Dynamics of gaze control during prey capture in freely moving mice
Figures
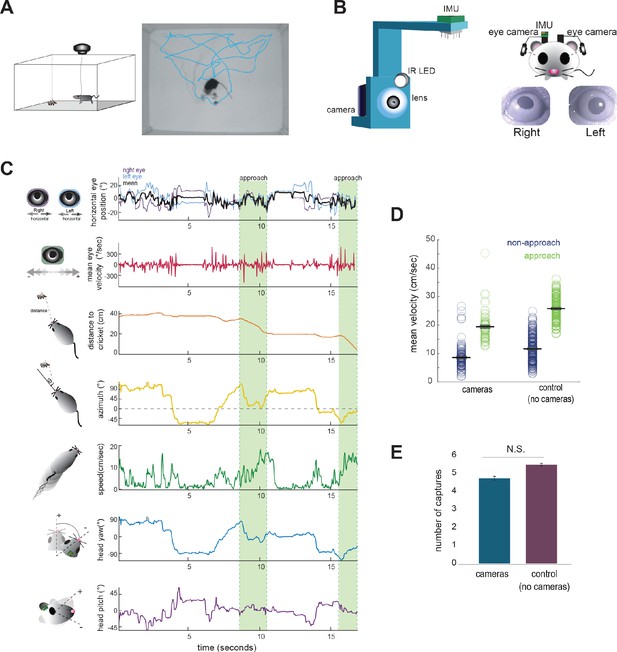
Tracking eye and head movements during prey capture.
(A) Unrestrained mice hunted live crickets in a rectangular plexiglass arena (45 × 38 × 30 cm). Using an overhead camera, we tracked the movement of the mouse and cricket. Example image with overlaid tracks of the mouse (cyan). (B) 3D printed holders house a miniature camera, collimating lens, an IR LED, and an IMU, and are reversibly attached to implants on the mouse’s head, with one camera aimed at each eye. (C) Synchronized recordings of measurements related to bilateral eye position and velocity, mouse position relative to cricket (distance and azimuth, as measured relative to the center of the head), mouse speed, and head rotation in multiple dimensions (analysis here focuses on yaw and pitch). (D) Average mouse locomotor speed did not differ across experimental and control experiments (no camera and IMU) for both non-approach and approach periods. Individual dots represent the average velocity per trial. (E) Average number of captures per 10 min session did not differ between experimental and control sessions (control N = 7 animals, 210 trials; cameras N = 7 animals, 105 trials; two-sample t-test, p=0.075).
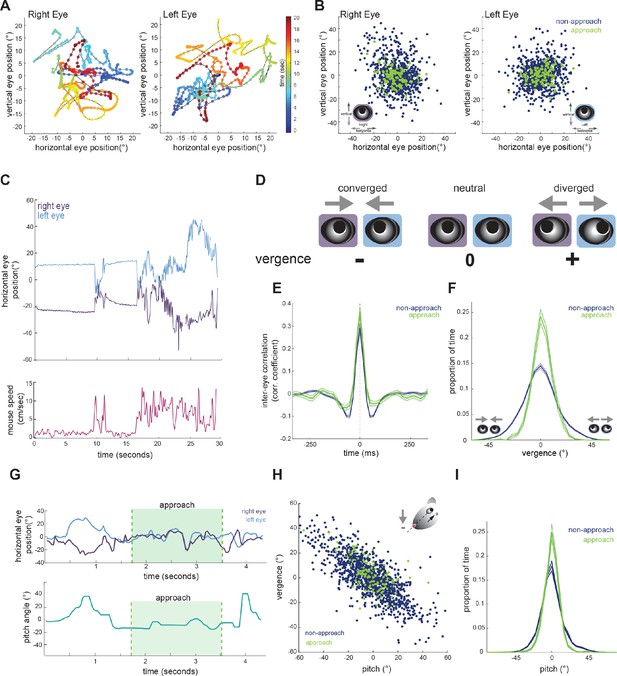
Eye position is more aligned across the two eyes during approach periods.
(A) Example eye movement trajectory for right and left eyes for a 20 s segment, with points color-coded for time. (B) Horizontal and vertical position for right and left eyes during approach and non-approach times. N = 7 animals, 105 trials, 792 time pts (non-approach), 110 time pts (approach), representing a random sample of 0.22% of non-approach and 0.52% of approach time points. (C) Example trace of horizontal eye positions (top) and running speed (bottom) for a 30 s segment. (D) Schematic demonstrating vergence eye movements. (E) Cross correlation of horizontal eye position across the two eyes for non-approach and approach periods. (F) Histogram of vergence during non-approach and approach. (G) Example trace of horizontal eye position (top) and head pitch (bottom) before, during, and after an approach. (H) Scatter plot of head pitch and eye vergence. As head pitch tilts downwards, the eyes move temporally to compensate (as in schematic). N = 7 animals, 105 trials, 1240 time points (non-approach), 132 time points (approach), representing a sample of 0.35% of non-approach and 0.63% of approach time points. (I) Histogram of head pitch during approach and non-approach periods, across all experiments.
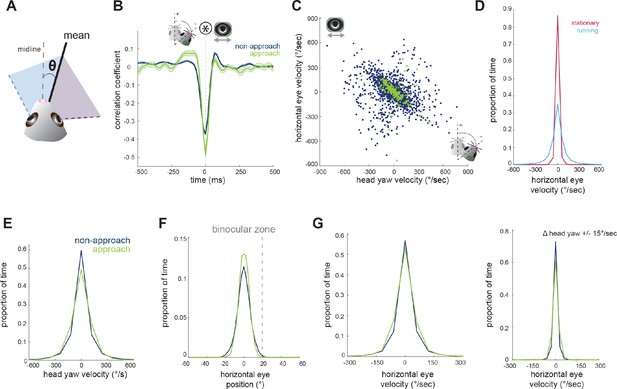
Horizontal eye movements are mostly compensatory for yaw head rotations.
(A) To remove the effect of non-conjugate changes in eye position (i.e. vergence shifts), we compute the average angular position of the two eyes. (B) Cross-correlation of change in head yaw and horizontal eye position. (C) Scatter plot of horizontal rotational head velocity and horizontal eye velocity. N = 7 animals, 105 trials, 3565 (non-approach) and 211 (approach) timepoints, representing 1% of non-approach and 1% of approach timepoints. (D) Distribution of horizontal eye position during stationary and running periods (defined as times when mouse speed is greater than 1 cm/sec; Kolmogorov-Smirnov test, p=0.032). (E) Distribution of head angle velocity (paired t-test, p=0.938). (F) Distribution of mean absolute eye position (paired t-test, p=0.156). (G) Distribution of horizontal eye velocity (paired t-test, p=0.155) and distribution of eye velocity when head yaw is not changing (change in head yaw between ±15 deg/sec; paired t-test, p=0.229; N = 7 animals, 105 trials).
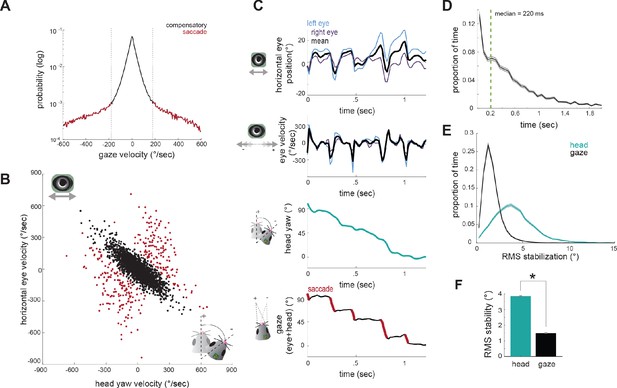
Compensatory and non-compensatory eye movements generate a saccade-and-fixate gaze pattern during head turns.
(A) Distribution of gaze velocity (N = 377459 time points) showing segregation of non-compensatory and compensatory movements with thresholds at ±180°/sec. (B) Joint distributions of head yaw and horizontal eye velocity colored by their type as defined in A. Black points represent compensatory movements and red represent non-compensatory saccadic movements. Points shown are a random sample of 2105 approach timepoints, 10% of total approach time points. (C) Example traces of horizontal eye position, head yaw, and gaze demonstrate a saccade-and-fixate pattern in gaze. (D) Histogram of fixation duration; fixations N = 9730, 105 trials. (E) Root Mean Squared (RMS) stabilization histograms for head yaw and gaze. (F) Bar graphs are medians of RMS stabilization distributions (median head = 3.87 deg; median gaze = 1.5 deg; paired t-test, p=0).
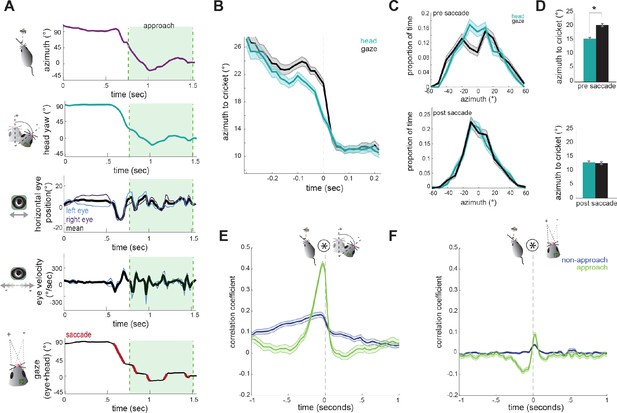
Head angle tracks cricket position more accurately than gaze position.
(A) Example traces of horizontal eye position, azimuth to cricket, head yaw, and gaze demonstrate a saccade-and-fixate pattern in gaze before and during an approach period. The head is pointed directly at the cricket when azimuth is 0°. Note the rapid decrease in azimuth, head yaw, and mean horizontal eye position creating a saccade immediately preceding the start of approach. (B) Average head yaw and gaze around the time of saccade as a function of azimuth to the cricket. Time = 0 is the saccade onset. (C) Histograms of head yaw and gaze position before and after saccades occur. (D) Medians of yaw and gaze distributions from C (paired t-test, ppre saccade=8.48x10−9; ppost saccade=0.979). (E) Cross correlation of azimuth and change in head yaw for non-approach and approach periods. (F) Cross correlation of azimuth and change in gaze for non-approach and approach periods. N = 105 trials, 7 animals.
Videos
Video of mouse performing prey capture with reversibly attached eye cameras, demonstrating synchronized measurement of bilateral eye positions and mouse/cricket behavior.
The direction of each eye is superimposed on the head (purple and light blue lines) based on calculated eye position and head angle.
Video of mouse performing prey capture, demonstrating dynamics of head orienting (dark blue) and gaze direction (cyan).
Note that during head turns the gaze is transiently offset from the head angle vector, due to compensatory eye movements, creating a stable image for the animal. Then, non-compensatory saccades shift the gaze position such that it aligns with the head to accurately target the cricket.
Tables
Reagent type (species) or resource | Designation | Source or reference | Identifiers | Additional information |
---|---|---|---|---|
Strain, strain background (Mus musculus) | C57Bl/6J | JAX | JAX: 000664 | Wild type animals |
Software, algorithm | Matlab | Matlab | Matlab R2020a | |
Software, algorithm | DeepLabCut | Mathis et al., 2018 | ||
Software, algorithm | Bonsai | Lopes et al., 2015 |