Cortical ChAT+ neurons co-transmit acetylcholine and GABA in a target- and brain-region-specific manner
Figures
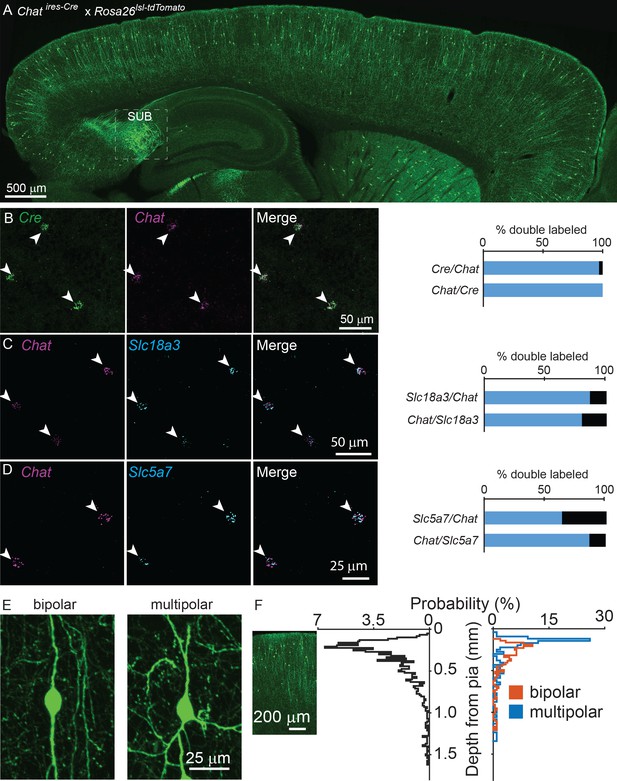
Cortical ChAT+ neurons are present throughout cortex and express genes necessary for synthesis and release of ACh.
(A) Sagittal view of a mouse neocortex with ChAT+ neurons expressing tdTomato (Chatires-Cre x Rosa26lsl-tdTomato) demonstrating the distribution of putative cholinergic neurons throughout the cortex. Strongly tdTomato-labeled neurons in the subiculum that do not express Chat are labeled (SUB). (B) Flourescent in situ hybridization of Cre faithfully reports Chat expression in Chatires-Cre mice in the cortex. Arrow heads indicate dual Chat+/Cre+ neurons. Quantification shown at right (n = 32 Chat+/Cre+ of 33 Chat+ and 32 Cre+ neurons from 2 Chatires-Cre mice). (C,D) Fluorescent in situ hybrization of Chat in cortex co-labels with Slc18a3, the gene encoding VAChT (n = 147 Chat+/Slc18a3+ of 170 Chat+ and 184 Slc18a3+ neurons from 3 wild-type mice) and Slc5a7, the gene encoding the membrane choline transporter (n = 72 Chat+,Slc5a7+ of 113 Chat+ and 83 Slc5a7+ neurons from 3 wild-type mice). Arrowheads indicate cortical ChAT+ neurons and quantification shown at right. (E) Cortical ChAT+ neurons are vertically oriented and are bipolar (left) or multipolar (right). (F) Distribution of cortical depth from the pia of all cortical ChAT+ neurons (left graph, black trace, n = 1059 neurons from 3 Chatires-Cre x Rosa26lsl-tdTomato mice), median cell body is 274 µm from pia ±15 µm, 95% C.I.) and according to morphology (right graph; orange = bipolar, n = 207, 66% of total, median 293 µm from pia ±23 µm, 95% C.I.; blue = multipolar, n = 107 neurons, 34% of total, median 173 µm from pia ±24 µm, 95% C.I.). Inset image is aligned to the relative depth shown in the graphs.
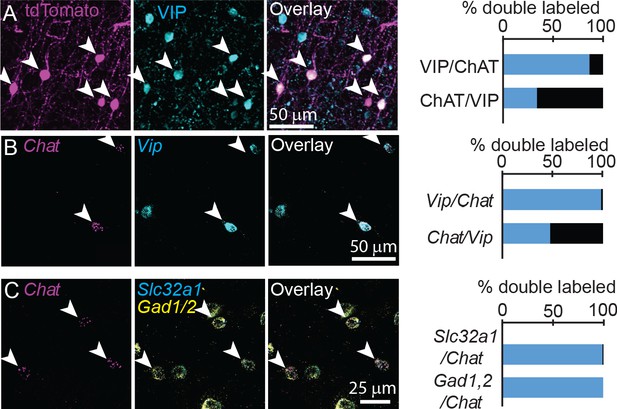
Cortical ChAT+ neurons are a subset of VIP+ interneurons and express genes necessary for synthesis and release of GABA.
(A) Cortical ChAT+ neurons expressing tdTomato (Chatires-Cre x Rosa26lsl-tdTomato) co-label with immunostained VIP (n = 127 ChAT+/VIP+ neurons of 147 total ChAT+ and 375 VIP+ neurons from 3 Chatires-Cre x Rosa26lsl-tdTomato mice). (B) Fluorescent in situ hybridization of Chat in cortex co-labels with Vip (n = 278 Chat+/Vip+ of 283 Chat+ and 579 Vip+ neurons from 3 wild-type mice). (C) Fluorescent in situ hybridization labeling of Chat in cortex co-labels with the GABAergic genes Slc32a1, which encodes for VGAT, and Gad1 and Gad2, which encodes for the GABA synthetic enzymes GAD67 and GAD65, respectively (n = 101 Chat+,Slc32a1+ and 102 Chat+,Gad1/2+ of 102 Chat+ neurons from 5 wild-type mice). Arrowheads indicate double labeled neurons.
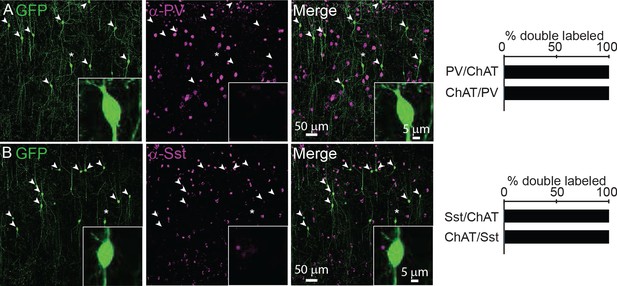
Cortical ChAT+ neurons do not express Parvalbumin or Somatostatin.
(A) Cortical ChAT+ neurons, labeled by injecting AAV(8)-DIO-EGFP into the frontal cortex of Chatires-Cre mice, do not co-label with immunostained PV (n = 1 ChAT+,PV+ of 180 CHAT+ and 576 PV+ neurons from 3 Chatires-Cre mice). (B) Cortical ChAT+ neurons, labeled as above, do not co-label with immunostained Sst (n = 2 ChAT+/Sst+ of 360 ChAT+ and 1016 Sst+ neurons from 3 Chatires-Cre mice). Arrowheads indicate GFP-expressing ChAT+ neurons and asterisks indicate cells shown in the insets.
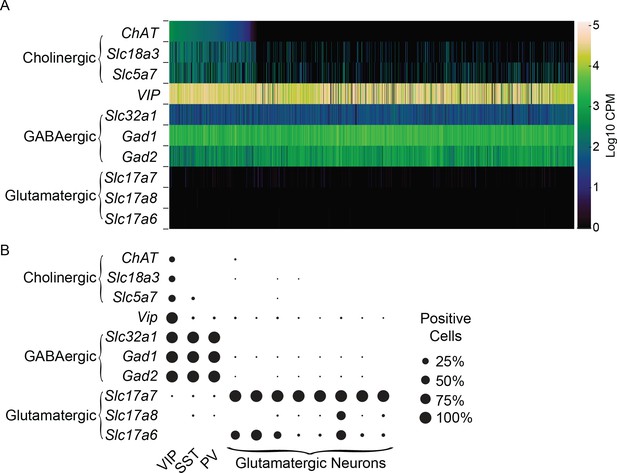
A subset of VIP+ interneurons express cholinergic genes by single-cell RNA sequencing.
(A) Heat map of the number of transcripts per cell (log counts per million, Log10 CPM) for various neurotransmitter synthesis and vesicular release machinery genes from 2952 single-cell transcriptomes of Vip-expressing neurons from visual cortex and anterior lateral motor cortex. Cells are ordered from left-to-right according to their expression of Chat. Data is downloaded from the Allen Brain Institute’s RNA-Seq Data Navigator, accessible at: http://celltypes.brain-map.org/rnaseq/mouse. (B) The proportion of cells in different cell sub-types that are positive for different transcripts for genes indicating different neurotransmitter phenotypes, as defined by >1 log10 CPM of each transcript.
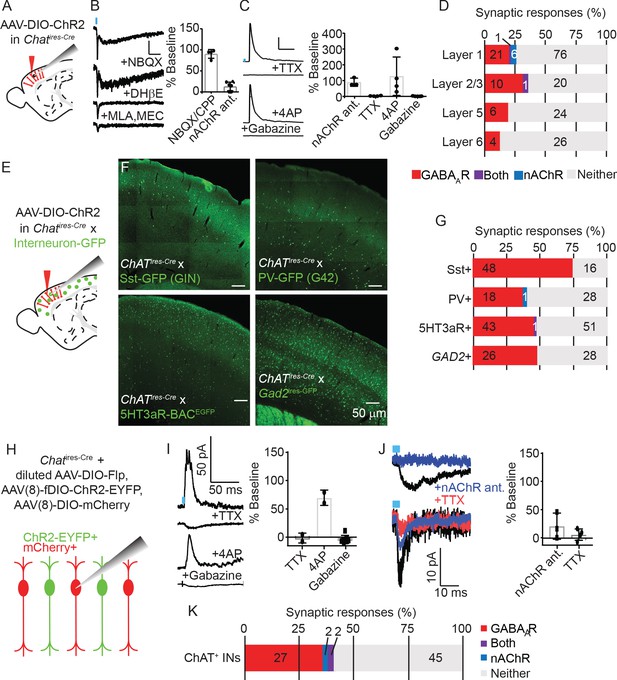
Cortical VIP+/ChAT+ interneurons primarily release GABA onto inhibitory interneurons and sparsely release ACh onto layer 1 and other ChAT+ neurons.
(A) Experimental design: Cre-dependent ChR2-mCherry is virally delivered (AAV(8)-DIO-ChR2-mCherry) to the cortex, expressed for 3 weeks, and whole-cell voltage clamp recordings obtained from unlabeled neurons. Recordings from motor cortex and visual cortex are pooled for panels A-D. (B) Examples trace of a biphasic nAChR-mediated synaptic currents isolated by voltage clamping the post-synaptic neuron at −70 mV and stimulating cortical ChAT+ neurons with 3 ms of 473 nm light (~7–9 mW/cm2). The synaptic current is insensitive to AMPA receptor antagonist NBQX, but the slow component is blocked by DHβE, selective for ɑ4 receptor subunits, and the fast component is blocked by the ɑ7-selective antagonist MLA and pan-nAChR antagonist MEC. Right panel shows summary quantification of sensitivity to glutamatergic antagonists NBQX/CPP and nAChR antagonists DHβE, MLA, and MEC. Error bars show mean +/- s.e.m. (C) Example GABAA receptor-mediated currents isolated by voltage clamping the post-synaptic neuron at 0 mV. Synaptic currents are blocked by voltage-gated sodium channel antagonists TTX, rescued by subsequent application of potassium channel antagonists 4AP, and further blocked by GABAAR-selective antagonist gabazine. Right panel shows summary quantification of the effects of nAChR antgonists, TTX, 4AP, and gabazine on inhibitory currents. (D) Summary of the proportion of neurons showing synaptic responses following optogenetic stimulation of cortical ChAT+ neurons across cortical layers. The numbers in each bar indicate the number of cells in each category. (n = 195 total neurons from 24 Chatires-Cre mice; p=0.8627 for layer 1 compared to non-layer 1 GABAAR-mediated responses and p=0.0695 or layer compared to non-layer1 nAChR-mediated responses, Fisher’s exact test). (E) Experimental design: AAV(8)-DIO-ChR2-mCherry was injected into the motor cortex of Chatires-Cre mice crossed to different mouse lines that express GFP in specific interneuron subpopulations. (F) Example images showing GFP expression in 4 different mouse lines expressing GFP in different interneuron subtypes. (G) Summary quantification of the proportion of cells of each interneuron subtype that had synaptic responses to optogenetic stimulation of cortical ChAT+ neurons. The numbers in each bar indicate the number of cells in each category. (n = 64 GFP+ cells from 6 Chatires-Cre x Sst-GFP (GIN) mice; n = 47 GFP+ cells from 4 Chatires-Cre x PV-GFP (G42) mice; n = 95 GFP+ cells from 6 Chatires-Cre x 5HT3aR-BACEGFP mice; n = 54 GFP+ cells from 4 Gad2ires-GFP mice; p=0.0307 for differences in GABAAR-responses between interneuron types, p=0.5775 for differences in nAChR-responses, Pearson’s chi-squared test). (H) Experimental design: To achieve mosaic expression of ChR2 in a subset of cortical ChAT+ neurons, we injected a diluted AAV(8)-DIO-FlpO virus so that a subset would express Flp. We then injected with high-titer AAV(8)-fDIO-ChR2-EYFP and AAV(8)-DIO-mCherry. We targeted mCherry+, EYFP- cells for whole-cell voltage clamp recording that neighbored EYFP+ neurons. (I) Example traces showing putative GABAAR-mediated synaptic response at baseline and following application of TTX, 4AP, and gabazine. Right panel shows summary quantification of block by TTX, rescue by 4AP, and block by gabazine. Error bars show mean ± s.e.m. (J) Example traces of two different neurons showing nAChR-mediated responses and their block by nAChR antagonists and TTX. These two cells have different response kinetics, potentially indicative of extra-synaptic (top) and synaptic (bottom) nAChRs. Right panel shows summary quantification of putative nAChR-mediated synaptic response sensitivity to nAChR antagonists (MEC, MLA, and DHβE) and TTX. (K) Summary quantification of the proportion of cortical ChAT+ neurons that showed synaptic responses following stimulation of neighboring ChR2-expressing cells. The numbers in each bar indicate the number of cells in each category (n = 76 neurons from 8 Chatires-Cre mice).

GABAAR-mediated synaptic currents from VIP+/ChAT+ interneurons are mono-synaptic.
(A–D). Synaptic response amplitudes, sensitivity to TTX, 4AP, and gabazine, and laminar distribution for GFP-expressing post-synaptic neurons in Sst-GFP (GIN) mice (A), PV-GFP (G42) mice (B), 5HT3aRires-GFP mice (C), and Gad2ires-GFP mice (D). Left: mean response amplitude. Middle left: Quantification of sensitivity to TTX, 4AP, and gabazine. All synaptic responses are blocked by TTX, substantially rescued by 4AP, and completely abolished by gabazine. Middle right: example traces showing synaptic response amplitude following consecutive application of TTX, 4AP, and gabazine. Right: Laminar distribution of recorded neurons. Numbers indicate the number of cells in each category. Total number of neurons and mice are the same as Figure 3.
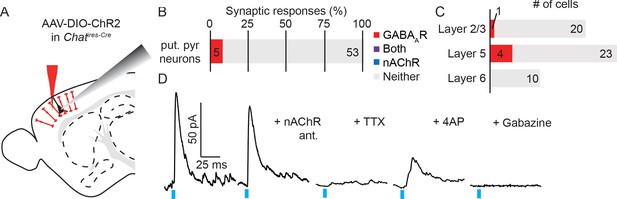
Cortical VIP+/ChaT+ neurons have a low rate of connectivity to putative pyramidal neurons.
(A) Experimental design: AAV(8)-DIO-ChR2 was injected into the motor cortex of Chatires-Cre mice. Following 3–4 weeks to allow for virus expression, whole-cell voltage clamp recordings of pyramidal neurons were made from acute sagittal slices. Pyramidal neurons were identified based on their morphology and laminar position. (B) Summary quantification of the proportion of pyramidal neurons with synaptic responses to optogenetic stimulation of VIP+/ChAT+ neurons. The number of cells per category are indicated. (n = 58 neurons from 14 Chatires-Cre mice). (C) Laminar distribution of recorded putative pyramidal neurons. The number of cells per lamina are indicated. (D) Example GABAAR-mediated synaptic current, which is insensitive to nAChR antagonists DHβE, MLE, and MEC, is blocked by TTX and subsequently rescued by 4AP, and completely blocked by gabazine.
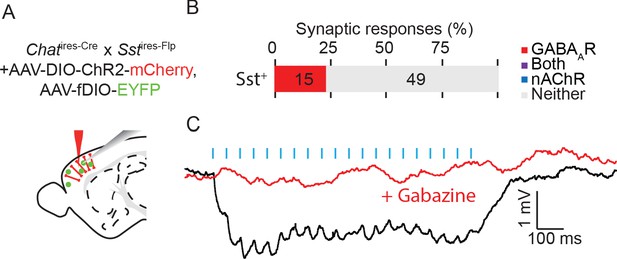
Cortical VIP+/ChAT+ neurons inhibit deep layer Sst+ interneurons.
(A) Experimental design: Because the Sst-GFP (GIN) BAC transgenic mouse line does not express in deeper layer Sst+ interneurons, we targeted Sst+ interneurons in Layer 5 and Layer 6 by crossing Chatires-Cre mice with Sstires-flp mice and injecting with a AAV(8)-DIO-ChR2-mCherry and AAV(DJ)-fDIO-EYFP. After 3–4 weeks to allow expression of viral genes, whole-cell current clamp recordings were obtained from EYFP+ neurons in layers 5 and 6. Current clamp recordings were obtained with a potassium-based internal recording solution to enable detection of muscarinic ACh receptor-mediated currents. (B) Summary quantification of the proportion of cells showing synaptic responses following trains of optogenetic stimulation (3 ms pulses of 473 light, 20 × 20 Hz) of VIP+/ChAT+ interneurons. GABAergic responses were identified based on hyperpolarizing post-synaptic potentials and sensitivity to gabazine. The number of neurons in each category are indicated (n = 74 EYFP+ neurons from 8 Chatires-Cre x Sstires-Flp mice). (C) Example synaptic response to a train of optogenetic stimulation showing hyperpolarization that is blocked by gabazine.
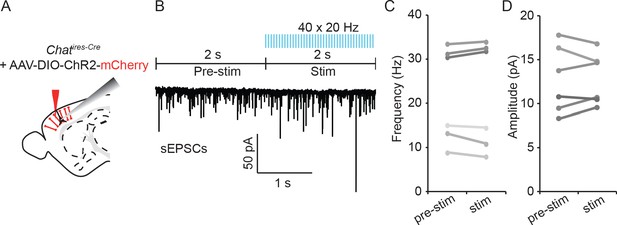
Cortical VIP+/ChAT+ interneuron have no effect on spontaneous excitatory post-synaptic currents in pyramidal neurons.
(A,B) Experimental Paradigm: AAV(8)-DIO-ChR2-mCherry was injected into the motor cortex of Chatires-Cre mice and acute sagittal slices cut after 3–4 weeks. Spontaneous excitatory post-synaptic currents (sEPSCs) were recorded during whole-cell voltage clamp of Layer 2/3 pyramidal neurons. Several seconds of baseline sEPSCs were gathered, followed by 2 s of optogenetic stimulation of ChR2+ neurons (40 × 20 Hz, 3 ms pulses). (C) sEPSC frequency (left) and amplitude (right) before and during stimulation (n = 5 neurons from 2 Chatires-Cre mice).

ACh release from VIP+ interneurons is not necessary for neurovascular coupling.
(A) Example images of pial arteries imaged in vivo over barrel cortex following retro-orbital injection of Alexa 633 hydrazide both prior to (left), immediately following (middle), and several seconds following (right) 3 second stimulation of the contralateral whiskers. (B) Kymograph of the red line illustrated in (A) showing the width of the artery over time. (C) Average percent change in pial artery diameter imaged over the barrel cortex of from 3 mice lacking VAChT in VIP+ interneurons (Vipires-Cre x Slc18a3fl/fl) and 3 heterozygous or wild-type littermate controls during contralateral stimulation of whiskers. (D) Average percent change in pial artery diameter imaged over the barrel cortex during optogenetic stimulation (5 × 20 hz, 5 ms pulses) from 3 mice lacking VAChT in VIP+ interneurons (Vipires-Cre x Rosa26lsl-ChR2-EYFP x Slc18a3fl/fl), 2 heterozygous littermate controls (Vipires-Cre x Rosa26lsl-ChR2-EYFP x Slc18a3fl/wt), and 2 wild-type controls that do not express ChR2.
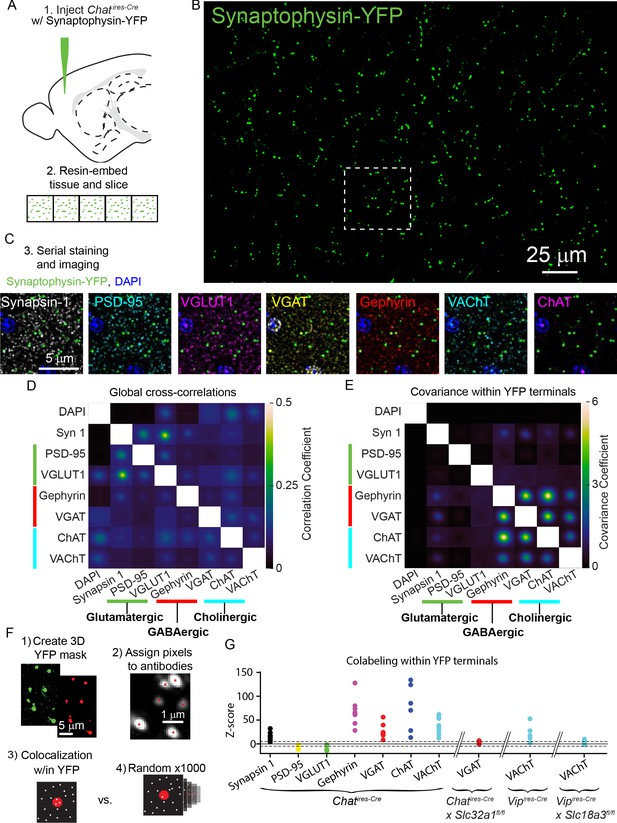
ACh and GABA synthesis and release machinery are both expressed in the presynaptic terminals of cortical ChAT+ interneurons.
(A) Array tomography workflow: Chatires-Cre mice are injected with Cre-dependent Synaptophysin YFP virus (AAV(8)-DIO-Synaptophysin-YFP) to label the pre-synaptic terminals of cortical ChAT+ neurons (1).~1 mm2 squares of tissue were then embedded in resin and cut into 70 nm slices in an array (2). (B) Example maximum-projection of Synaptophysin-YFP staining. (C) The ribbons of tissue are serially stained with antibodies against pre- and post-synaptic proteins (3). Example images show the inset from (B) to demonstrate the staining of pre-synaptic marker Synapsin 1, glutamatergic markers PSD-95 and VGLUT1, GABAergic markers VGAT and Gephyrin, and cholinergic markers VAChT and ChAT. (D) The average cross-correlation between all pairs of raw images of pre- and post-synaptic antibody stains (n = 8 image stacks from 3 Chatires-Cre mice). (F) The average co-variance between all pairs of raw images specifically within a mask created by the Synaptophysin-YFP stain. Co-variance within YFP terminals is not limited to values between −1 and 1 because all antibody stains were z-scored prior to masking and calculating the co-variance, and therefore antibody signals may be more or less concentrated within the YFP mask (n = 8 image stacks from 3 mice). High covariance specifically between GABAergic and cholinergic proteins emerge when limiting analysis to signal within YFP masks (E), but not the entire image, where correlations between glutamatergic markers are highest (D). (F) Summary of antibody colocalization analysis. First, a 3D mask of the YFP signal is created corresponding to the ChAT+ presynaptic terminals. Next, each punctum of antibody signal is assigned a pixel corresponding to where a Gaussian fit of fluorescence has the highest intensity. Then, the colocalization of each antibody pixel within the YFP terminals is determined and a z-score calculated by comparing to the colocalization from 1000 rounds of randomized antibody pixel locations. (G) Colocalization z-scores across antibodies for all samples. Higher positive z-scores indicate relative enrichment of antibody puncta within YFP terminals compared to randomized controls, while negative scores indicate depletion of antibody puncta within YFP terminals (see Figure 4—figure supplement 2). Tissue samples from Chatires-Cre mice are shown, as well as the VGAT antibody z-score from VGAT conditional knock-out mice (6 image stacks from 3 Chatires-Cre x Slc32a1fl/fl mice), and VAChT antibody z-scores from Vipires-Cre (5 image stacks from 3 mice) and Vipires-Cre x Slc18a3fl/fl (6 image stacks from 3 mice). Dashed lines indicate ± 5 z-scores.
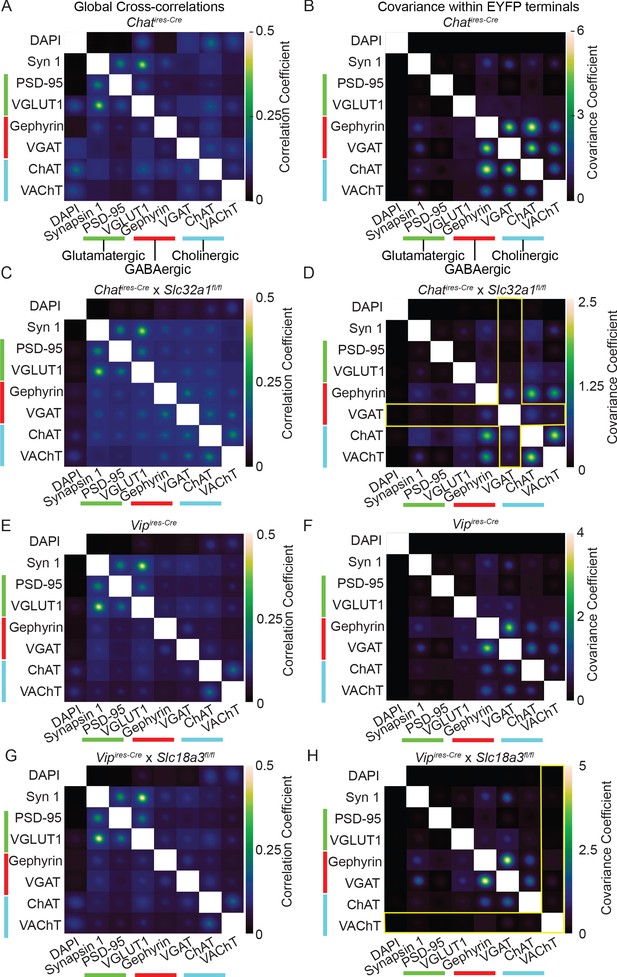
Average cross-correlations of synaptic antibody image arrays from Chatires-Cre, VGAT mosaic knock-out (Chatires-Cre x Slc32a1fl/fl), Vipires-Cre, and VAChT mosaic knockout mice (Vipires-Cre x Slc18a3fl/fl).
(A,B) Global cross-correlations (A) and co-variances within YFP masks of VIP+/ChAT+ pre-synaptic terminals (B) of tissue collected from Chatires-Cre mice injected with AAV(8)-DIO-Synaptophysin-YFP. These graphs are duplicated from Figure 2D & E. (C,D) To test the specificity of the VGAT antibody for VIP+/ChAT+ terminals, we repeated the analysis from Figure 2 in tissue from Chatires-Cre x Slc32a1fl/fl mice, and found that VGAT staining is no longer correlated with Gephyrin or the cholinergic markers ChAT and VAChT. Yellow bars in (D) highlight the loss of VGAT correlations within VIP+/ChAT+ terminals compared to (B). (E,F) To confirm the specificity of VAChT staining, we selectively deleted VAChT in VIP+ interneurons (Vipires-Cre x Slc18a3fl/fl). We therefore first tested the global cross-correlations and YFP-masked co-variances of tissue collected from Vipires-Cre mice injected with AAV(8)-DIO-Synptophysin-YFP, and saw similar patterns of enrichment for GABAergic and Cholinergic markers as in (B). (G,H) In contrast to tissue from Vipires-Cre mice, global cross-correlations and YFP-masked co-variances of tissue collected from mice with VAChT deleted from all VIP+ interneurons (Vipires-Cre x Slc18a3fl/fl) show that VAChT is no longer correlated with ChAT or GABAergic markers. Yellow bars in (H) highlight the loss of VAChT correlations within cortical VIP+ terminals compared to (F). Total number of image stacks and mice are the same as in Figure 4.

Summary antibody colocalization with VIP+/ChAT+ pre-synaptic terminals.
(A–C) Illustration of antibody colocalization analysis. (A) A 3D mask is created from the YFP signal corresponding to ChAT+ presynaptic terminals, and individual pixels are assigned to each punctum of antibody signal according to the location of peak intensity of a Gaussian fit of the raw antibody fluorescence. (B) The colocalization of the binary antibody puncta and YFP masks is then determined as the percent of YFP pixels that overlap with antibody pixels, and compared both to the colocalization within expanding concentric areas around the YFP masks and to 1000 rounds of randomized antibody puncta locations. (C) Example of the colocalization of Synapsin 1 within the YFP masks compared to the region surrounding the YFP masks and to the randomized antibody locations. A z-score is taken by subtracting the random level of colocalization from the actual colocalization and dividing by the standard deviation of the randomized trials. (D) Example graphs from a single sample showing the colocalization of PSD-95, VGLUT1, Gephyrin, VGAT, ChAT, and VAChT within and around the YFP masks. (E–H) Summary z-scores of colocalizatoin both within (left panels) and around (right panels) the YFP mask across samples. Data are shown from Chatires-Cre mice (n = 8 samples from 3 mice) to show the baseline colocalization in cortical ChAT+ interneurons and from mice with VGAT deleted from cholinergic neurons (Chatires-Cre x Slc32a1fl/fl; n = 6 from 3 mice). Loss of VGAT enrichment is highlighted in red. Colocalization is also shown for cortical VIP+ interneurons from Vipires-Cre mice (n = 5 from 3 mice) and from mice with VAChT deleted from VIP+ interneurons (Vipires-Cre x Slc18a3fl/fl; n = 6 from 3 mice). Loss of VAChT enrichment is highlighted in red. Portions of this figure are duplicated from Figure 4.
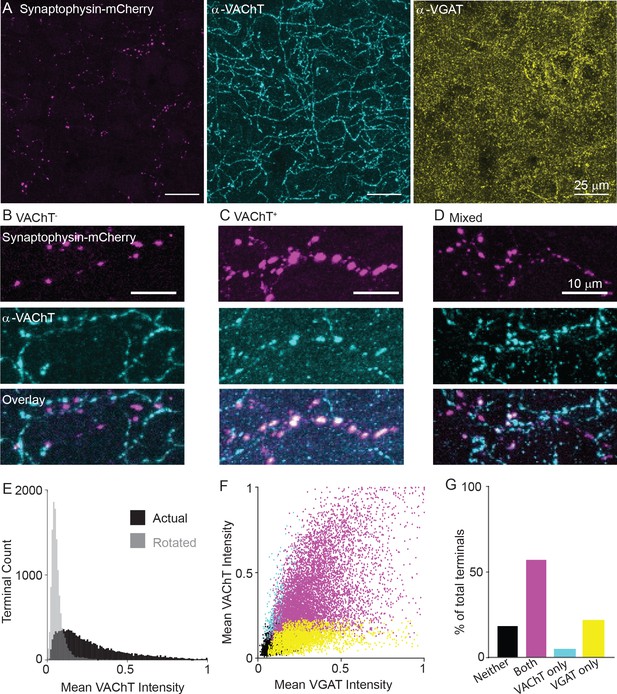
Variable expression of VAChT in the presynaptic terminals of cortical ChAT+ neurons.
(A) Example images of cortical ChAT+ pre-synaptic terminals labeled with AAV(8)-DIO-Synaptophysin-mCherry injected in the motor cortex of Chatires-Cre mice. Left: Synaptophysin mCherry; Middle: VAChT immunostain; Right: VGAT immunostain. (B–D) Example images showing putative Synaptophysin-mCherry+ axons, with VAChT- terminals (B), VAChT+ terminals (C), and intermingled terminals that are both VAChT+ and VAChT-. (D). (E) Histogram of mean VAChT fluorescence intensity within Synaptophysin-mCherry+ terminals. Black histogram represent the actual VAChT intensities, grey histogram represents the mean VAChT intensities when the mCherry image mask is rotated 90° relative to the VAChT immunostain image. (F) Scatter plot of mean VGAT intensity and VAChT intensity in each putative pre-synaptic terminals (n = 12,356 putative terminals from 30 image stacks from 3 Chatires-Cre mice). Terminals are color-coded according to expression of VAChT and VGAT (Black – neither VGAT or VAChT, Magenta – both VGAT and VAChT, Cyan – VAChT only, Yellow – VGAT only). (G) Quantification of the number of terminals of each type in (F).
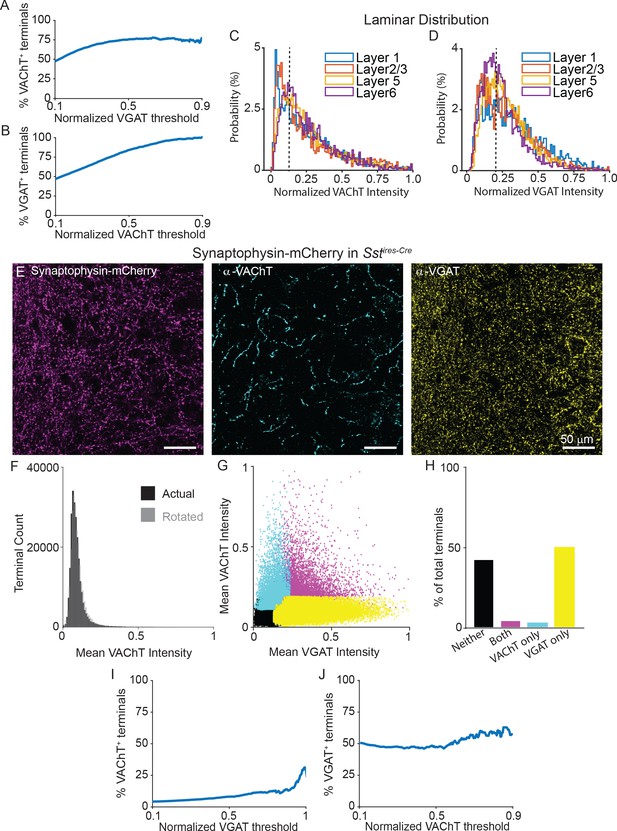
VAChT is present in a subset of terminals across intensity thresholds, and is absent from the terminals of Sst+ interneurons.
(A) The percent of terminals that are positive for VAChT (as determined by a mean intensity threshold greater than the Otsu threshold) across a range of VGAT intensity thresholds. As the threshold for VGAT intensity increases, the percent of VAChT+ terminals plateaus around 70%. (B) The percent of terminals that are positive for VGAT (also determined by a mean intensity threshold greater than the Otsu threshold) across a range of VAChT intensity thresholds. The percent of VGAT+ terminals increases to 100% as the VAChT intensity threshold increases. This indicates that there are two main populations of terminals – those that express both VGAT and VAChT, and those that express only VGAT. (C,D) Distribution of VAChT (C) and VGAT (D) intensity within each terminal across cortical layers in motor cortex. Dashed vertical lines indicate the threshold for classifying terminals as VAChT or VGAT positive. VAChT+ terminals are not uniformly distributed across layers (VAChT+ terminals: 64.25% (1643/2557) in layer 1, 59.35% (15552/2615) in layer 2/3, 70.73% (4137/5849) in layer 5, and 71.21% (3166/4446) in layer 6; p=3.1×10−10, Pearson’s Chi-squared test), and VGAT terminals are not uniformly distributed (VGAT+ terminals: 67.32% (1333/1980) in layer 1, 62.86% (1481/2356) in layer 2/3, 66.72% (3304/4952) in layer 5, 55.80% (2405/4310) in layer 6; p=1.6×10−11, Pearson’s Chi-squared test). (E) Example images of Sst+ pre-synaptic terminals labeled with AAV(8)-DIO-Synaptophysin-mCherry injected into the cortex of Sstires-Cre mice. Left: Synaptophysin-mCherry; Middle: VAChT immunostain; Right: VGAT immunostain. (F) Histogram of mean VAChT fluorescence intensity within Synaptophysin-mCherry+ pre-synaptic terminals of Sst+ interneurons. Black histogram represents the actual VAChT intensities, the grey histogram represents the mean VAChT intensities when the mCherry+ image mask is rotated 90° relative to the VAChT immunostain image. (G) Scatter plot of mean VGAT and VAChT intensity in each putative mCherry+ pre-synaptic terminal (n = 249,899 putative terminals from 14 image stacks from 2 Sstires-Cre mice). Terminals are color-coded according to expression of VAChT and VGAT (Black – neither VGAT or VAChT, Magenta – both VGAT and VAChT, Cyan – VAChT only, Yellow – VGAT only). (H) Quantification of the number of terminals of each type in (E). (I,J) The percent of VAChT+ terminals (G) and VGAT+ terminals (H) across a range of VGAT and VAChT intensity thresholds, respectively. As VGAT intensity threshold increases, there is no relationship with the percent of terminals that are VAChT+ (G). Likewise, as VAChT intensity threshold increases, there is no relationship with the percent of terminals that are VGAT+ (H).
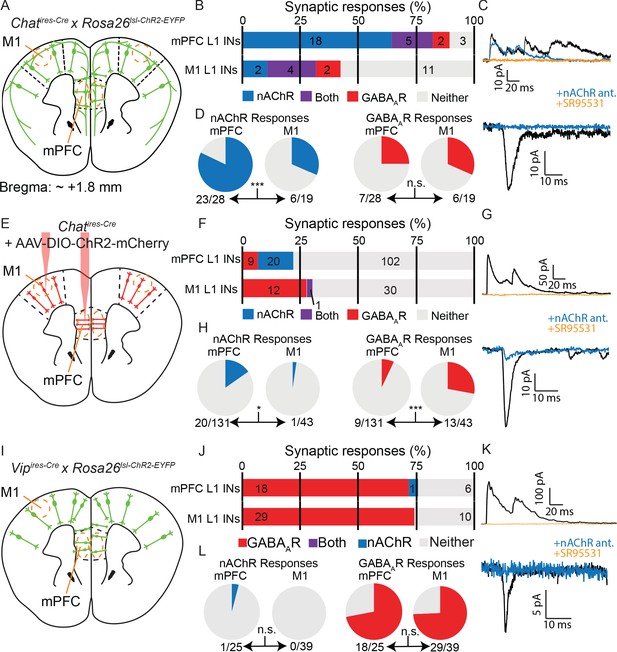
Cholinergic connectivity to Layer 1 interneurons is greater in medial pre-frontal cortex than motor cortex, but not from VIP+ interneurons.
(A) Experimental design: Acute coronal slices taken ~1.8 mm anterior to bregma were obtained from mice where all cholinergic neurons express ChR2 (Chatires-Cre x Rosa26lsl-ChR2) and whole-cell voltage clamp recordings made from layer 1 interneurons in mPFC and M1. (B) Proportion of layer 1 interneurons showing nAChR-mediated and GABAAR-mediated synaptic responses, as determined by clamping the cell to −70 mV and 0 mV, respectively. (n = 47 total neurons from 5 Chatires-Cre x Rosa26lsl-ChR2 mice). (C) Example traces of GABAAR-mediated (top) and nAChR-mediated synaptic responses (bottom), as confirmed by block by gabazine and a cocktail of nAChR antagonists (DHβE, MLA, MEC). (D) Direct comparison of the proportion of cells showing nAChR-mediated responses (left, p=0.0007) or GABAAR-mediated responses (right, p=0.7431). (E–H) Same as (A–D), but only cortical ChAT+ neurons now express ChR2, through injection of Cre-dependent ChR2 virus to both M1 and mPFC (AAV(8)-DIO-ChR2-mCherry). Direct comparison of the proportion of cells with nAChR-mediated responses (bottom) shows a significant decrease in M1 compared to mPFC (left, p=0.0301), while those with GABAAR-mediated responses show a significant increase (right, p=0.0002; n = 174 total neurons from 9 Chatires-Cre mice). (I–L) Same as (A–C) and (E–G), but for acute coronal slices with all VIP+ interneurons expressing ChR2 (Vipires-Cre x Rosa26lsl-CHR2-EYFP). (n = 64 neurons from 7 Vipires-Cre x Rosa26lsl-ChR2-EYFP mice). Example of the sole nAChR-mediated synaptic response obtained from a Layer 1 interneuron following optogenetic stimulation of VIP neurons is shown in (K). Direct comparison of the proportion of cells with nAChR- or GABAAR-mediated responses is not significantly different between mPFC and M1 (p=0.3906 and p=1, respectively). Single asterisk (*) indicates significance at p<0.05, triple asterisk (***) indicates significance at p<0.001 and all p-values calculated by Fisher’s exact test.

Difference in ChAT+ interneuron connectivity are not explained by differences in slicing solution or whole-cell internal recording solution.
(A) Experimental design: Chatires-Cre mice were injected in both mPFC and M1 with AAV(8)-DIO-ChR2-mCherry, and whole cell voltage clamp recordings were obtained from layer 1 interneurons while optogenetically stimulating ChAT+ neurons. (B) Upper panel: summary quantification of cells showing synaptic responses, with GABAAR or nAChR-mediated responses differentiated by their reversal potential (−70 mV and 0 mV, respectively), and pharmacology. Numbers in the bar graph indicate the number of post-synaptic neurons in each category (n = 99 neurons from 7 Chatires-Cre mice for NMDG slicing solution, n = 75 neurons from 3 Chatires-Cre mice for choline cut solution, p=0.1049 for nAChR-mediated responses, p=0.4989 for GABAAR-mediated responses, Fisher’s exact test). Lower panel: comparison of cells showing nAChR-mediated responses (left) or GABAAR-mediated responses (right). (C) As in (B), but comparing cells recorded with CsMeSO4 or Cs-gluconate based internal solutions (n = 96 neurons from 5 Chatires-Cre mice with CeMeSO4, n = 78 neurons from 5 Chatires-Cre mice with Cs-gluconate, p=0.106 for nAChR-mediated responses, p=0.2524 for GABAAR-mediated responses, Fisher’s exact test).
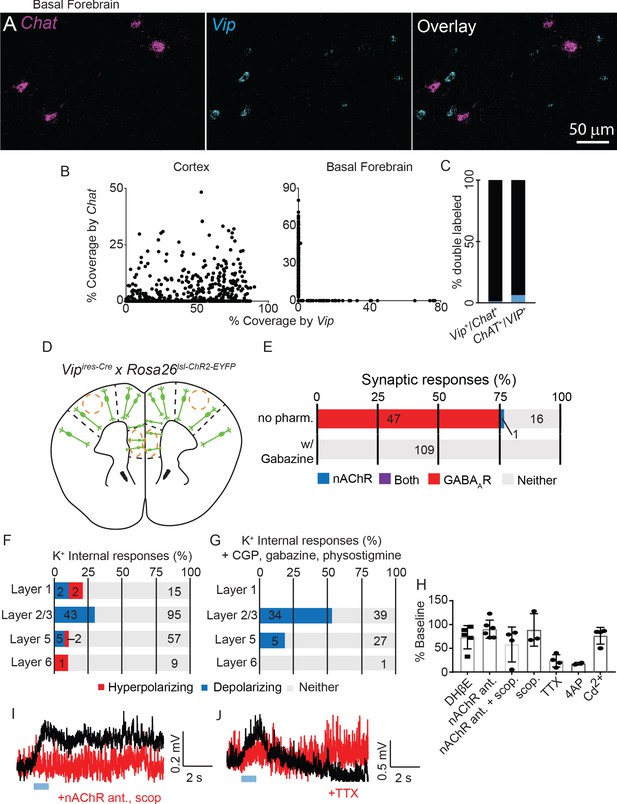
Synaptic connectivity from VIP+ neurons, which excludes basal forebrain cholinergic neurons, results in little to no detectable muscarinic ACh receptor-mediated responses.
(A) Example fluorescent in situ hybridization for Chat and Vip. While Vip-expressing neurons are present in the basal forebrain, they do not colabel with Chat. (B) Comparison of cell coverage by Vip and Chat signal between the cortex and basal forebrain. In the cortex, all neurons with positive cell coverage by Chat also label with Vip. In the basal forebrain, cell coverage by Vip and Chat are mutually exclusive. (C) Summary quantification of the proportion of double-labeled Chat- and Vip-expressing neurons (n = 1 Vip+/Chat+, 41 Vip+ only, 183 Chat+ only cells from 3 wild-type mice). (D) Experimental design: Acute slices from Vipires-Cre x Rosa26lsl-ChR2-EYFP mice were prepared and whole cell recordings with obtained from neurons in different cortical layers. (E) Summary quantification of the proportion of layer 1 interneurons showing synaptic responses following whole-cell voltage clamp and optogenetic stimulation. The responses from both M1 and mPFC, were not significantly different (see Figure 6I–L), and are pooled here. For a significant portion of our recordings, we included we included gabazine in the bath to improve screening for nAChR-mediated currents (n = 174 neurons from 14 Vipires-Cre x Rosa26lsl-ChR2-EYFP mice). (F) Summary quantification of the proportion of cells in whole-cell current clamp showing a synaptic response following trains of optogenetic stimulation of VIP+ interneurons (3 ms pulse, 20 × 20 Hz). Numbers indicate the number of neurons in each category. (n = 231 neurons from 21 Vipires-Cre x Rosa26lsl-ChR2-EYFP mice G) Summary quantification of a subset of cells recorded in (F) were obtained in the presence of gabazine, GABABR-antagonist CGP-35348, and ACh esterase-inhibitor physostigmine in order to improve the chances of observed nAChR- or mAChR-mediated voltage responses. (n = 106 neurons from 10 Vipires-Cre x Rosa26lsl-ChR2-EYFP mice). (H) Summary quantification of the sensitivity of depolarizing responses to different antagonists, including DHβE to block only non-α7 containing nAChRs, a nAChR antagonists cocktail to block all nAChRs (containing MEC, MLA, and DHβE), and scopolamine to block muscarinic ACh receptors. These depolarizing responses were largely insensitive to different ACh receptor antagonists, with one exception shown in (H). We also blocked these responses with voltage-gated sodium channel antagonist TTX, but could not rescue with subsequent addition of 4AP. Finally, these depolarizing responses were insensitive to Cd2+, which inhibits release of synaptic vesicles. Based on these results, we conclude that these depolarizing responses are an artifact of strong synchronous stimulation of VIP+ interneurons resulting in ephaptic potentials. (I) Example depolarizing response that was blocked by ACh receptor antagonists. (J) Additional depolarizing example response that is partially sensitive to block by TTX.
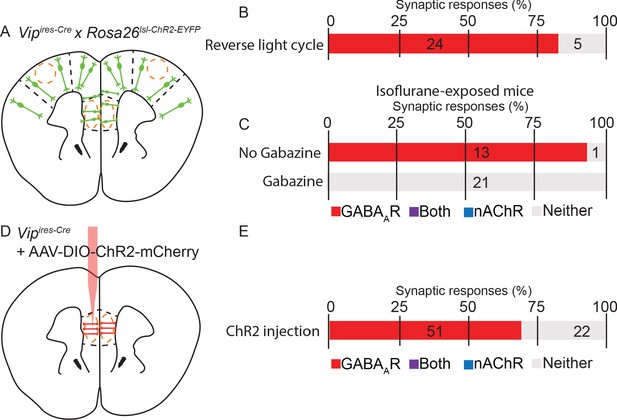
ACh-mediated synaptic connectivity is not effected by circadian cycle or viral injection.
(A) Experimental design: Acute slices from Vipires-Cre x Rosa26lsl-ChR2-EYFP mice were prepared and whole cell voltage clamp recordings obtained from layer 1 interneurons. (B) Summary quantification of cells showing post-synaptic responses in mice raised in a reverse-light cycle (n = 29 neurons from 2 Vipires-Cre x Rosa26lsl-ChR2-EYFP mice). (C) Same as (B), but for mice that experience 3 hr of isoflurane exposure 3 weeks before the experiment. In a portion of the experiments, all recordings were obtained in the presence of gabazine to block GABAAR-mediated synaptic responses and allow for more efficient screening for nAChR-mediated responses. (n = 35 neurons from 2 Vipires-Cre x Rosa26lsl-ChR2-EYFP mice). (D,E) Same as (A–C), but for Vipires-Cre mice injected into the mPFC with AAV(8)-DIO-ChR2-mCherry. (n = 73 neurons from 3 Vipires-Cre mice).
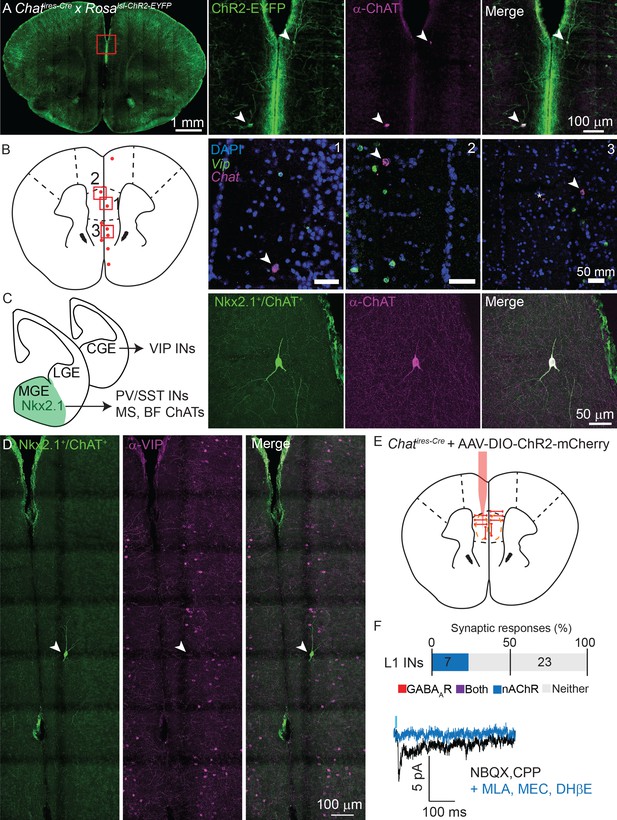
Non-VIP ChAT+ neurons contribute to cholinergic connectivity in the mPFC.
(A) Example images from a Chatires-Cre x Rosa26lsl-CHR2-EYFP mouse. In the mPFC (red inset, left panel), two neurons are shown that are oriented parallel to the cortical surface, as opposed to the typical perpendicular orientation, (middle left panel, arrowheads) which have strong staining against ChAT (middle right panel, arrowheads). (B). Fluorescent in situ hybridization from mPFC reveals a sparse population of cells with strong labeling for Chat, but not for Vip. Approximate location of Chat-expressing, Vip-lacking neurons across 3 different mice are indicated by red dots, and the locations of three example images (right panels) shown by red boxes. Arrowheads indicate Vip-lacking, Chat+ neurons, the asterisk in panel 3 indicates a nearby neuron expressing both Vip and Chat. (C) Cortical VIP+ neurons develop from the caudal ganglionic eminence (CGE), whereas PV+, Sst+, and most cholinergic neurons of the medial septum (MS) and basal forebrain (BF), derive from Nkx2.1-expressing neurons of the medial ganglionic eminence (MGE). Neurons genetically labeled by transient expression of Nkx2.1 and ChAT (Chatires-Cre x Nkx2.1ires-FLP x RC::FLTG, middle left panel) exist in the mPFC that strongly label for ChAT protein (middle right panel) in adult mice. (D) Example Nkx2.1+/ChAT+ neuron (left panel) demonstrating a lack of colabeling with Vip (middle, right panels). (E) Experimental design: AAV(8)-DIO-ChR2-mCherry is injected directly into the mPFC and allowed to express for 3 weeks. Whole-cell voltage clamp recordings were then taken from layer 1 interneurons in the immediate vicinity of putative non-VIP ChAT+ neurons, as indicated by their morphology and large soma compared to VIP+ interneurons. (F) Summary of the proportion of layer 1 interneurons showing synaptic responses following optogenetic stimulation of nearby putative Non-VIP ChAT+ neurons. GABAAR- and nAChR-mediated synaptic responses are differentiated based on reversal potential and sensitivity to selective antagonists. Example nAChR-mediated synaptic response recorded at −70 mV in the presence of glutamatergic antagonists NBQX and CPP, blocked by nAChR antagonists DHβE, MLA, and MEC. No GABAAR-mediated synaptic responses were observed near putative Non-VIP ChAT+ neurons in the absence of other nearby VIP+/ChAT+ neurons. (n = 30 neurons from 6 Chatires-Cre mice).
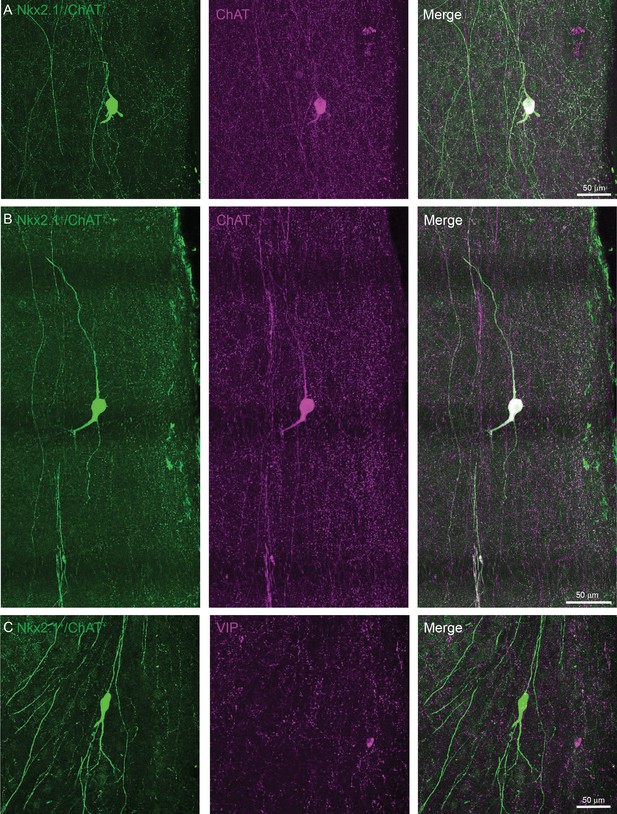
Example images of Non-VIP, ChAT+/Nkx2.1+ neurons in the mPFC.
(A,B) Examples of Nkx2.1+/ChAT+ neurons (Chatires-Cre x Nkx2.1ires-FLP x RC::FLTG, left panels) that colabel with immunostained ChAT (middle panels), demonstrating distinctive morphology of non-VIP, ChAT+ neurons. Superficial surface of the mPFC is aligned to the right in each image. (C) Example of a Nkx2.1+/ChAT+ neurons (left panel) that does not colabel with immunostained VIP (middle panel).
Tables
Reagent type (species) or resource | Designation | Source or reference | Identifiers | Additional information |
---|---|---|---|---|
Strain, strain background (Mus musculus) | Wild-type | Jackson Labs | C57BL6/J | Stock#00664 |
Strain, strain background (Mus musculus) | Chatires-Cre | Jackson Labs | B6;129S6- Chattm1(cre)Lowl/J | Stock # 006410 |
Strain, strain background (Mus musculus) | Vipires-Cre | Jackson Labs | VIPtm1(cre)Zjh/J | Stock # 010908 |
Strain, strain background (Mus musculus) | Sstires-Flp | Jackson Labs | Ssttm3.1(flpo)Zjh/J | Stock # 028579 |
Strain, strain background (Mus musculus) | Rosa26lsl-tdTomato, (Ai14) | Jackson Labs | B6.129Sg-Gt(ROSA)26Sortm14(CAG-tdTomato)Hze/J | Stock # 007908 |
Strain, strain background (Mus musculus) | Rosa26lsl-ChR2-EYFP, (Ai32) | Jackson Labs | B6;129S-Gt(ROSA)26Sortm32(CAG-COP4*H134R/EYFP)Hze/J | Stock # 012569 |
Strain, strain background (Mus musculus) | Sst-GFP (GIN) | Jackson Labs | FVB-Tg(GadGFP)45704Swn/J | Stock # 003718, referred to as "GIN" mice by the Jackson Laboratory, as "Sst-GFP" mice in the text. |
Strain, strain background (Mus musculus) | PV-GFP (G42) | Jackson Labs | CB6-Tg(GAD1-EGFP)G42zjh/J | Stock # 007677, referred to as "GAD67-GFP" or "G42 line" mice by the Jackson laboratory, as "PV-GFP" mice in the text. |
Strain, strain background (Mus musculus) | 5HT3aR-GFP | Gift from B. Rudy lab (NYU) | Tg(Htr3a-EGFP)DH30Gsat | |
Strain, strain background (Mus musculus) | Gad2ires-GFP | Jackson Labs | Gad2tm2(cre)Zjh)/J | Stock # 010802 |
Strain, strain background (Mus musculus) | Slc18a3fl/fl | Gift of V. and M. Prado (UWO) | VAChTflox/flox | Martins-Silva et al., 2011 |
Strain, strain background (Mus musculus) | Slc32a1fl/fl | Jackson Labs | Slc32a1tm1Lowl/J | Stock # 012897 |
Strain, strain background (Mus musculus) | Nkx2.1ires-Flp | Jackson Labs | Nkx2-1tm2.1(flop)Zjh | Stock # 028577 |
Strain, strain background (Mus musculus) | RC::FLTG | Jackson Labs | B6.Cg-Gt(ROSA)26SorTm1.3(CAG-tdTomato,-EGFP)Pjen/J | Stock #026932 |
Strain, strain background (AAV) | AAV(8)-EF1ɑ-hChR2(H134R)-mCherry-WPRE-pA | UNC Vector Core | Titer:~2×1013 gc/ml | |
Strain, strain background (AAV) | AAV(DJ)-EF1ɑ-fDIO-EYFP | BCH Viral Core | Titer:~2×1012 gc/ml | |
Strain, strain background (AAV) | AAV(1)- EF1ɑ-fDIO-ChR2-EYFP | BCH Viral Core | Titer:~5×1013 gc/ml | |
Strain, strain background (AAV) | AAV(8)- EF1ɑ-DIO-FlpO | BCH Viral Core | Titer:~7×1011 gc/ml | |
Strain, strain background (AAV) | AAV(8)- EF1ɑ-DIO-mCherry | UNC Vector Core | Titer:~6×1012 gc/ml | |
Strain, strain background (AAV) | AAV(8)-CMV-DIO-Synaptophysin-EYFP | UNC Vector Core | Titer:~6×1012 gc/ml | |
Strain, strain background (AAV) | AAV(9)-CAG-DIO-Synaptophysin-mCherry | MIT McGovern Viral Core | Titer:~2×1013 gc/ml | |
Antibody | chicken ɑ-GFP | GeneTex | GTC13970 | Dilution: 1:500 |
Antibody | mouse ɑ-Gephyrin | Biosciences Parmingen | 612632 | Dilution: 1:500 |
Antibody | rabbit ɑ-Synapsin-1 | Cell Signaling Technology | 5297S | Dilution: 1:500 |
Antibody | rabbit ɑ-PSD95 | Cell Signaling Technology | 3450 | Dilution: 1:500 |
Antibody | rabbit ɑ-VGAT | Synaptic Systems | 131 011 | Dilution: 1:500 |
Antibody | mouse ɑ-VAChT | Synaptic Systems | 139 103 | Dilution: 1:500 |
Antibody | guinea pig ɑ-VGAT | Millipore | AB5905 | Dilution: 1:500 |
Antibody | goat ɑ-ChAT | Millipore | AB144P | Dilution: 1:500; |
Antibody | Rabbit ɑ-VIP | ImmunoStar | 20077 | Dilution: 1:500 |
Antibody | Rabbit ɑ-Somatostatin, Clone YC7 | Millipore | MAB354 | Dilution: 1:500 |
Antibody | mouse ɑ-Parvalbumin | Millipore | MAB1572 | Dilution: 1:500 |
Sequence-based reagent | Mm-Chat-C2 | ACDBio | 408731-C2 | |
Sequence-based reagent | Mm-Slc32a1 | ACDBio | 319191 | |
Sequence-based reagent | Mm-Gad1-C3 | ACDBio | 400951-C3 | |
Sequence-based reagent | Mm-Gad2-C3 | ACDBio | 439371-C3 | |
Sequence-based reagent | Mm-Slc5a7-C3 | ACDBio | 439941-C3 | |
Sequence-based reagent | Mm-Slc18a3-C3 | ACDBio | 448771-C3 | |
Sequence-based reagent | Mm-Vip | ACDBio | 4159341 | |
Sequence-based reagent | Cre-01-C3 | ACDBio | 474001-C3 | |
Commercial assay or kit | RNAscope Fluorescent Multiplex Detection Reagents | ACDBIO | 320851 |