Amphetamine reduces reward encoding and stabilizes neural dynamics in rat anterior cingulate cortex
Figures
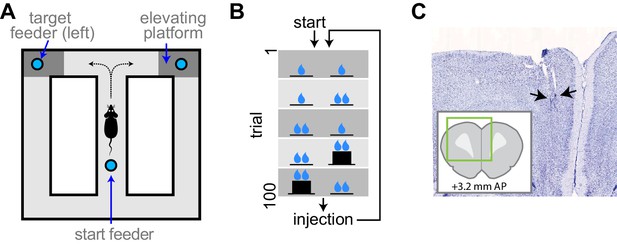
Experimental apparatus and task.
(A) Illustration of the figure 8 maze. The target feeders are elevated on some trials to impart physical effort. (B) Schematic illustration of the task. The reward volume is indicated by the number of drop symbols, and the climbing effort by the black trace of feeder platform height. The sequence of trial blocks (background shading) is repeated after drug injection. (C) Representative sample of a histological section showing the endpoints of electrodes (arrows). The inset show the relative position of the section aligned to a standard rat brain atlas (Paxinos and Watson, 2014).
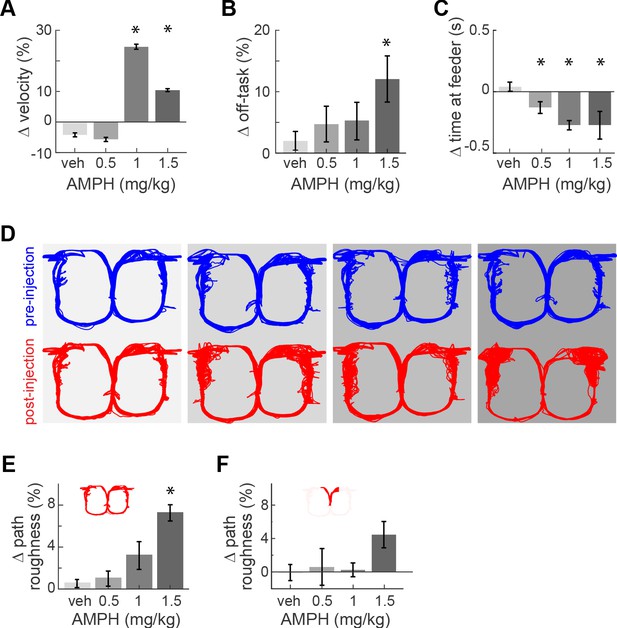
Task performance after AMPH injection.
(A) Change of median running velocity after injection of AMPH with respect to values before injection. (B) Mean change in the relative proportion of trials with off-task behavior. (C) Change in the median time of occupancy at the start feeder. (D) Representative examples of running path superimposed for all trials in one session before (blue) or after (red) injection of vehicle or AMPH. (E) Mean change in running path roughness of all rats for the task epoch from the target feeders back to the start feeder. (F) Change in roughness of running path from the start feeder to target feeders, as measured by mean change in Hausdorff fractal dimension for all rats. Error bars show the Standard Error of the Mean (SEM) or Median (SEMd). Here and following figures: asterisks (*) indicate statistically significant differences at α = 0.05 with Bonferroni correction; only comparisons with saline are illustrated.
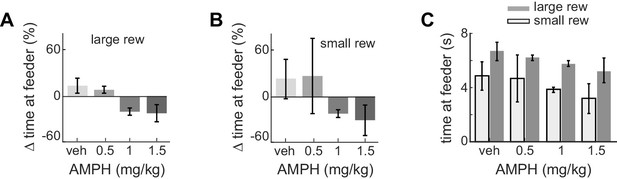
Time at target feeders decreases after AMPH.
(A) Post-injection change in median time at target feeders when given the large reward volume, with respect to the time at feeders for large reward prior to drug. Data show mean and standard error of the mean for the session-wise median for all animals, which significantly reduce as AMPH increases (ANOVA, F3,62 = 3.93, p=0.012). (B) The change in feeder dwell times during small reward after AMPH relative to baseline, computed over all sessions. Time tends to reduce as AMPH increases, but this is not statistically significant because of the large variance at 0.5 mg/kg. (C) Median time spent at feeders after injection for all sessions. Plot shows median and standard error of the median for each session-wise median value. Rats spent less time at feeders during small rewards as compared to large after every dose (Kruskal-Wallis within each dose, α <0.05 after Bonferroni correction).
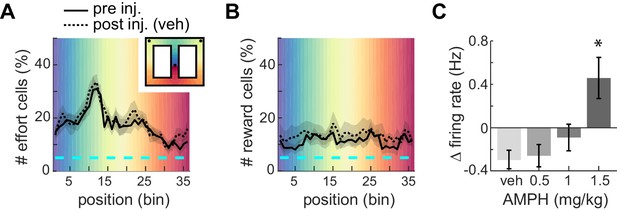
Proportion of ACC neurons encoding effort or reward.
(A) Mean proportion of recorded neurons that discriminate barrier height in pre- or post-injection conditions. The proportion increases up to the barrier traverse. The shaded region surrounding the curves indicates SEM. Background colors correspond to each of the 36 spatial bins of the maze shown in the inset. (B) Mean proportion of recorded neurons responding to the reward. (C) Relative change in mean firing rate after injection (mean and SEM).
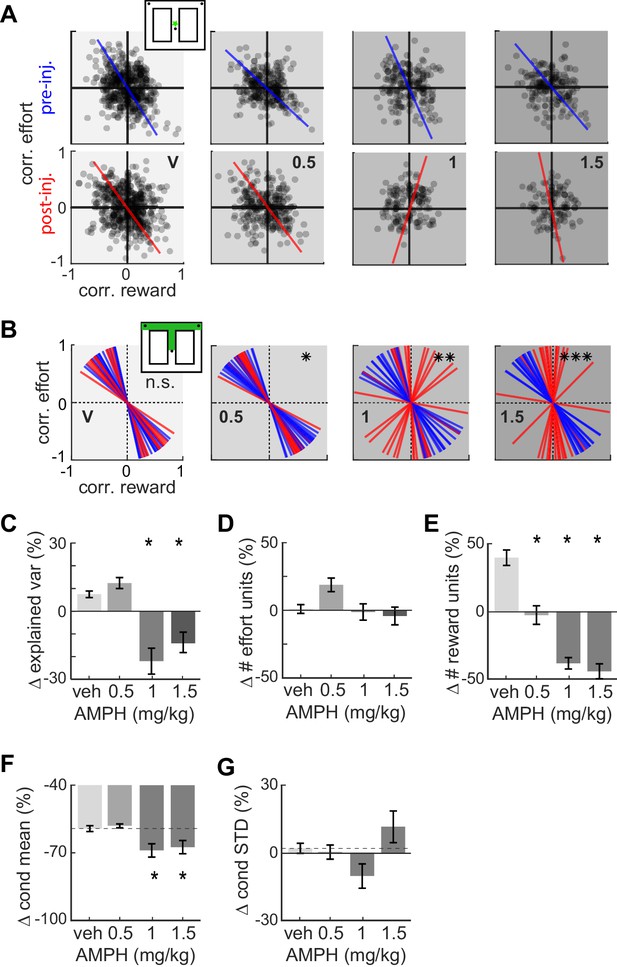
Effect of AMPH on the encoding of reward and effort by single neurons.
(A) Joint effort-reward encoding by ACC neurons in all sessions in the spatial bin immediately following departure from the starting feeder (star in inset). Each black circle shows the Pearson correlation coefficient between the amount of effort and firing rate of one neuron, plotted against the correlation value for reward volume and firing rate of the same neuron. The lines indicate the first principal component (PC) coefficient during pre-injection (blue) and post-injection (red) for all units in the dataset recorded for each dose. (B) First PC of the effort vs reward correlation distribution for cells recorded in all sessions with similar treatment. Each line represents one spatial bin from center feeder to side feeder. Blue lines indicate PCs prior to injection, and red lines indicate the PC for the same cells after the injection. Statistical difference of the coefficient distributions pre versus post injection is computed by the Kuiper two-sample test, and indicated by asterisks: * significant at p<0.05, **: p<0.01, ***: p<0.001. (C) AMPH-evoked changes in mean explained variance by the first PC of effort-reward distributions, showing that the relationship between reward and effort for individual cells breaks down under higher AMPH doses. (D) Post-injection change of the proportion of effort-selective cells, indicating that effort signaling is not reduced by AMPH. (E) Post-injection change in the proportion of reward-selective cells, showing that AMPH decreases reward encoding as measured by the number of cells discriminating reward volume. (F) Post-injection change in the absolute mean difference of firing rate of effort discriminating cells among trials of large vs small reward. The signaling of reward volume is decreased under 1.0 and 1.5 mg/kg. (G) AMPH-related change in the variance of neural firing around the mean firing rate in large and small reward (i.e. class-conditioned variance). The variance is not statistically different under AMPH, but tends to decrease under 1.0 mg/kg and increase under 1.5 mg/kg. Data show mean and SEM. Asterisks (*) indicate statistically significant differences at p<0.05; only comparisons with vehicle are illustrated.
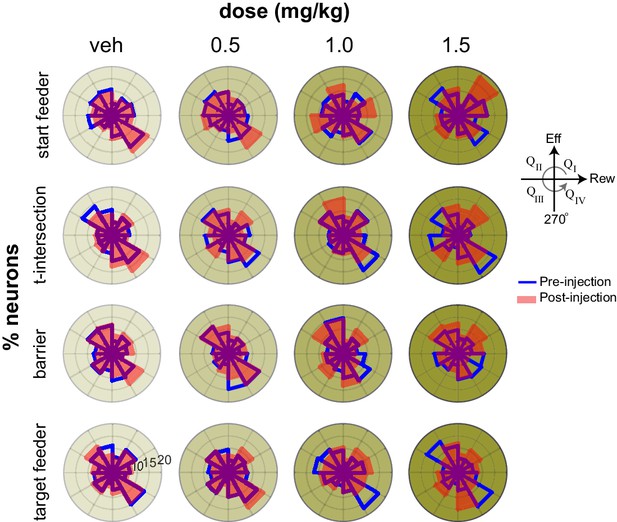
Effort-reward tuning in key task epochs.
Polar histograms of the number of cells in each region of the space defined by the correlation of firing with effort (vertical axis) and correlation of firing with reward (horizontal axis). Prior to injection (blue lines), the population is primarily oriented along the diagonal axis bisecting quadrants QII and QIV (see inset), which signal positive and negative utility, respectively. This tuning is weakest at the barrier, but evident at both feeders as well as the approach and turn at the t-intersection. AMPH diminishes this tuning, as evident by the more uniform distribution of points after drug (red lines).
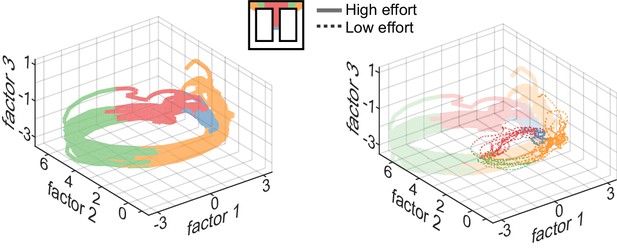
Neural trajectories encode task sequence.
Plots show trajectories of neural encoding projected into low-dimensional space, and are color-coded by the sequential task epochs shown in the inset. Several trials are superimposed, revealing that the patterns of neural activity are distinct for each epoch but are highly similar across trials of the same type. The trajectories diverge for trials requiring different effort (right panel), and the divergence begins well before the actual barrier traverse, which occurs in the green region, as seen by divergence of encoding in the central stem of the track (red region).
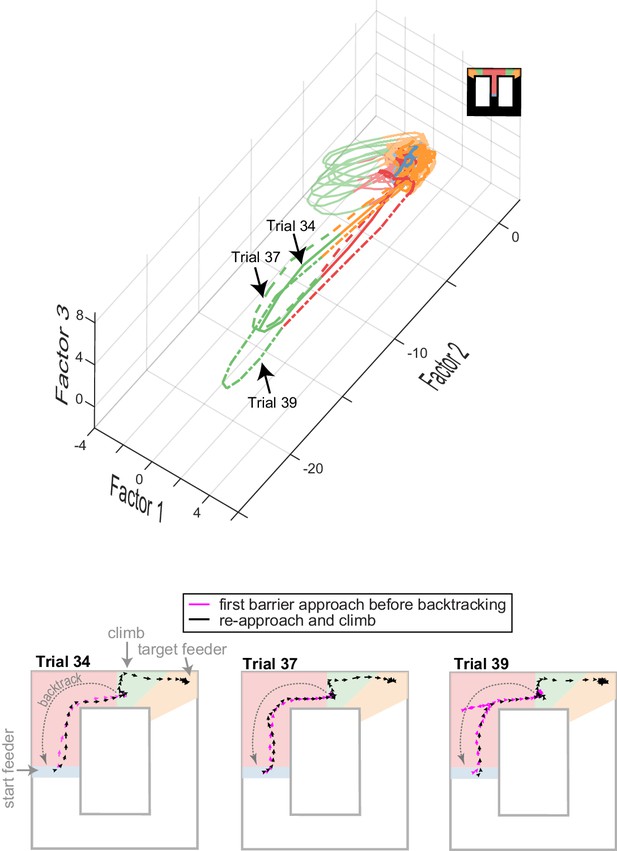
Ensemble encoding is task-specific.
Top plot shows ACC trajectories of population activity in low-dimensional space for several trials in one session, including three trials with off-task behavior (identified by trial numbers). In these cases, the animal approached the barrier, then backtracked from the barrier to the start feeder before returning to commit the climb and reach the target feeder. The running paths and velocity vectors are shown in the lower panels. Note that the neural trajectories deviate largely when the animal is on the second approach and climb (dashed lines in upper panel) as compared to trials with no off-task behavior (solid red-green-orange sequence of lines). This deviation occurs, even though the paths and velocity of the initial approach (fuchsia) and re-approach (black) are similar. This suggests that ACC is sensitive to past events – in this case, off-task behavior.
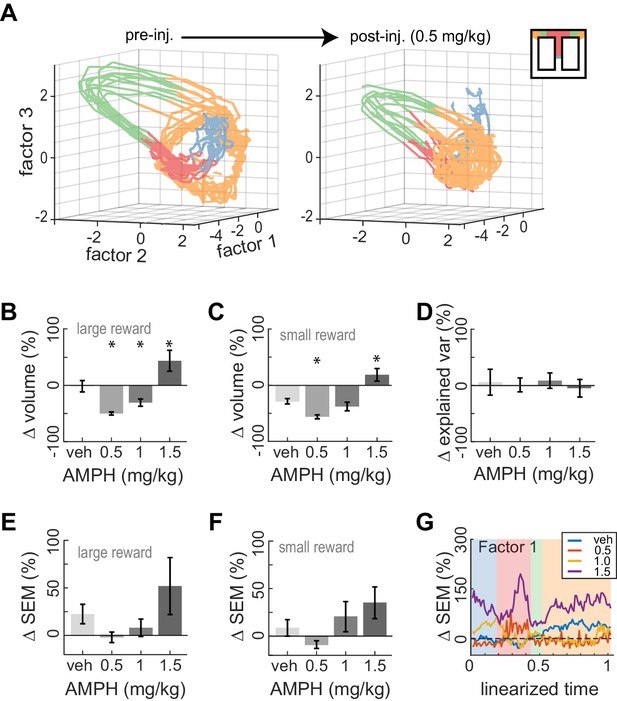
Dose-dependent contraction of neural trajectories.
(A) Example of ACC neural trajectories before injection (left panel), and after injection of 0.5 mg/kg AMPH (right panel) plotted into the same low-dimensional space for one session. The volume enclosed by the envelope (outer hull) of the trajectories contracts after AMPH. (B) Post-AMPH changes in mean trajectory volume over all sessions and trials with large reward. The volume decreases for intermediate doses, and expands at the highest dose. (C) The same dose-dependent volume changes occur for small-reward trials. (D) Changes in the amount of variance explained by the first three factors after AMPH. Medians and 95% confidence intervals are shown, revealing that explained variance is not significantly reduced after AMPH because the confidence intervals encompass 0. (E) Change in SEM of neural trajectories after AMPH on large reward trials averaged over all the first three factors and all spatial bins, showing a reduction in trial-by-trial variance for low-dose AMPH. (F) Same as panel E for small reward trials. (G) Change in SEM for the first factor across task epochs. Data are averaged over all sessions with similar treatment for large reward trials. Asterisks (*) indicate statistically significant differences at p<0.05; only comparisons with vehicle are illustrated. Panels B, C, E, and F show means and SEM.