BLOS1 mediates kinesin switch during endosomal recycling of LDL receptor
Figures
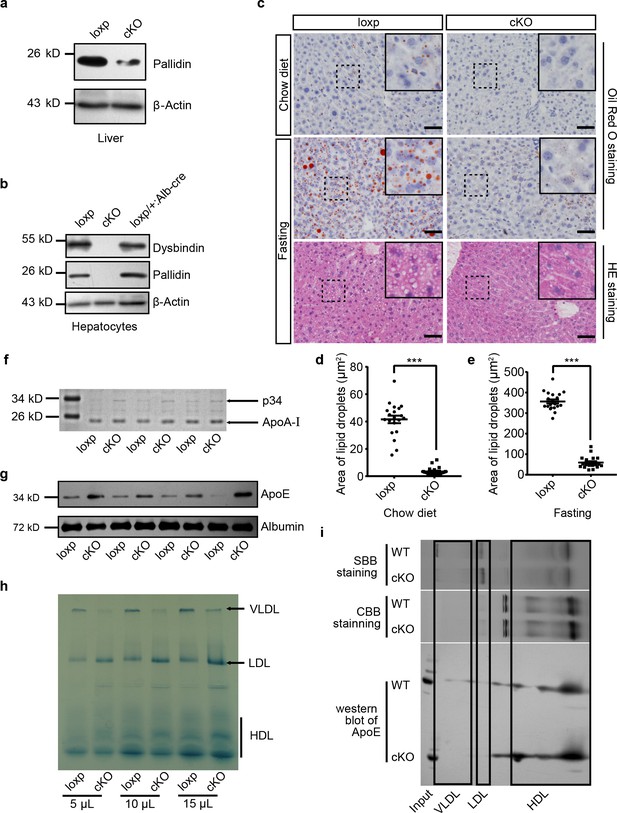
Mice with hepatocyte-specific deletion of BLOS1 showed abnormal lipid metabolism.
(a, b) Immunoblot of the destabilized BLOC-1 subunits (Pallidin and Dysbindin) in lysates of liver (a) and purified primary hepatocytes (b) from loxp and cKO mice. The loxp heterozygous mice in (b) were used as another control. Note that the weak band of Pallidin in (a) indicates the protein from other non-hepatocytes in the liver. (c) Representative images showing the lipid droplets in liver frozen sections of loxp and cKO mice under indicated conditions. For mice after fasting, both Oil Red O staining and HE staining results are displayed. Magnified insets of boxed areas are placed on the top right corners of each picture. Scale bars, 50 μm. (d, e) Average area of lipid droplets in 20 random 50 μm × 50 μm square regions before (d) and after (e) starvation showing reduced lipid droplet content in cKO mice. Quantifications were performed on Oil Red O stained sections. Mean ± SEM. Two-tailed t test, ***p<0.001. (f) Coomassie brilliant blue staining (CBB staining) of plasma proteins in loxp and cKO mice after separation by SDS-PAGE (see the full image in Figure 1—source data 1). (g) Immunoblot of ApoE in plasma of different loxp and cKO mice, albumin is a loading control. (h) Lipoproteins prestained by Sudan Black B in plasma of loxp and cKO mice were separated by 4–15% gradient native PAGE at different loading volume of plasma. (i) Immunoblot of ApoE in lipoproteins separated by native PAGE and a second dimensional SDS-PAGE. Gel slices of prestained lipoproteins and CBB-stained proteins were used to determine the location of different lipoproteins in immunoblots. SBB, Sudan Black B. See also Figure 1—figure supplement 1.
-
Figure 1—source data 1
Full image of CBB staining showing the comparison of control and cKO mice plasma proteins.
Representative figure of CBB staining result of control and cKO mouse plasma proteins separated by SDS-PAGE. Arrow indicates the protein band which was further verified as apoE.
- https://cdn.elifesciences.org/articles/58069/elife-58069-fig1-data1-v2.pptx
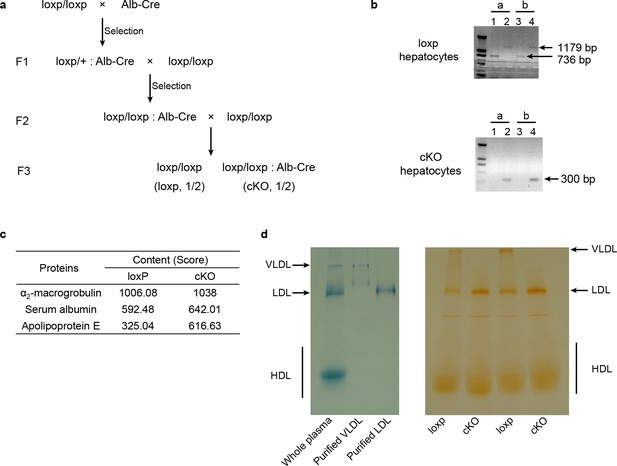
Generation of cKO mice and the abnormal plasma lipoprotein level in cKO mice.
(a) Breeding strategy for the generation and maintaining of loxp and cKO mice littermates which were used in subsequent experiments. (b) Genotyping of hepatocytes of loxp and cKO mice. a and b represent two different mice. Primers used in lane 1 and 3 recognize the loxp sites, and primers used in lane 2 and 4 amplify a larger fragment containing both loxp sites. (c) Mass spectrometry of p34 gel slices showing increased ApoE content (indicated by Score) in cKO mice plasma. (d) Bands of purified lipoproteins after native gel electrophoresis show the position of these lipoproteins in separated plasma samples (left). Oil Red O staining result of native gel separated unstained lipoproteins (right).
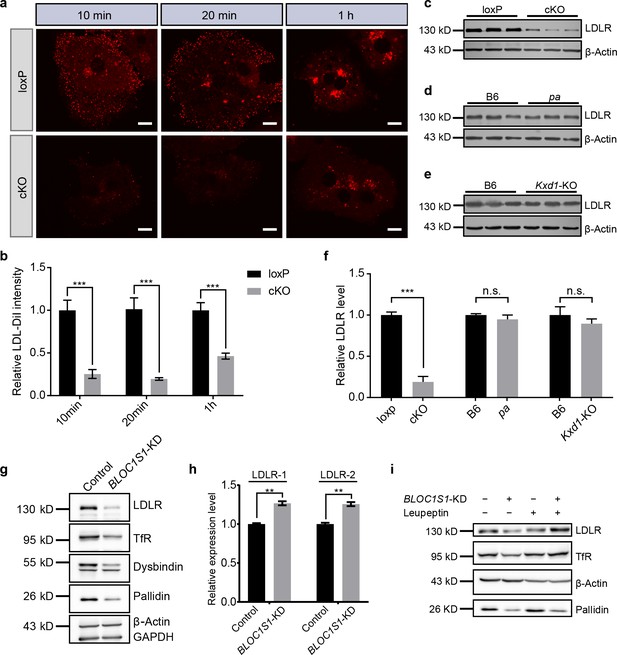
BLOS1 regulates LDLR membrane trafficking and interacts with LDLR.
(a) Endocytosis of DiI-labeled LDL in primary hepatocytes from loxp and cKO mice after indicated time points. Scale bars, 10 μm. (b) Average intensity of LDL-DiI signal after endocytosis in loxp (n = 10 [10min], n = 10 [20 min], n = 11 [2h]) and cKO (n = 8 [10min], n = 9 [20 min], n = 8 [2h]) hepatocytes at indicated time points. Mean ± SEM. Two-tailed t test, ***p<0.001. (c–e) Immunoblot of LDLR in cKO, pa and Kxd1-KO mouse livers showing the decreased LDLR in cKO mice, but not in pa or Kxd1-KO mice. (f) Quantification of relative LDLR protein level (normalized to β-Actin) in pa (n = 3), Kxd1-KO (n = 6) and cKO (n = 5) mice and their control goups. Mean ± SEM. Two-tailed t test; n.s., not significant; ***p<0.001. (g) Detection of LDLR and TfR in BLOC1S1 stable knockdown Hep G2 cells (BLOC1S1-KD cells). Destabilization of Pallidin or Dysbindin is also shown. (h) qRT-PCR of LDLR normalized to GAPDH using two different primer pairs (LDLR-1 and LDLR-2) in control and BLOC1S1-KD cells. Mean ± SEM, three repeats. Two-tailed t test, **p<0.01. (i) Recovery of LDLR level (as well as TfR level) in BLOC1S1-KD cells after the inhibition of lysosomal degradation by treating with leupeptin. See also Figure 2—figure supplement 1.
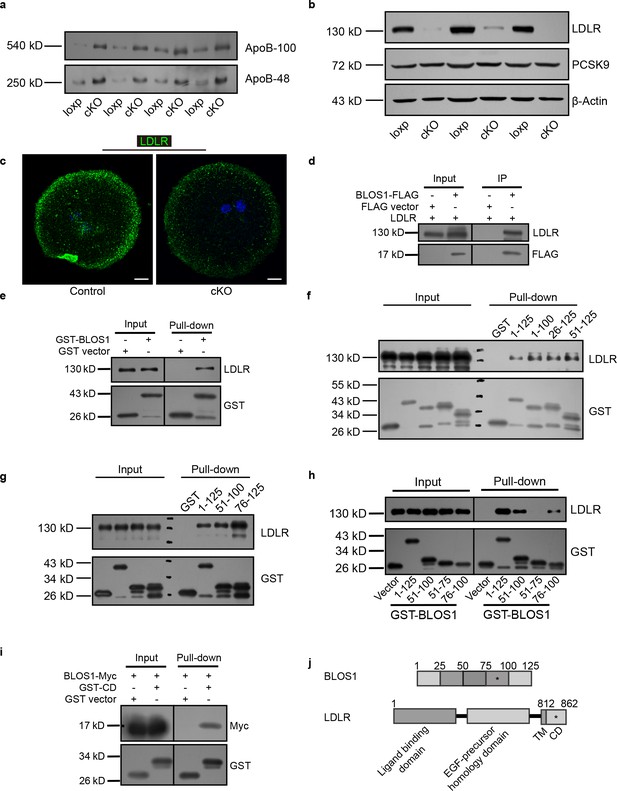
Reduced LDLR in cKO mice and LDLR interacting region mapping on BLOS1.
(a) Immunoblots of ApoB proteins in the plasma of loxp and cKO mice. (b) Immunoblots of LDLR (with another LDLR antibody) and PCSK9 in loxp and cKO mice livers. (c) Representative confocal images of LDLR (green) distribution in control (left) and cKO (right) primary hepatocytes. Scale bars, 10 μm. (d) Co-immunoprecipitation (co-IP) of LDLR with co-overexpressed BLOS1-FLAG in HEK293T whole cell lysate after incubation with anti-FLAG beads, followed by immunoblotting. (e) Pull-down of LDLR in mouse liver lysate by GST-BLOS1 purified with glutathione sepharose beads, followed by immunoblotting. (f, g) Pull-down of LDLR with different BLOS1 truncations fused to GST. BLOS1 was truncated from both sides, and the interacting region was further narrowed with shorter truncations. (h) Pull-down of LDLR by different truncations of BLOS1 fused to GST, showing the interacting region on BLOS1. (i) Pull-down of BLOS1-Myc in HEK293T cell lysate by GST-CD (cytoplasmic domain of LDLR fused to GST). (j) Schematic diagram of mapping of the interacting regions on BLOS1 and LDLR, the star sign (*) represents the mapped region.
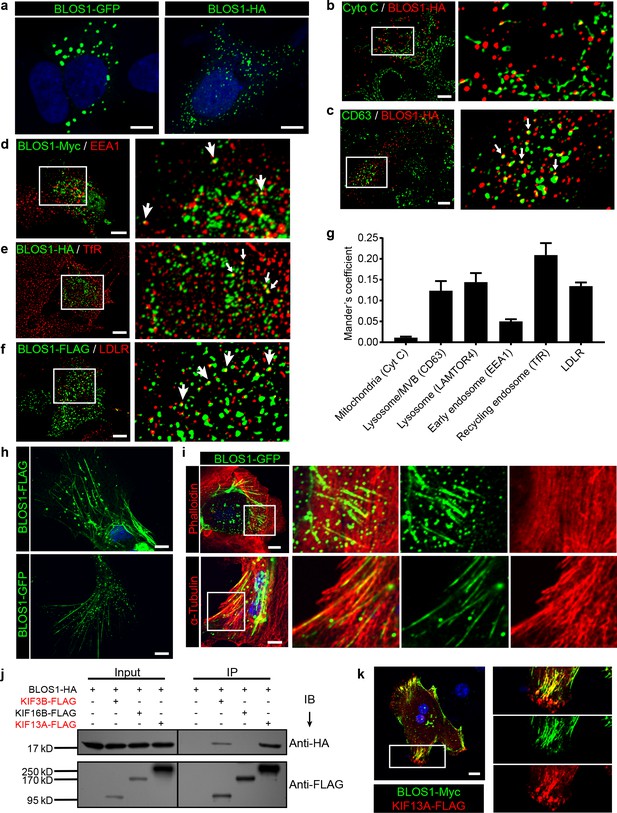
BLOS1 localizes to microtubules and interacts with kinesin motors.
(a) Representative confocal images of puncta patterns of BLOS1-GFP-C2 and immunofluorescence stained BLOS1-HA in Hep G2 cells. (b–f) BLOS1 partially colocalizes with multivesicular body/lysosome marker CD63 (
, white arrows), early endosome marker EEA1 (d), recycling endosome marker TfR (e) and LDLR vesicles (f) in Hep G2 cells, while almost no colocalization was observed between BLOS1-HA and mitochondria marker Cytochrome C (b). Magnified insets of boxed areas are shown on right. (g) Quantification of Mander’s colocalization coefficient showing the percentage of BLOS1 that colocalizes with other proteins or organelle markers. n = 5, 6, 6, 5, 6 and 6 from left to right, respectively. Data are presented as Mean ± SEM. (h) Representative confocal images showing tubular structures of overexpressed BLOS1 with different tags in mouse primary hepatocytes. (i) Tubular BLOS1-GFP expressed in mouse primary hepatocytes distributes on microtubule (indicated by α-Tubulin) (bottom), but not actin filaments (labeled by Phalloidin, top). Merged and single labeling images of magnified insets of boxed areas are shown in bottom panels of each figure. (j) co-IP (immunoprecipitation) of BLOS1-HA with co-overexpressed KIF3B-FLAG or KIF13A-FLAG, but not KIF16B-FLAG, in HEK293T whole cell lysate after incubation with anti-FLAG beads, followed by immunoblotting (IB). (k) BLOS1 tubules colocalize well with KIF13A-FLAG positive microtubules in mouse primary hepatocytes. Merged and single labeling images of magnified insets of boxed areas are shown on right. Scale bars in all pictures, 10 μm.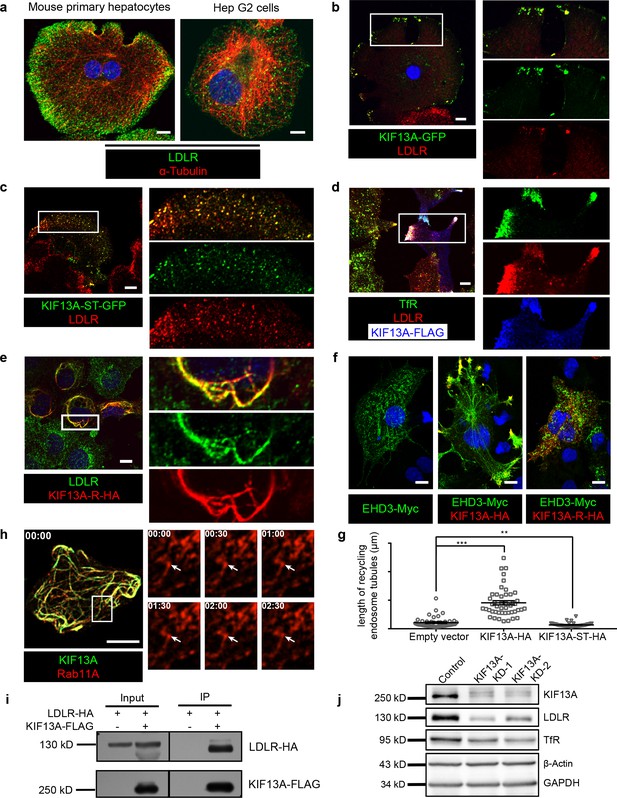
KIF13A transports recycling endosome-resident LDLR.
(a) Representative confocal images showing different distribution patterns of endogenous LDLR (green) in mouse primary hepatocytes and Hep G2 cells. Microtubules were co-labeled with α-tubulin (red) to show the cell morphology. (b) Immunofluorescence staining of LDLR in mouse primary hepatocytes after the expression of KIF13A-GFP. Merged and single labeling images of magnified insets of boxed areas are shown on right. (c) Retention of LDLR in the cytosol of mouse primary hepatocytes by expressing KIF13A-ST truncation. Merged and single labeling images of magnified insets of boxed area are shown on right. (d) Peripherally distributed LDLR driven by KIF13A-FLAG colocalizes with TfR labeled recycling endosomes in Hep G2 cells. Merged and single labeling images of magnified insets of boxed areas are shown on right. (e) Stuck of LDLR on microtubules caused by KIF13A-R point mutant expression in Hep G2 cells. Merged and single labeling images of magnified insets of boxed area are shown on right. (f) Representative immunostaining results showing the elongation of recycling endosome tubules caused by full-length KIF13A and shortening of recycling endosome tubules caused by KIF13A-ST truncation in Hep G2 cells. (g) Average tubular recycling endosomes length measured in empty vector (n = 44), KIF13A-HA (n = 46) and KIF13A-ST-HA (n = 46) expressing cells in (f). Mean ± SEM, two-tailed t test, **p<0.01, ***p<0.001. (h) Confocal live-cell microscopy of RAB11A-Scarlet labeled recycling endosomes in KIF13A-R-GFP expressing Hep G2 cells. Magnified insets (of boxed area) of consecutive time-lapse images (image/30 s) showed that most of the recycling endosomes were retained on KIF13A-R positive microtubules (see Figure 4—video 1). White arrows indicate representative recycling endosomes that were almost static during the imaging period. Time stamps are in the format of minutes: seconds. (i) Co-IP of LDLR-HA with co-overexpressed KIF13A-FLAG in HEK293T whole cell lysate after incubation with anti-FLAG beads, followed by immunoblotting. (j) Immunoblots of LDLR and TfR in KIF13A stable knockdown cells. Scale bars in all pictures, 10 μm. See also Figure 4—figure supplements 1 and 2, Figure 4—videos 1, 2, 3 and 4, Figure 4—source data 1.
-
Figure 4—source data 1
KIF13A transport LDLR to cell periphery in both control and cKO hepatocytes.
(a) Representative confocal image showing the periphery accumulation of LDLR (red) after KIF13A-GFP (green) expression in control primary hepatocytes. (b) Representative confocal image showing the periphery accumulation of LDLR (red) after KIF13A-GFP (green) expression in cKO primary hepatocytes. Magnified insets of boxed areas are shown on bottom. Bar = 10 µm.
- https://cdn.elifesciences.org/articles/58069/elife-58069-fig4-data1-v2.pptx
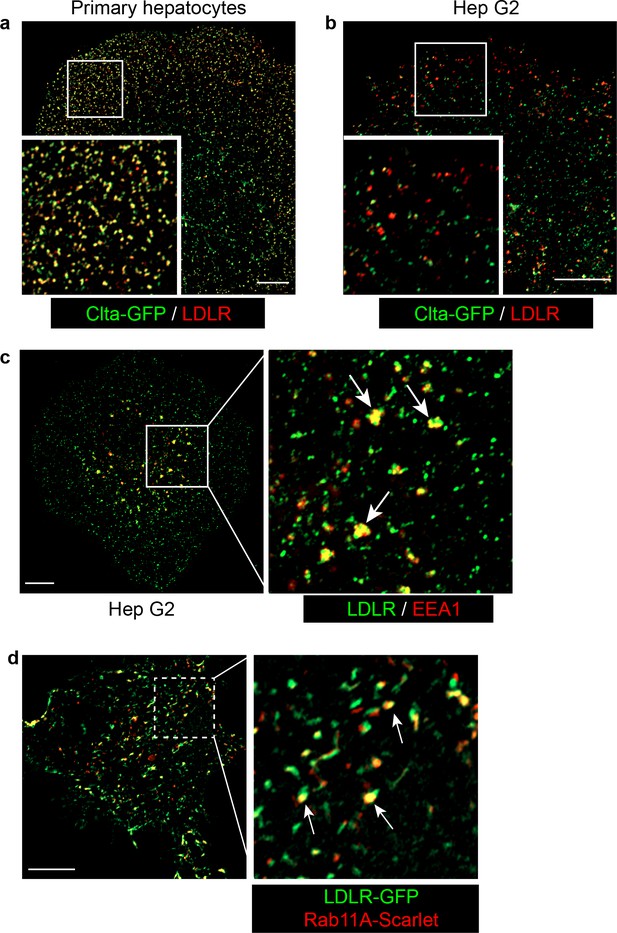
LDLR locates on multiple endocytic compartments in Hep G2 cells.
(a) Representative confocal image of the well colocalization of LDLR and clathrin-coated vesicles (indicated by Clta-GFP) in primary hepatocytes. Magnified insets of boxed areas are shown on the left bottom left corner. (b) Representative confocal image of the partial colocalization of LDLR and clathrin-coated vesicles (indicated by Clta-GFP) in Hep G2 cells. Magnified insets of boxed areas are shown on the left bottom left corner. (c) Representative confocal image showing the partial colocalization (such as puncta indicated by arrows) of LDLR (green) and early endosome marker EEA1 (red) in Hep G2 cells. Magnified inset of boxed area is shown on right. (d) Representative confocal image showing the colocalization of overexpressed Rab11A-Scarlet and LDLR-GFP in Hep G2 cells. Most vesicles labeled by Rab11A are also LDLR-positive (indicated by arrowed). Magnified inset of boxed area is shown on right. Scale bars in all pictures, 10 μm.
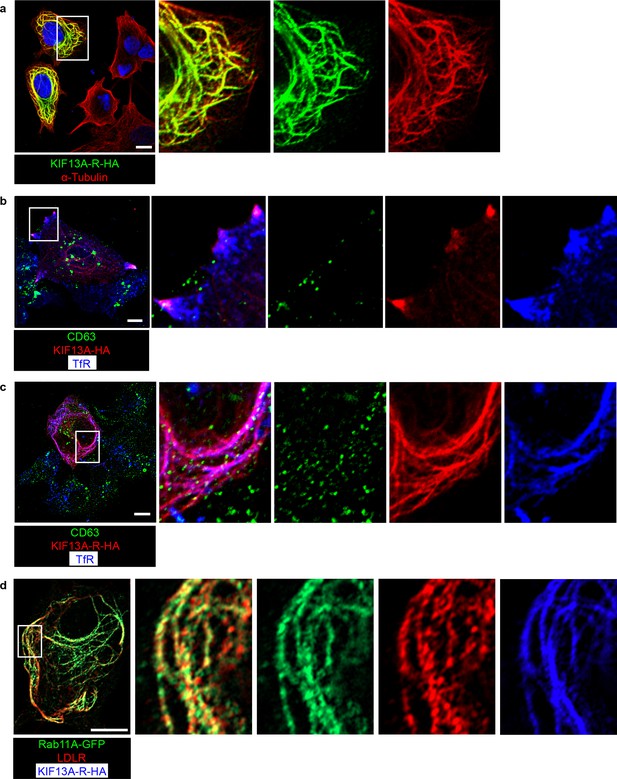
KIF13A and its rigor mutant do not affect lysosome distribution.
(a) Representative confocal image showing KIF13A-R distributes along a proportion of microtubules (indicated by α-Tubulin). (b) Representative confocal image showing that overexpressed full-length KIF13A redistributes recycling endosomes (indicated by TfR) but not lysosomes/MVB (indicated by CD63) to the cell periphery. (c) Representative confocal image showing that KIF13A-R retains recycling endosomes (indicated by TfR) but not lysosomes/MVB (indicated by CD63) on KIF13A-R-positive microtubules. (d) Representative confocal image showing that KIF13A-R retains LDLR on recycling endosomes (indicate by Rab11A). Scale bars in all pictures, 10 μm.
Live-cell imaging of Hep G2 cells transfected with KIF13A-R-Scarlet and RE marker RAB11A-GFP.
Cells were recorded every 1 s for 120 s. Panels on the right show magnified views of the boxed region. Note those almost static REs on KIF13A-R tubules during the imaging period.
Live-cell imaging of Hep G2 cells transfected with KIF13A-R-GFP and then incubated with Dextran-TMR (molecular weight = 10,000) to label lysosomes.
Cells were recorded every 1 s. Boxed areas were enlarged and showed on right.
Live-cell imaging of Hep G2 cells transfected with LDLR-GFP and Rab11a-Scarlet.
Cells were recorded every 0.54 s. Actively moving Rab11a-positive recycling endosomes which were also labeled by LDLR were indicated by arrows.
Live-cell imaging of Hep G2 cells transfected with KIF13A-GFP and Rab11a-Scarlet.
Cells were recorded every 0.2 s. Note the active transport of recycling endosomes in this cell, which was opposite to what we have observed in KIF13A-R expressing cells.
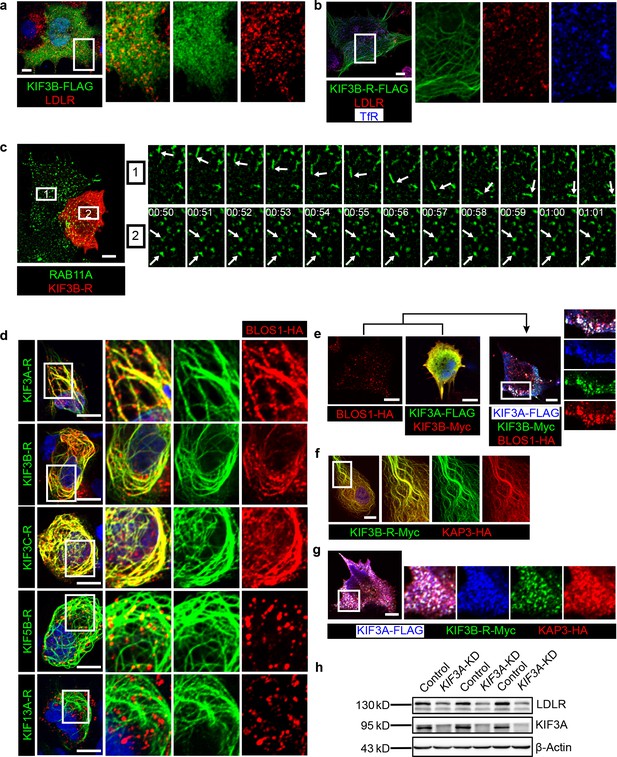
BLOS1 acts as an adaptor protein of KIF3 complex in the regulation of RE anterograde transport.
(a) Immunofluorescence staining of LDLR in KIF3B-FLAG expressing Hep G2 cells. Merged and single labeling images of magnified insets of boxed areas are shown on right. (b) Puncta distribution of LDLR and TfR in KIF3B-R expressing Hep G2 cells. Merged and single labeling images of magnified insets of boxed area are shown on right. (c) Confocal live-cell microscopy of RAB11A-GFP labeled recycling endosomes in KIF3B-R expressing and non-transfected Hep G2 cells. Magnified insets (of box 1 and 2 in the same time points) of consecutive time-lapse images (image/1 s) showed that recycling endosomes in control cells had active motion, while this movement was impaired in KIF3B-R expressing cells (see Figure 5—video 1). White arrows indicate representative recycling endosomes in control and KIF3B-R-expressing cells. Time stamps are in the format of minutes: seconds. (d) Retention of BLOS1 on microtubules caused by co-expressed KIF3A, KIF3B and KIF3C rigor mutants, but not KIF5B or KIF13A rigor mutants in Hep G2 cells. Merged and single labeling images of magnified insets of boxed areas are shown on right. (e) Co-expression of BLOS1 with both KIF3A and KIF3B redistributed KIF3A/B to BLOS1-positive puncta in Hep G2 cells. Merged and single labeling images of magnified insets of boxed area are shown on right. (f, g) Similar retention of KAP3 on microtubules caused by KIF3B-R (f) and redistribution of KIF3A/B resulting from the co-expression of KAP3 (g) in Hep G2 cells. Merged and single labeling images of magnified insets of boxed area are shown on right. (h) Immunoblot of LDLR in KIF3A stable knockdown Hep G2 cells (KIF3A-KD cells). Scale bars in all pictures, 10 μm. See also Figure 5—video 1 and 2, Figure 5—source data 1.
-
Figure 5—source data 1
KIF3A distributes evenly in primary hepatocytes.
Representative confocal images of non-puncta and even distribution pattern of overexpressed KIF3A-Scarlet (red) in mouse primary hepatocytes. Bar = 10 µm.
- https://cdn.elifesciences.org/articles/58069/elife-58069-fig5-data1-v2.pptx
Live-cell imaging of Hep G2 cells transfected with (or without) KIF3B-R-Scarlet and RE marker RAB11A-GFP.
Cells were recorded every 1 s for 120 s. Panels on the right show magnified views of REs in boxed region. Note the active movement of REs in cells without KIF3B-R expression (top right) and impaired RE trafficking in KIF3B-R expressing cells (bottom right).
Live-cell imaging of Hep G2 cells transfected with KIF3B-R-GFP and then incubated with Dextran-TMR (molecular weight = 10,000) to label lysosomes.
Non-transfected cells with Dextran-TMR incubation were used as control. Cells were recorded every 1 s. Boxed areas were enlarged and showed by covering the original pictures.
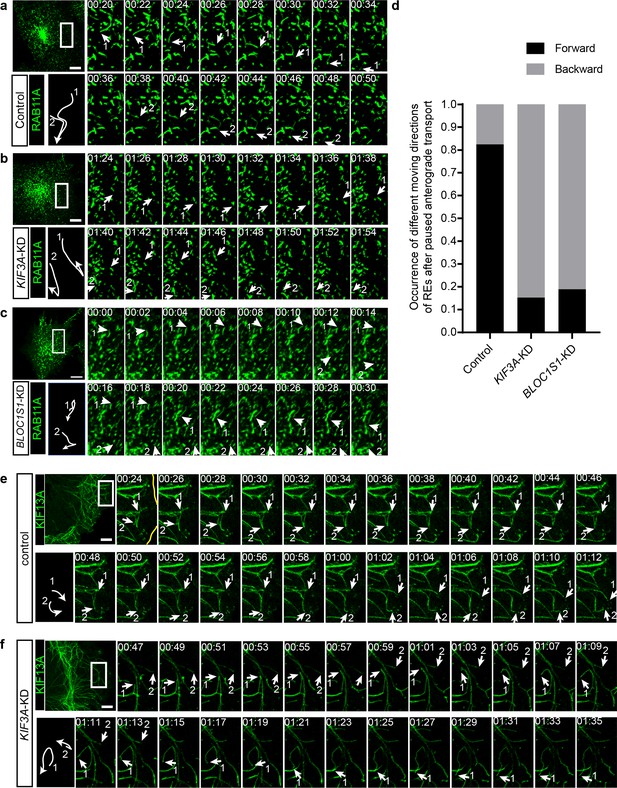
KIF3 is essential for the long-range anterograde transport of REs driven by KIF13A.
(a–c) Confocal live-cell microscopy of RAB11A-GFP labeled recycling endosomes in control (a), KIF3A-KD (b) and BLOC1S1-KD (c) cells (see also Figure 6—videos 1 and 2). Magnified insets (of boxed areas) of consecutive time-lapse images (image/2 s) showed that, compared to control cells, the occurrence of backward movement of recycling endosomes following paused anterograde transport is significantly increased in KIF3A-KD and BLOC1S1-KD cells. White arrows labeled 1 and 2 indicate representative recycling endosomes in these cells. The arrows were drawn perpendicularly to the direction of motion and were reversed when moving in the opposite direction. A trajectory diagram of the representative recycling endosomes is shown on the left bottom. Time stamps are in the format of minutes: seconds. (d) Histogram showing the occurrence of forward and backward motion of recycling endosomes following paused anterograde transport in control (n = 50) and KIF3A-KD (n = 124) cells. (e, f) Confocal live-cell microscopy of KIF13A-GFP tubules in control and KIF3A-KD cells (see Figure 6—video 3). Magnified insets (of boxed areas) of consecutive time-lapse images (image/2 s) showed impaired long-range transport of KIF13A tubules in KIF3A-KD cells. White arrows labeled 1 and 2 indicate tips of representative KIF13A-positive tubules in control (e) and KIF3A-KD (f) cells. The arrows were drawn perpendicularly to the direction of motion and were reversed when moving in the opposite direction. A trajectory diagram of the representative KIF13A tubule tips is shown on the left bottom. Time stamps are in the format of min:s. Scale bars in all pictures, 10 μm. See also Figure 6—figure supplement 1, Figure 6—video 1, 2, 3, 4 and 5.
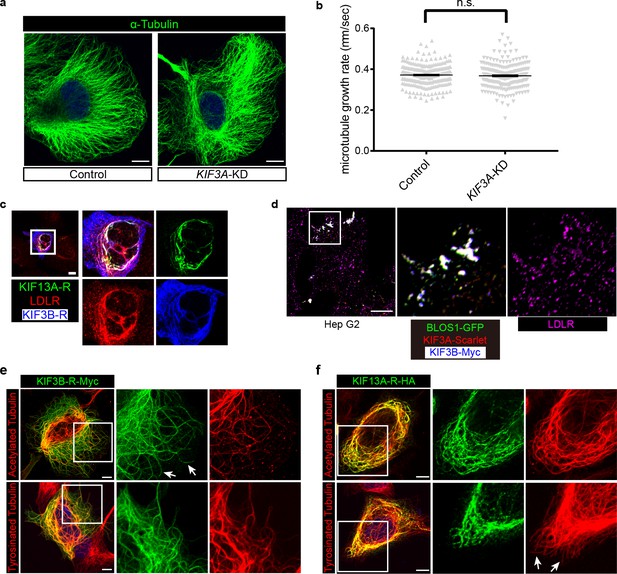
KIF3 and KIF13A bind to the same set of microtubule tracks with acetylation tubulin.
(a) Representative confocal image of microtubules (stained by α-Tubulin) in control and KIF3A-KD cells. (b) Quantification of the microtubule growth rate (indicated by the movement of EB1-GFP labeled positive tips of microtubules) in control (n = 231 tips) and KIF3A-KD (n = 224 tips) cells. Data are presented as Mean ± SEM. Two-tailed t test; n.s., not significant. (c) Immunofluorescence staining of LDLR after co-expression of KIF13A-R-HA and KIF3B-R-Myc. Merged and single labeling images of magnified insets of boxed area are shown on right. (d) When BLOS1 (green), KIF3A (red) and KIF3B (blue) were co-overexpressed in Hep G2 cells, they colocalized well with each other and showed periphery accumulation. But the dispersed distribution of LDLR (Magenta) was not significantly affected by the periphery accumulation of KIF3A/3B-BLOS1. Magnified insets of boxed areas are shown on right. (e) Representative confocal image showing the distribution of KIF3B-R on acetylated and tyrosinated microtubules. Arrows indicate the KIF3B-R-positive microtubules without acetylated Tubulin. (f) Representative confocal images showing the distribution of KIF13A-R on acetylated and tyrosinated microtubules. Arrows indicate the tyrosinated microtubules without KIF13A-R binding. Scale bars in all pictures, 10 μm.
Live-cell imaging of REs in control and KIF3A-KD cells.
Control (left) and KIF3A-KD (right) cells expressing RAB11A-GFP (grayscale) were recorded every 1 s for 120 s. Representative RE vesicles are outlined by circles with distinct color and trajectories are showed in a backward (fast) manner. Arrows on the left indicate pausing points of REs in control cells. Note the reversed moving polarity of circled REs in KIF3A-KD cells after paused anterograde transport.
Live-cell imaging of REs in BLOC1S1-KD cells.
Images were acquired and processed as described in Figure 6—video 1.
Live-cell imaging of KIF13A tubules in control and KIF3A-KD cells.
Control (left) and KIF3A-KD (right) cells expressing KIF13A-GFP were recorded every 1 s for 120 s. Boxed regions in control and KIF3A-KD cells are magnified and showed in the latter part of the movie. Filled arrowheads indicate the tips of KIF13A tubules in control cells that could successfully reach the cell periphery, and the open arrowheads indicate the tip of KIF13A tubules in KIF3A-KD cells that retracted after they encounter other KIF13A tubules.
Live-cell imaging of Hep G2 cells transfected with KIF13A-GFP and KIF3B-R-Scarlet.
Cells were recorded every 0.5 s. Panels on the right show magnified views of the boxed region. KIF13A tubules with repeated forward and backward movement were indicated by arrow.
Live-cell imaging of microtubule dynamics in control (left) and KIF3A-KD (right) cells.
The (+) tips of microtubules were labeled with EB1-GFP, and the movement of these EB1-GFP tips were recorded every 1 s for 120 s.
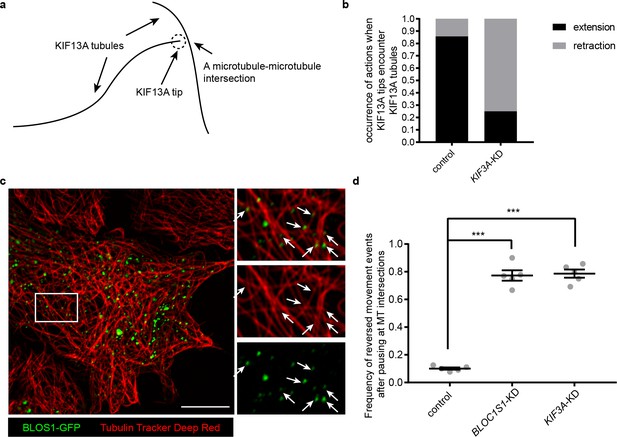
KIF3 and BLOS1 function at specific microtubule intersections.
(a) Schematic of tip of one KIF13A-GFP tubule encounters another KIF13A-GFP tubule. (b) Histogram showing the occurrence of extension and retraction action when tips of KIF13A tubule encounter other KIF13A tubules in control (n = 64) and KIF3A-KD (n = 100) cells. (c) Super-resolution microscopy of the BLOS1-GFP puncta (green) on microtubule tracks (red, labeled by Tubulin Tracker Deep Red) in Hep G2 cells. Merged and single labeling images of magnified insets of boxed area are shown on right. Arrows indicate BLOS1 puncta that locate near microtubule intersections. (d) Frequency of reversed movement after pausing at specific microtubule intersection was increased in both BLOC1S1-KD and KIF3A-KD cells as compared to control cells. Data are presented as Mean ± SEM, n = 5 cells. Two-tailed t test, ***p<0.001. Scale bars in all pictures, 10 μm. See also Figure 7—figure supplement 1, Figure 7—videos 1, 2 and 3.
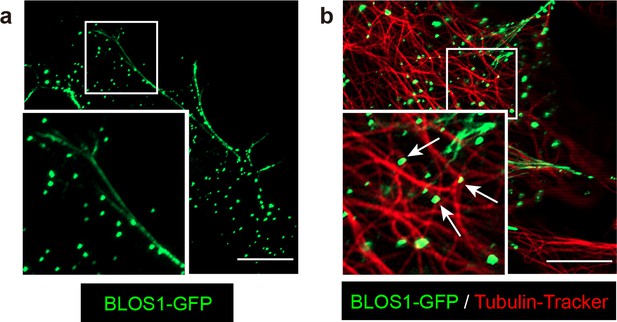
BLOS1 also showed puncta pattern in primary hepatocytes and locates near microtubule intersections.
(a) Overexpressed BLOS1-GFP (green) showed both tubular and puncta distribution in primary hepatocytes. (b) A proportion of BLOS1 puncta (green) locate near the intersections of microtubules (labeled by Tubulin-tracker). Representative BLOS1 puncta were indicated by arrows. Magnified insets of boxed areas are shown on the left bottom left corner of each figure. Scale bars in all pictures, 10 μm.
3D-reconstruction of super-resolution Z-stack images of BLOS1-HA (green) and alpha-tubulin (red).
Z-stacks images were acquired every 170 nm for a total of 50 slices.
Live-cell imaging of recycling endosomes (indicated by RAB11A-GFP) moving on microtubule tracks (stained by Tubulin Tracker Deep Red probe) showing the passage of recycling endosomes after pausing at specific microtubule intersections.
Arrows indicate intersections where recycling endosomes (marked with magenta circles) pause and then go through. Images were acquired with Zeiss LSM 880 using the Airyscan module with line mode, and the time interval between every two frames was 0.32 s.
Live-cell imaging of recycling endosomes (indicated by RAB11A-GFP) moving on microtubule tracks (stained by Tubulin Tracker Deep Red probe) showing the reversed movement of recycling endosomes at specific microtubule intersections.
Arrows indicate intersections where recycling endosomes (marked with magenta circles) pause and then reversed their moving directions. Images were acquired with Zeiss LSM 880 using the Airyscan module with line mode, and the time interval between every two frames was 0.32 s.
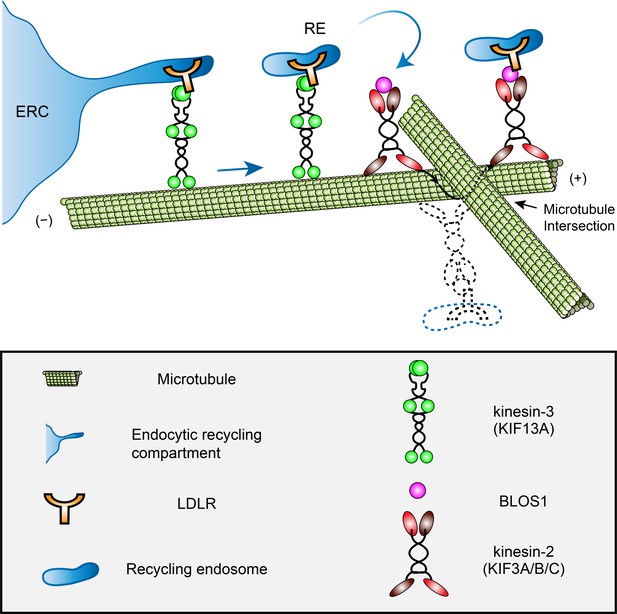
A proposed model of how BLOS1 coordinates kinesin-3 and kinesin-2 at microtubule intersections.
KIF13A drives the generation and extension of tubules at the initial peripheral transport of recycling endosomes (REs) from the endocytic recycling compartment (ERC). When REs (and associated LDLR) arrive at specific microtubule-microtubule intersections, their motion will be paused by steric hindrance. At these sites, through the interaction with kinesin-3 (KIF13A) and LDLR, BLOS1 (and kinesin-2 heterodimers) assists the REs in overcoming obstacles probably by switching between microtubule protofilaments and finally causing the rotation of cargo along the microtubule surface. Dysfunction of KIF3 or BLOS1 results in the inability of REs to pass through these sites and frequently backward movements at specific microtubule intersections. Thus, impaired recycling causes alternative lysosomal degradation of LDLR. See also Figure 8—videos 1 and 2.
Live-cell imaging of BLOS1-GFP puncta showing the frequent z-position change of BLOS1 puncta during recording period.
Two typical BLOS1 puncta are marked by dashed circles, and their intensity profiles are plotted on the right. Arrows indicate the time points at which BLOS1 puncta are in focus and show higher intensity. The time interval between every two frames was 0.58 s.
Live-cell imaging of BLOS1-GFP in Hep G2 cells stained with Tubulin-Tracker (Thermo Fisher).
Two Panels (A and B) on the right show magnified views of two representative regions. The time interval between every two frames was 0.14 s.
Tables
Reagent type (species) or resource | Designation | Source or reference | Identifiers | Additional information |
---|---|---|---|---|
Genetic reagent (M. musculus) | Kxd1-KO | Prepared in our lab (Yang et al., 2012) | ||
Genetic reagent (M. musculus) | pa | The Jackson Laboratory | JAX: 000024; RRID:IMSR_JAX:000024 | |
Genetic reagent (M. musculus) | Alb-Cre | Model animal research center of Nanjing university | RRID:IMSR_JAX:003574 | Derived from The Jackson Laboratory |
Genetic reagent (M. musculus) | loxp | Prepared in our lab (Zhang et al., 2014) | ||
Genetic reagent (M. musculus) | Bloc1s1-cKO | This paper | ||
Strain, strain background (Escherichia coli) | BL21(DE3) | Vazyme | C504 | Chemical competent cells |
Strain, strain background (Escherichia coli) | DH5α | Vazyme | C502 | Chemical competent cells |
Cell line (Homo-sapiens) | Hep G2 | Cell bank of Chinese Academy of Sciences (Shanghai, China) | Cat#TCHu72; RRID:CVCL_0027 | Has been authenticate by STR profiling and tested negative for mycoplasma in cell bank |
Cell line (Homo-sapiens) | HEK293T | Cell bank of Chinese Academy of Sciences (Shanghai, China) | Cat#GNHu17; RRID:CVCL_0063 | Has been authenticate by STR profiling and tested negative for mycoplasma in cell bank |
Biological sample (M. musculus) | Primary mouse hepatocytes | This paper | Freshly isolated from mouse liver | |
Antibody | Mouse monoclonal anti-alpha Tubulin (clone DM1A) | Abcam | Cat#ab7291;RRID:AB_2241126 | IF (1:500) |
Antibody | Rabbit polyclonal anti-alpha Tubulin | Abcam | Cat#ab18251; RRID:AB_2210057 | IF (1:1000) |
Antibody | Rabbit polyclonal anti-Transferrin Receptor | Abcam | Cat#ab84036; RRID:AB_10673794 | IF (1:200), WB (1:2000) |
Antibody | Rabbit polyclonal anti-LDL Receptor | Abcam | Cat#ab30532; RRID:AB_881272 | WB (1:2000) |
Antibody | Rabbit monoclonal anti-LDL Receptor (clone EP1553Y) | Abcam | Cat#ab52818; RRID:AB_881213 | WB (1:5000) |
Antibody | Rabbit polyclonal anti-PCSK9 | Abcam | Cat#ab31762; RRID:AB_777140 | WB (1:1000) |
Antibody | Goat polyclonal anti-HA tag antibody | Abcam | Cat#ab9134; RRID:AB_307035 | IF (1:1000), WB (1:5000) |
Antibody | Rat monoclonal anti-mouse IgG for IP (HRP) | Abcam | Cat#ab131368; N/A | WB (1:5000) |
Antibody | Donkey anti-rabbit Alexa Fluor 405 | Abcam | Cat#ab175649; AB_2715515 | IF (1:1000) |
Antibody | Mouse monoclonal anti-EEA1 (clone 14) | BD | Cat#610457; RRID:AB_397830 | IF (1:500) |
Antibody | Mouse monoclonal anti-Cytochrome C (clone 6H2.B4) | BD | Cat#556432; RRID:AB_396416 | IF (1:250) |
Antibody | Mouse monoclonal anti-CD63 (clone H5C6) | BD | Cat#556019; RRID:AB_396297 | IF (1:200) |
Antibody | Goat polyclonal anti-mouse LDL Receptor | R and D | Cat#AF2255; RRID:AB_355203 | IF (1:100) |
Antibody | Goat polyclonal anti-human LDL Receptor | R and D | Cat#AF2148; RRID:AB_2135126 | IF (1:100) |
Antibody | Mouse monoclonal anti-beta Actin (clone AC-15) | Sigma-Aldrich | Cat#A5441; RRID:AB_476744 | WB (1:50000) |
Antibody | Mouse monoclonal ant-Acetylated Tubulin antibody (clone 6-11B-1) | Sigma-Aldrich | Cat#T7451; RRID:AB_609894 | IF (1:200) |
antibody | Mouse monoclonal anti-FLAG tag antibody (clone M2) | Sigma-Aldrich | Cat#F3165; RRID:AB_259529 | IF (1:1000), WB (1:5000) |
Antibody | Rabbit polyclonal anti-FLAG tag antibody | Sigma-Aldrich | Cat#F7425; RRID:AB_439687 | IF (1:1000) |
Antibody | Rat monoclonal anti-Tyrosinated Tubulin antibody (clone YL1/2) | Millipore | Cat#MAB1864; RRID:AB_2210391 | IF (1:200) |
Antibody | Rabbit monoclonal anti-KIF3A antibody (clone D7G3) | Cell Signaling Technology | Cat#8507; RRID:AB_11141049 | WB (1:1000) |
Antibody | Rabbit polyclonal anti-KIF13A antibody | Bethyl Laboratories | Cat#A301-077A; RRID:AB_873053 | WB (1:1000) |
Antibody | Rabbit polyclonal anti-Myc tag antibody | MBL | Cat#562; RRID:AB_591105 | IF (1:500) |
Antibody | Mouse monoclonal anit-Myc tag antibody (clone Myc3) | MBL | Cat#M192-3S; RRID:AB_11161202 | IF (1:500), WB (1:2000) |
Antibody | Mouse monoclonal anti-HA tag antibody (clone F-7) | Santa Cruz | Cat#sc-7392; RRID:AB_627809 | IF (1:100) |
Antibody | Mouse monoclonal anti-GST antibody (clone B-14) | Santa Cruz | Cat#sc-138; RRID:AB_627677 | WB (1:5000) |
Antibody | Rabbit polyclonal anti-Pallidin antibody | Proteintech | Cat#10891–2-AP; RRID:AB_2164174 | WB (1:2000) |
Antibody | Rabbit polyclonal anti-Dysbindin antibody | Prepared in our lab Wang et al., 2014 | N/A | WB (1:20000) |
Antibody | Donkey anti-mouse Alexa Fluor 488 | ThermoFisher | Cat#A-21202; RRID:AB_141607 | IF (1:1000) |
Antibody | Donkey anti-mouse Alexa Fluor 594 | ThermoFisher | Cat#A-21203; RRID:AB_2535789 | IF (1:1000) |
Antibody | Donkey anti-Rabbit Alexa Fluor 488 | ThermoFisher | Cat#A-21206; RRID:AB_2535792 | IF (1:1000) |
Antibody | Donkey anti-Rabbit Alexa Fluor 594 | ThermoFisher | Cat#A-21207; RRID:AB_141637 | IF (1:1000) |
Antibody | Donkey anti-Goat Alexa Fluor 488 | ThermoFisher | Cat#A-11055; RRID:AB_2534102 | IF (1:1000) |
Antibody | Donkey anti-Goat Alexa Fluor 594 | ThermoFisher | Cat#A-11058; RRID:AB_2534105 | IF (1:1000) |
Recombinant DNA reagent | BLOS1-GFP-C2 (plasmid) | This paper | ||
Recombinant DNA reagent | BLOS1-GFP-N2 | This paper | ||
Recombinant DNA reagent | BLOS1-FLAG | This paper | ||
Recombinant DNA reagent | BLOS1-Myc | This paper | ||
Recombinant DNA reagent | BLOS1-HA | This paper | ||
Recombinant DNA reagent | GST-BLOS1 | This paper | ||
Recombinant DNA reagent | RAB11A-GFP-C2 | This paper | ||
Recombinant DNA reagent | RAB11A-Scarlet-C2 | This paper | ||
Recombinant DNA reagent | KIF13A-FLAG | This paper | ||
Recombinant DNA reagent | KIF13A-GFP-N2 | This paper | ||
Recombinant DNA reagent | KIF13A-ST-GFP-N2 | This paper | ||
Recombinant DNA reagent | KIF13A-HA | This paper | ||
Recombinant DNA reagent | KIF13A-R-HA | This paper | ||
Recombinant DNA reagent | KIF13A-R-Scarlet-N2 | This paper | ||
Recombinant DNA reagent | KIF3B-Myc | This paper | ||
Recombinant DNA reagent | KIF3B-R-Myc | This paper | ||
Recombinant DNA reagent | KIF3B-R-Scarlet-N2 | This paper | ||
Recombinant DNA reagent | KIF3A-FLAG | This paper | ||
Recombinant DNA reagent | KIF3A-R-FLAG | This paper | ||
Recombinant DNA reagent | KIF3C-HA | This paper | ||
Recombinant DNA reagent | KIF3C-R-HA | This paper | ||
Recombinant DNA reagent | KAP3-HA | This paper | ||
Recombinant DNA reagent | KIF5B-R-Myc | This paper | ||
Recombinant DNA reagent | KIF16B-FLAG | This paper | ||
Recombinant DNA reagent | KIF3A-FLAG-KIF3B-Myc-BLOS1-HA | This paper | ||
Sequence-based reagent | LDLR_1F | This paper | RT-PCR primers | GTCTTGGCACTGGAACTCGT |
Sequence-based reagent | LDLR_1R | This paper | RT-PCR primers | CTGGAAATTGCGCTGGAC |
Sequence-based reagent | LDLR-2F | This paper | RT-PCR primers | ACGGCGTCTCTTCCTATGACA |
Sequence-based reagent | LDLR-2R | This paper | RT-PCR primers | CCCTTGGTATCCGCAACAGA |
Sequence-based reagent | GAPDH-F | This paper | RT-PCR primers | GGAGCGAGATCCCTCCAAAAT |
Sequence-based reagent | GAPDH-R | This paper | RT-PCR primers | GGCTGTTGTCATACTTCTCATGG |
Sequence-based reagent | KIF13A-R-F | This paper | Site-directed mutagenesis primers | GAGCCTGGTAGACCTGGCGGCGAGCGAGAGAGTGTCGAAGAC |
Sequence-based reagent | KIF13A-R-R | This paper | Site-directed mutagenesis primers | GTCTTCGACACTCTCTCGCTCGCCGCCAGGTCTACCAGGCTC |
Sequence-based reagent | KIF3B-R-F | This paper | Site-directed mutagenesis primers | CTGAATCTTGTAGATCTTGCTGCCAGTGAGCGGCAAGCCAAG |
Sequence-based reagent | KIF3B-R-R | This paper | Site-directed mutagenesis primers | CTTGGCTTGCCGCTCACTGGCAGCAAGATCTACAAGATTCAG |
Sequence-based reagent | KIF3C-R-F | This paper | Site-directed mutagenesis primers | GTAGACCTGGCCGCCAGTGAGAGACAG |
Sequence-based reagent | KIF3C-R-R | This paper | Site-directed mutagenesis primers | CTGTCTCTCACTGGCGGCCAGGTCTAC |
Sequence-based reagent | KIF5B-R-F | This paper | Site-directed mutagenesis primers | CTGGTTGATTTAGCTGCTAGTGAAAAGGTTAG |
Sequence-based reagent | KIF5B-R-R | This paper | Site-directed mutagenesis primers | CTAACCTTTTCACTAGCAGCTAAATCAACCAG |
Sequence-based reagent | MfeI-F | This paper | Site-directed mutagenesis primers for pSilencer 5.1-H1 Retro vector | ATGGAGGACCCCAATGCCAAGG |
Sequence-based reagent | MfeI-R | This paper | Site-directed mutagenesis primers for pSilencer 5.1-H1 Retro vector | CCGAGTGGCTGTGGCTTCC |
Sequence-based reagent | BLOS1-1F | This paper | shRNA template primers | gatccgTCGGAATGGTGGAGAACTTgagaAAGTTCTCCACCATTCCGAttttttggaaa |
Sequence-based reagent | BLOS1-1R | This paper | shRNA template primers | agcttttccaaaaaaTCGGAATGGTGGAGAACTTtctcAAGTTCTCCACCATTCCGAcg |
Sequence-based reagent | BLOS1-2F | This paper | shRNA template primers | gatccGCACTGGAATATGTCTACAgagaTGTAGACATATTCCAGTGCttttttggaaa |
Sequence-based reagent | BLOS1-2R | This paper | shRNA template primers | agcttttccaaaaaaGCACTGGAATATGTCTACAtctcTGTAGACATATTCCAGTGCg |
Sequence-based reagent | BLOS1-3F | This paper | shRNA template primers | gatccgCAGAAGCTTTGGTGGATCAgagaTGATCCACCAAAGCTTCTGttttttggaaa |
Sequence-based reagent | BLOS1-3R | This paper | shRNA template primers | agcttttccaaaaaaCAGAAGCTTTGGTGGATCAtctcTGATCCACCAAAGCTTCTGcg |
Sequence-based reagent | KIF13A-1F | This paper | shRNA template primers | cggGGAAACCTCCCAAGGTATTTGgagaCAAATACCTTGGGAGGTTTCCtttttga |
Sequence-based reagent | KIF13A-1R | This paper | shRNA template primers | agcttCAAAAAGGAAACCTCCCAAGGTATTTGtctcCAAATACCTTGGGAGGTTTCC |
Sequence-based reagent | KIF13A-2F | This paper | shRNA template primers | ccggTTAACGAACTTCTGGTTTATTgagaAATAAACCAGAAGTTCGTTAAtttttga |
Sequence-based reagent | KIF13A-2R | This paper | shRNA template primers | agcttCAAAAATTAACGAACTTCTGGTTTATTtctcAATAAACCAGAAGTTCGTTAA |
Sequence-based reagent | KIF3A-1F | This paper | shRNA template primers | ccggCGTCAGTCTTTGATGAAACTAgagaTAGTTTCATCAAAGACTGACGtttttga |
Sequence-based reagent | KIF3A-1R | This paper | shRNA template primers | agcttCAAAAACGTCAGTCTTTGATGAAACTAtctcTAGTTTCATCAAAGACTGACG |
Sequence-based reagent | KIF3A-2F | This paper | shRNA template primers | ccggGCCTGTTTGAACACATTCTAAgagaTTAGAATGTGTTCAAACAGGCtttttga |
Sequence-based reagent | KIF3A-2R | This paper | shRNA template primers | agcttCAAAAAGCCTGTTTGAACACATTCTAAtctcTTAGAATGTGTTCAAACAGGC |
Sequence-based reagent | KIF3A-3F | This paper | shRNA template primers | ccggCGGGATTATCAGGAAATGATTgagaAATCATTTCCTGATAATCCCGtttttga |
Sequence-based reagent | KIF3A-3R | This paper | shRNA template primers | agcttCAAAAACGGGATTATCAGGAAATGATTtctcAATCATTTCCTGATAATCCCG |
Peptide, recombinant protein | Streptavidin | Thermo Fisher | Cat. #: 434302 | |
Commercial assay or kit | iScript cDNA Synthesis Kit | BIO-RAD | 1708891 | |
Commercial assay or kit | RNeasy Mini Kit | QIAGEN | 74104 | |
Commercial assay or kit | ClonExpress II One Step Cloning Kit | Vazyme | C112 | |
Chemical compound, drug | Puromycin | InvivoGen | ant-pr-1 | |
Chemical compound, drug | Leupeptin | Sigma-Aldrich | L5793 | |
Chemical compound, drug | Oil Red O | Sigma-Aldrich | O9755 | |
Chemical compound, drug | Sudan Black B | Sigma-Aldrich | 199664 | |
Chemical compound, drug | 1,1’-Dioctadecyl-3,3,3’,3’-tetramethylindocarbocyanine perchlorate (DiI) | Sigma-Aldrich | 42364 | |
Software, algorithm | Fiji | http://fiji.sc/; Schindelin et al., 2012 | RRID:SCR_002285 | Version 2.0.0-rc-69/1.52 n |
Other | Minimal Essential Medium (MEM) | GE Healthcare | SH30024.01 | |
Other | Glutathione Sepharose 4B resin | GE Healthcare | 17075601 | |
Other | Collagen, Type I | Sigma-Aldrich | C3867 | |
Other | Collagenase, TypeIV | Sigma-Aldrich | C5138 | |
Other | Anti-FLAG M2 affinity gel | Sigma-Aldrich | A2220 | |
Other | Phalloidin Alexa Fluor 594 | ThermoFisher | A12381 | |
Other | Prolong Gold Antifade Mountant | ThermoFisher | P36935 | |
Other | Lipofectamine 3000 | ThermoFisher | L3000015 | |
Other | Sodium pyruvate | ThermoFisher | 11360070 | |
Other | MEM Non-Essential Amino Acids | ThermoFisher | 11140050 | |
Other | Tubulin Tracker Deep Red | ThermoFisher | T34076 | |
Other | jetPEI-Hepatocyte | Polyplus | 102–05N |
-
Note: The listed references in this table can be referred to the reference list in main text.