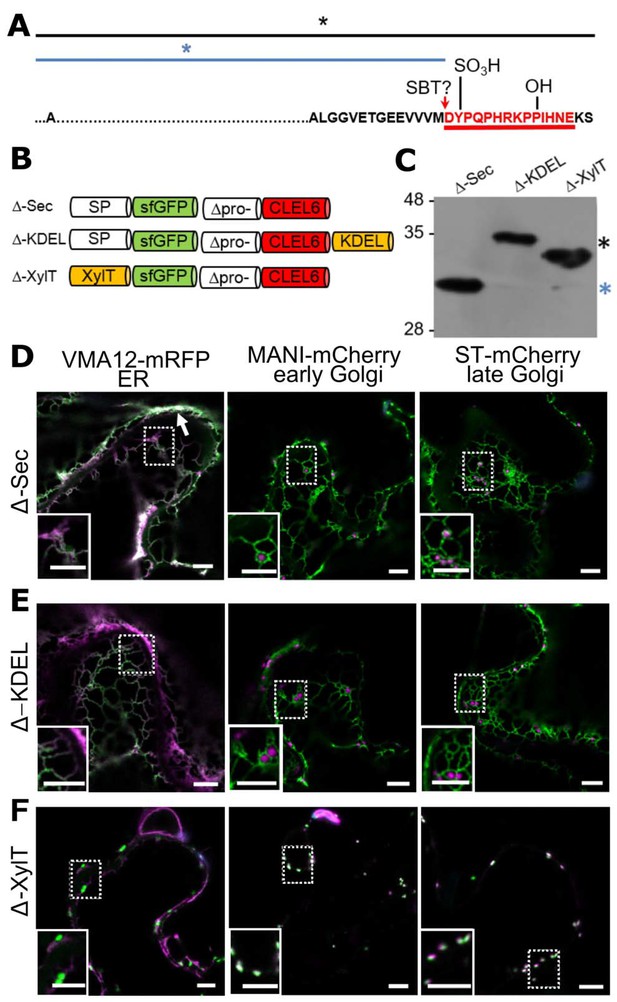
N-terminal maturation of CLEL6 occurs in a post-Golgi compartment (the subcellular localization of CLEL9 maturation is analyzed in Figure 3—figure supplement 1).
(A) Sequence and post-translational modification sites of Δ-CLEL6 constructs lacking the prodomain region encompassed by the two SBT6.1 cleavage sites; mature CLEL6 peptide sequence underlined. Black and blue lines and asterisks were included to represent the unprocessed and processed forms of the precursor, respectively. (B) Schematic representation of expression constructs used to localize the subcellular compartment of CLEL6 maturation. (C) Processing of the secreted (Δ-Sec), ER-restrained (Δ-KDEL) and Golgi-localized (Δ-XylT) constructs analyzed by anti-GFP immunoblotting. Unprocessed and processed forms of the precursor are indicated by the black and blue asterisks, respectively. (D–F) Co-localization of the different fusion proteins with ER (VMA12-mRFP) and Golgi (ManI-mCherry and ST-mCherry) markers analyzed by fluorescence microscopy. Pictures show an overlay of the green (500–550 nm) and red (610–670 nm) fluorescence channels. The dotted areas are shown in higher magnification in the insets. The white arrow marks apoplastic localization; scale bars represent 5 µm.
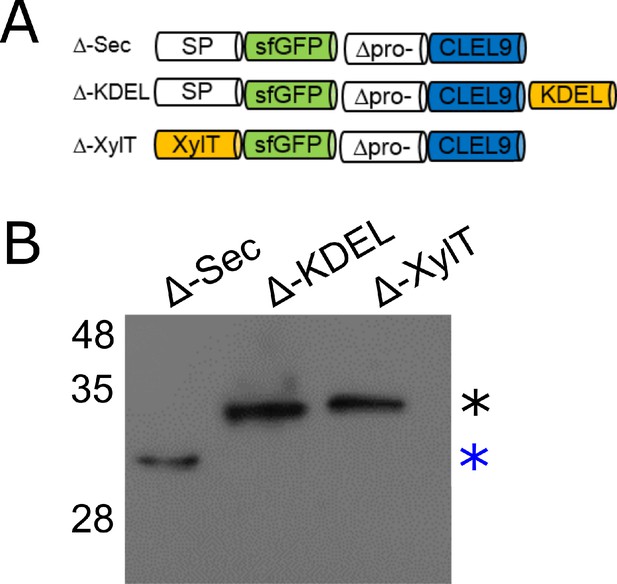
N-terminal maturation of CLEL9 occurs in a post-Golgi compartment.
(A) Constructs used to localize the subcellular compartment of the N-terminal maturation step of CLEL9 (B) Processing of the secreted (Δ-Sec), ER-restrained (Δ-KDEL) and Golgi-localized (Δ-XylT) prodomain-deletion constructs of CLEL9 transiently expressed in N. benthamiana was analyzed by anti-GFP immunoblotting. Processed and unprocessed precursors are marked by the blue and black asterisks, respectively.
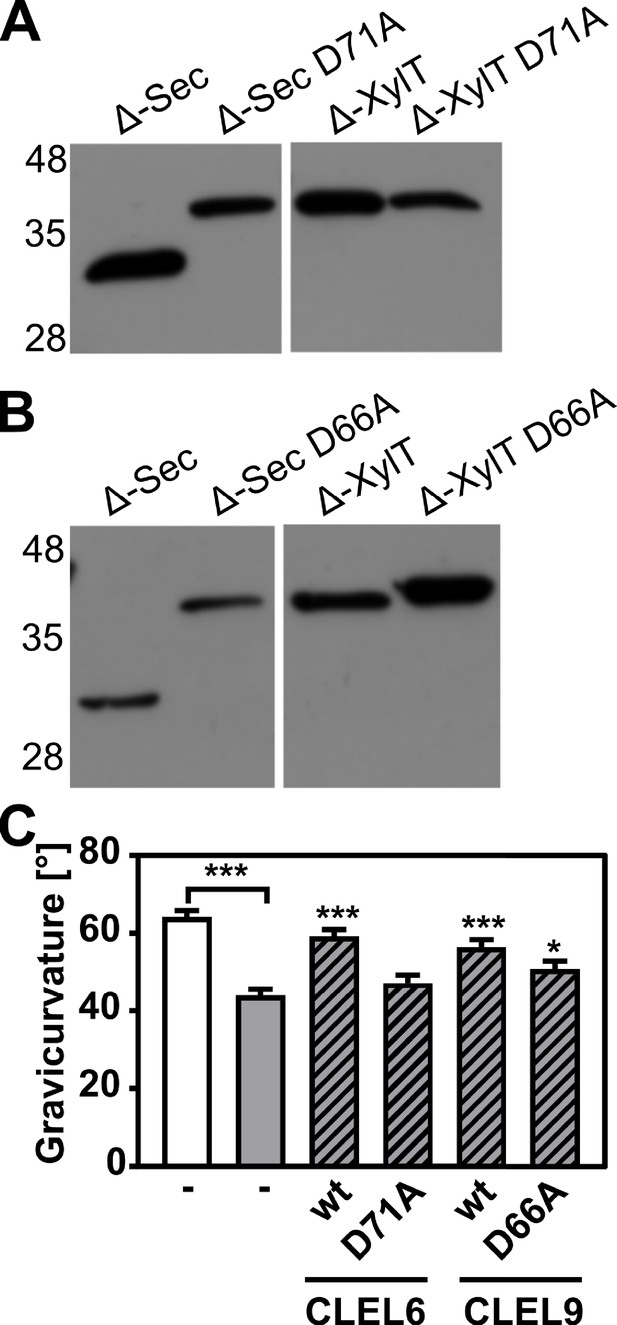
N-terminal maturation and the formation of bioactive CLEL6 and 9 peptides are aspartate-dependent.
(A, B) The relevance of the N-terminal aspartate for precursor processing was analyzed on anti-GFP immunoblots for the secreted (Δ-Sec) and Golgi-anchored (Δ-XylT) constructs by alanine substitution (D71A and D66A for the CLEL6 and CLEL9 precursors, respectively). Aspartate-dependent processing requires SBT activity (Figure 4—figure supplement 1). (C) Complementation of the gravitropic response of the tpst-1 mutant by CLEL peptides produced in planta. The gravitropic response of the tpst-1 mutant (gray bar) was restored to wild-type levels (white bar) by treatment with cell wall extracts from plants expressing the CLEL6 or CLEL9 precursors (hatched bars). Activity was much reduced for extracts from plants expressing the D71A and D66A precursor mutants. Seedlings were grown for five days in the dark on ½ MS medium with peptides added as indicated. Plates were rotated 90° and gravicurvature was assessed after two days as the angle of the hypocotyl with the horizontal. Data show the average of three independent experiments as the mean ± SE (n ≥ 103). Unless otherwise indicated * and *** indicate significant differences to the tpst-1 control (gray bar) at p<0.05 and p<0.001, respectively (two-tailed t test).
-
Figure 4—source data 1
Source data for hypocotyl gravitropic responses shown in Figure 4.
- https://cdn.elifesciences.org/articles/55580/elife-55580-fig4-data1-v1.xlsx
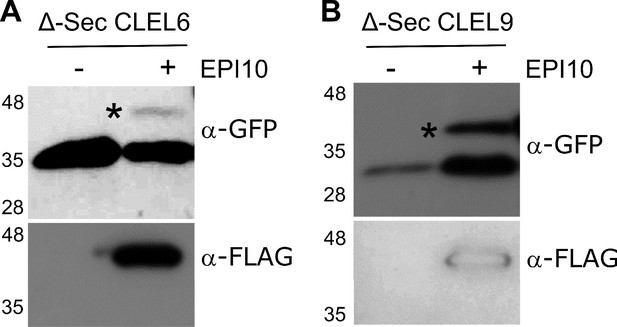
SBT activity is required for N-terminal maturation of CLEL6 and CLEL9.
To analyze the relevance of SBT activity for N-terminal maturation, Δ-Sec constructs of CLEL6 (A) and CLEL9 (B) precursors were co-expressed with SBT inhibitor EPI10. Processing of the GFP-tagged precursors was analyzed on anti-GFP western blots (top panel). The unprocessed precursors are marked by black asterisks. Expression of the EPI10 inhibitor was confirmed by anti-FLAG immunoblotting (bottom panel).
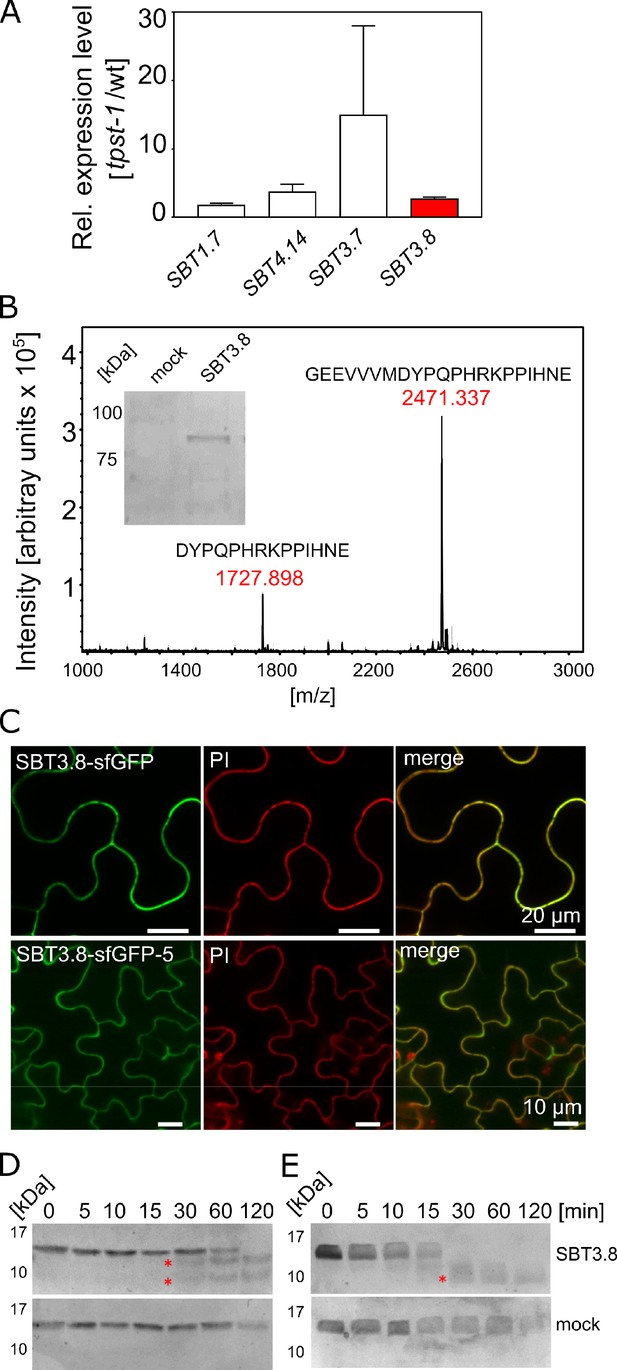
SBT3.8 is localized extracellularly and requires Asp at the cleavage site for peptide activation.
(A) qPCR analysis of SBT genes that are expressed at higher levels in etiolated tpst-1 hypocotyls in comparison to wild type. Relative SBT mRNA levels were determined after normalization to three reference genes (Actin2, EF and Tubulin). (B) MALDI-TOF analysis of eCLEL6 (GEEVVVMDYPQPHRKPPIHNE) cleavage by SBT3.8. Masses of eCLEL6 and mature CLEL6 (DYPQPHRKPPIHNE) are indicated. SDS-PAGE analysis of purified SBT3.8 and the mock control is shown in the insert. The control digest is shown in Figure 5—figure supplement 1. (C) Subcellular localization of SBT3.8-sfGFP transiently expressed in tobacco leaves (upper panels) and in stably transformed Arabidopsis plants (lower panels) under the control of the CaMV 35S promoter. Cell walls were stained with propidium iodide vacuum-infiltrated five min prior to imaging. (D) Cleavage of the CLEL6 precursor by SBT3.8. The recombinant CLEL6 precursor was incubated with purified SBT3.8 (top) or the mock control (bottom) for the time indicated. Cleavage was detected by Coomassie staining after SDS-PAGE. Red asterisks mark SBT3.8 cleavage products (E) Cleavage of the D71A CLEL6 precursor mutant by SBT3.8. The analysis was performed as described for (D). Note missing cleavage product in in (E) compared to (D).
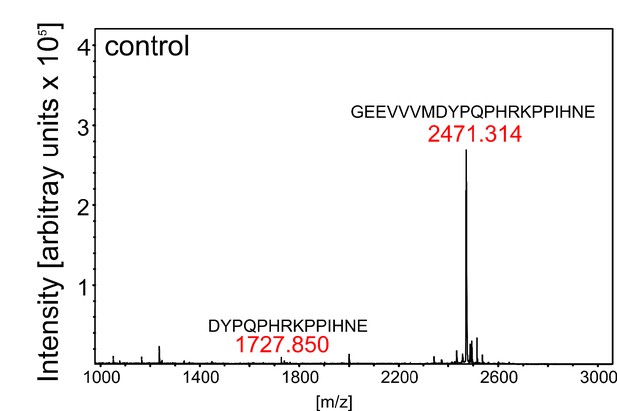
Control digest of eCLEL6.
MALDI-TOF analysis of eCLEL6 (GEEVVVMDYPQPHRKPPIHNE) cleavage by a mock-purified cell wall extract from empty-vector infiltrated plants. Masses of eCLEL6 and mature CLEL6 (DYPQPHRKPPIHNE) are indicated. The mature CLEL6 peptide, apparently generated by N. benthamiana protease(s) constitutively present in the leaf apoplast, is barely detectable.
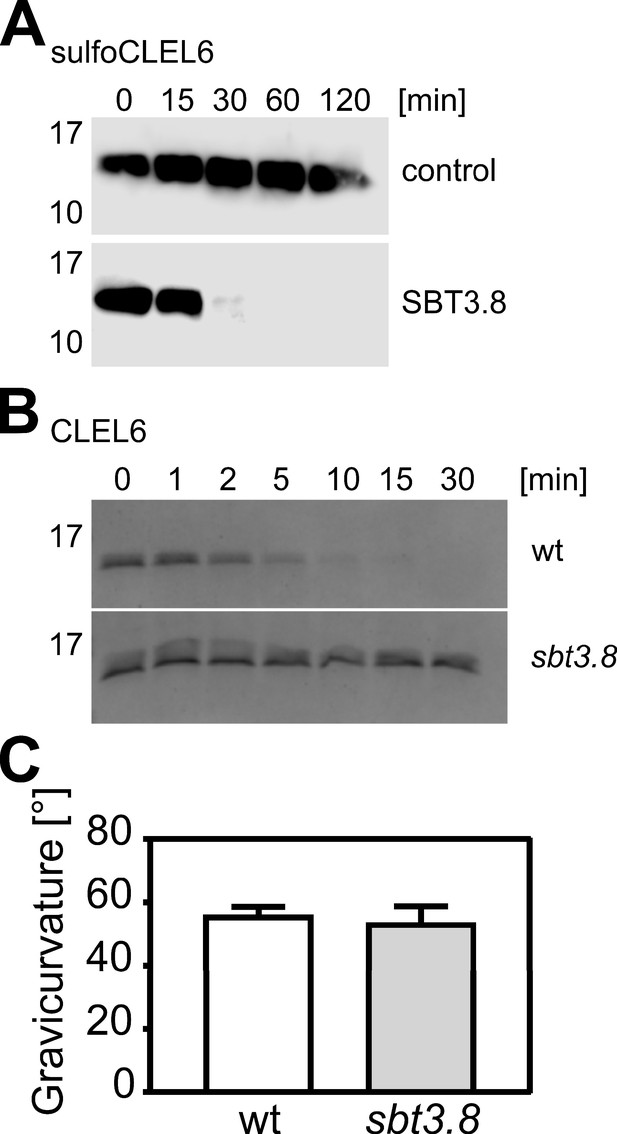
Cleavage of the CLEL6 precursor by SBT3.8 is not affected by tyrosine sulfation and impaired in the sbt3.8 mutant.
(A) Tyrosine-sulfated CLEL6 precursor sulfoCLEL6 is cleaved by SBT3.8. sulfoCLEL6 was expressed in E. coli and purified via the C-terminal His tag. Time-dependent cleavage of sulfoCLEL6 is observed in presence of SBT3.8 but not for the buffer control. Proteins were detected after SDS-PAGE on western blots developed with anti-HIS monoclonal antibodies. (B) The CLEL6 precursor is cleaved by wild-type seedling exudates, but not by sbt3.8 mutant exudates. Proteins were separated by Tris-Tricine PAGE and stained with Coomassie Brilliant Blue. (C) Gravitropic response of sbt3.8 loss-of-function mutants in comparison to the wild-type control. Seedlings were grown on slanted plates in the dark, rotated by 90 degrees, and after two more days seedlings were analyzed. Representative data are shown for one out of three independent experiments as the mean ± SE (n ≥ 22). No significant differences were observed (two-tailed t test).
-
Figure 5—figure supplement 2—source data 1
Source data for hypocotyl gravitropic responses shown in Figure 5—figure supplement 2.
- https://cdn.elifesciences.org/articles/55580/elife-55580-fig5-figsupp2-data1-v1.xlsx
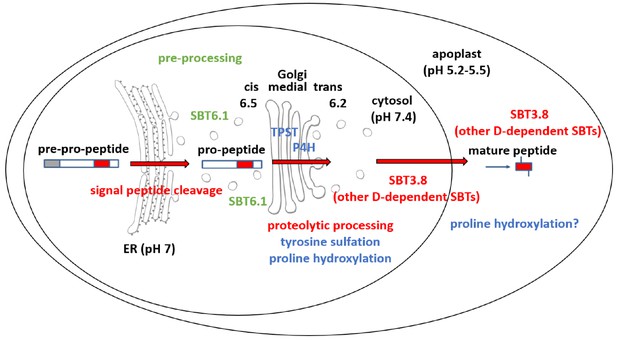
Maturation of the CLEL6 precursor in the secretory pathway.
As a first processing step, the signal peptide is cleaved off upon entry into the ER. After exit from the ER, the precursor is processed by SBT6.1 in the cis-Golgi at two S1P cleavage sites within its variable prodomain. Still in the Golgi, the peptide moiety is tyrosine-sulfated by TPST, and proline hydroxylated. Candidate proline-4-hydroxylases have been identified, but it is still unclear which of these enzymes is responsible for peptide modification. There is also circumstantial evidence for proline-4-hydroxylase activity in the cell wall (Stührwohldt et al., 2018). N-terminal maturation by Asp-dependent SBT3.8 occurs late in the secretory pathway in a post-Golgi compartment, or in the apoplastic space. The figure was modified and updated from Stührwohldt and Schaller (2019).
Tables
Reagent type (species) or resource | Designation | Source or reference | Identifiers | Additional information |
---|---|---|---|---|
Gene (Arabidopsis thaliana) | SBT3.8 | The ArabidopsisInformation Resource (TAIR) | At4g10540 | amplified from genomic DNA |
Strain, strainbackground (Escherichia coli) | BL21-RIL | Agilent | ||
Strain, strainbackground (Escherichia coli) | BL21 pEVOL SYRS | Liu et al., 2009 | ||
Genetic reagent(Arabidopsis thaliana) | sbt3.8 | Nottingham Arabidopsis Stock Center | SALK_052039 | |
Genetic reagent(Arabidopsis thaliana) | tpst-1 | Nottingham Arabidopsis Stock Center | SALK_009847 | |
Genetic reagent(Arabidopsis thaliana) | PCLEL6:EPI1a; PCLEL9:EPI1a | this paper | Transgenic lines expressingEPI1a under control of theCLEL6or CLEL9 promoter | |
Genetic reagent (Arabidopsis thaliana) | PCLEL6:EPI10; PCLEL9:EPI10 | this paper | Transgenic lines expressingEPI10 under control of theCLEL6 or CLEL9 promoter | |
Genetic reagent(Agrobacterium tumefaciens) | C58C1; GV3101 | Community resource | NCBI:txid176299 | GV3101 with and withoutpSOUP helper plasmid |
Antibody | anti-GFP, polyclonal, rabbit | Thermo Fisher Scientific | Cat# A-11122 | 1:10000 |
Antibody | anti-His, monoclonal, mouse | Dianova | Cat# DIA-900–200 | 1:10000 |
Antibody | anti-FLAG-HRP, monoclonal, mouse | Sigma-Aldrich | Cat# A8592 | 1:5000 |
Antibody | Goat-anti-rabbit IgG, HRP conjugate | Calbiochem | Cat# 401315 | 1:10000 |
Antibody | Goat-anti-mouse IgG, HRP conjugate | Calbiochem | Cat# 402335 | 1:10000 |
Recombinant DNA reagent | pCR2.1-Topo | Life Technologies | Cat# K4510-20 | PCR cloning vector |
Recombinant DNA reagent | pART7, pART27 | Gleave, 1992 | plant transformationvectors | |
Recombinant DNA reagent | sfGFP | Pédelacq et al., 2006 | used as template for cloning | |
Recombinant DNA reagent | pETDuet1 | Novagen/Merck | Cat# 71146 | for expression of recombinant proteins |
Recombinant DNA reagent | pMS119EH-sfGFP | Pross et al., 2016 | ||
Recombinant DNA reagent | EPI1a, EPI10 | Schardon et al., 2016 | codon-optimized, with plant signal peptide | |
Recombinant DNA reagent | pGreen0229 | Hellens et al., 2000 | plant transformation vector | |
Recombinant DNA reagent | VMA12-mRFP | Viotti et al., 2013 | ER marker | |
Recombinant DNA reagent | VHP1Pro:ManI-mCherry | this paper | Golgi marker; see Materialsand methods section andSupplementary file 2 | |
Recombinant DNA reagent | VHP1Pro:ST-mCherry | this paper | Golgi marker; see Materialsand methods section andSupplementary file 2 | |
Recombinant DNA reagent | Sec | this paper | sfGFP-tagged CLEL6/9expression constructs; Figure 2 | |
Recombinant DNA reagent | KDEL | this paper | sfGFP-tagged CLEL6/9expression constructs withER retention signal; Figure 2 | |
Recombinant DNA reagent | XylT | this paper | sfGFP-tagged CLEL6/9expression constructs withXylT membrane anchor; Figure 2 | |
Recombinant DNA reagent | Secm | this paper | sfGFP-tagged CLEL6/9 expression constructs, SBT6.1 cleavage sitesmutated; Figure 2 | |
Recombinant DNA reagent | Δ-Sec | this paper | sfGFP-tagged CLEL6/9 pro-domain deletion constructs; Figure 3 | |
Recombinant DNA reagent | Δ-KDEL | this paper | sfGFP-tagged CLEL6/9prodomain deletionconstructs with ER retention signal; Figure 3 | |
Recombinant DNA reagent | Δ-XylT | this paper | sfGFP-tagged CLEL6/9 prodomain deletionconstructs with XylTmembrane anchor; Figure 3 | |
Recombinant DNA reagent | SBT3.8-sfGFP | this paper | Expression construct forSBT3.8 C-terminally fusedwith sfGFP; Figure 5 | |
Peptide, recombinant protein | SBT3.8 | this paper | Recombinant, His-taggedSBT3.8, purified from N. benthamiana cell wall extracts | |
Peptide, recombinant protein | CLEL6 | PepMic | DsYPQPHRKPPIHNE | |
Peptide, recombinant protein | CLEL9 | PepMic | DMDsYNSANKKRPIHNR | |
Peptide, recombinant protein | eCLEL6 | PepMic | GEEVVVMDYPQPHRKPPIHNE | |
Peptide, recombinant protein | proCLEL6; CLEL6 precursor | this paper | purified from E. coli BL21-RIL cells | |
Peptide, recombinant protein | CLEL6-D71A | this paper | Site-directed mutant of the CLEL6precursor purified from E. coli BL21-RILcells | |
Peptide, recombinant protein | sulfoCLEL6 | this paper | purified from E. coliBL21-pEVOL SYRScells | |
Commercial assay or kit | Ni-NTA agarose | Qiagen | Cat# 30210 | |
Software, algorithm | GraphPad Prism | Graphpad | preparation of figures andstatistical analyses | |
Software, algorithm | ImageJ | ImageJ | analysis ofgravitropic response |
Additional files
-
Supplementary file 1
List of primers for cloning.
- https://cdn.elifesciences.org/articles/55580/elife-55580-supp1-v1.docx
-
Supplementary file 2
GreenGate modules used including (A) the list of modules, and (B) sources of templates and cloning primers.
- https://cdn.elifesciences.org/articles/55580/elife-55580-supp2-v1.docx
-
Supplementary file 3
Primers used for qPCR analysis.
- https://cdn.elifesciences.org/articles/55580/elife-55580-supp3-v1.docx
-
Transparent reporting form
- https://cdn.elifesciences.org/articles/55580/elife-55580-transrepform-v1.docx