Functional specification of CCK+ interneurons by alternative isoforms of Kv4.3 auxiliary subunits
Figures
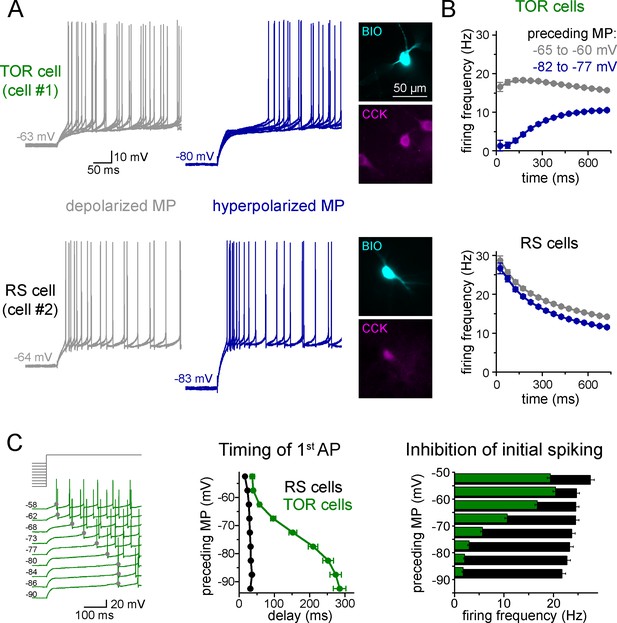
Two distinct firing patterns within CA3 CCK+ cells.
(A) Firing properties of two representative CCK+INs in the CA3 hippocampal region. Firing was elicited with square pulse current injection of identical amplitude, but from depolarized (grey traces), or hyperpolarized MPs (blue traces). Several trials are superimposed to show the stability of the timing of the first action potential. Insets show the immunolabelling of the biocytin filled (BIO) recorded cells for CCK. (B) Average time course of AP occurrence in TOR and RS cells from two MP ranges (n = 120 and 113 representative cells, respectively). (C) Timing of the first AP and probability of APs during the first 150 ms of the square pulse stimulus shows steep MP-dependence in TOR cells, whereas the initial spikes are stable in the RS cells. The amplitude of stimulating current steps was standardized for each cell and only the preceding holding current (3 s) was varied in individual trials. Traces show a representative recording from a TOR cell. The average data derived from 85 TOR and 81 RS cells.
-
Figure 1—source data 1
Electrophysiological parameters of TOR and RS CCK+INs.
Note that only those cells were included in this table from which all parameters could be retrieved. Thus, the number of analyzed cells may differ from specific analysis of the main text.
- https://cdn.elifesciences.org/articles/58515/elife-58515-fig1-data1-v1.docx
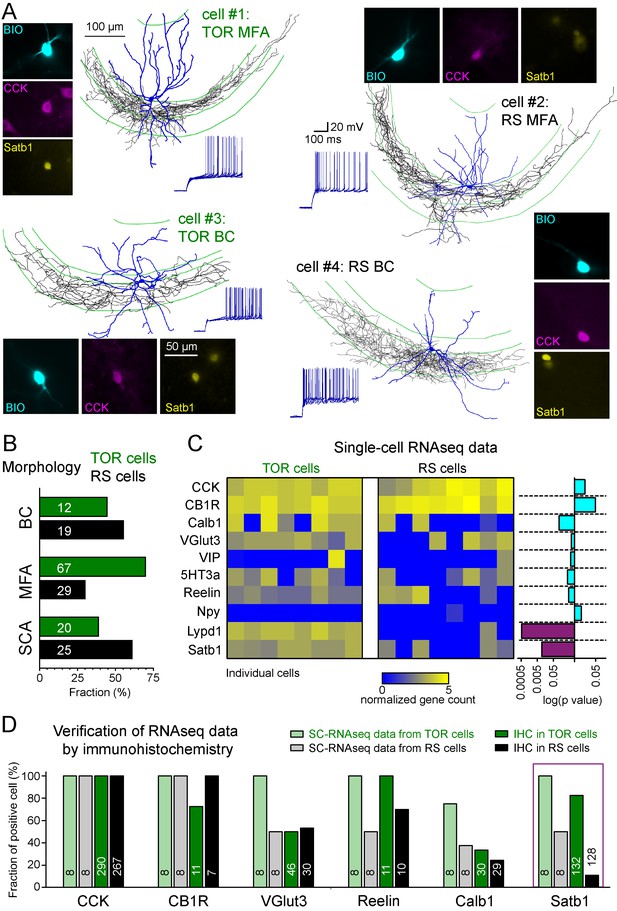
Different excitability does not correlate with previously known diversity of CCK+ cells.
(A) Axonal (black) and dendritic (blue) reconstructions of four CCK+INs in the CA3 region representing two major morphological types, the mossy-fiber associated cells and basket cells. The firing of cell#1 and #2 are shown in detail in Figure 1A. The firing from hyperpolarized MP is shown for each cell. Immuno-labelling for CCK and the nuclear protein Satb1 are shown next to each recorded cell. (B) Prevalence of TOR and RS cells within three major morphological types of CA3 CCK+. Numbers of identified cells are indicated on each bar (BC: CCK+ basket cells, MFA: mossy-fiber associated cells, SCA: Schaffer-collateral associated cells). (C) Single cell RNAseq characterization of recorded TOR (left, n = 8) and RS (right, n = 8) cells for known GABAergic cell markers. Each column corresponds to single identified CCK+INs. The bars on the right shows the logarithm of p values of the statistical comparison of TOR and RS cells for each genes. Significantly different mRNA content between TOR and RS cells was found for Satb1 and Lypd1 (p=0.0095 and p=0.0006, Mann-Whitney Test). (D) Comparison of immunohistochemical and single cell RNAseq data. Bar plots show the fraction of the recorded cells with detectable RNA content (threshold: 0.2) for the selected markers and immunopositivity for the same proteins (for examples of the immunolabelling see Figure 2—figure supplement 3). The number of tested cells are shown on each bar.
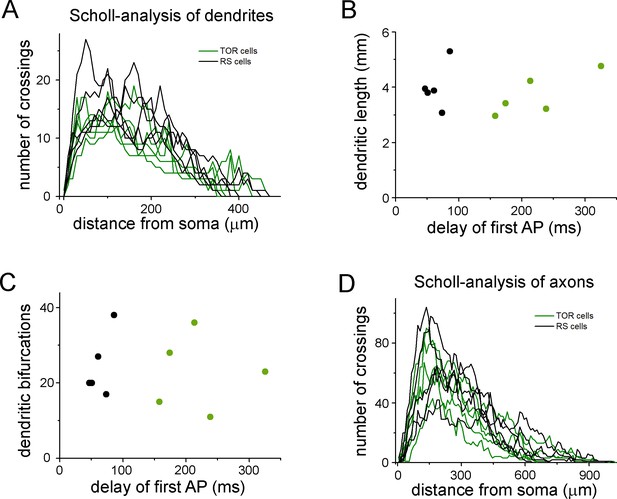
Quantitative comparison of the dendritic and axonal morphologies of TOR and RS cells.
(A) Number of dendrites branches at various radii from the soma. This Scholl-type analysis was performed on 10 three-dimensionally reconstructed cells (5 TOR and 5 RS cells, each line represents individual cells). (B) The total length of the dendrites of the ten reconstructed cells did not show correlation with the delay of the first APs, which was distinct between TOR and RS cells. (C) The number of branching points did not correlate with the delay of the first APs. (D) Scholl-type analysis of the axonal arbor of the ten cells.
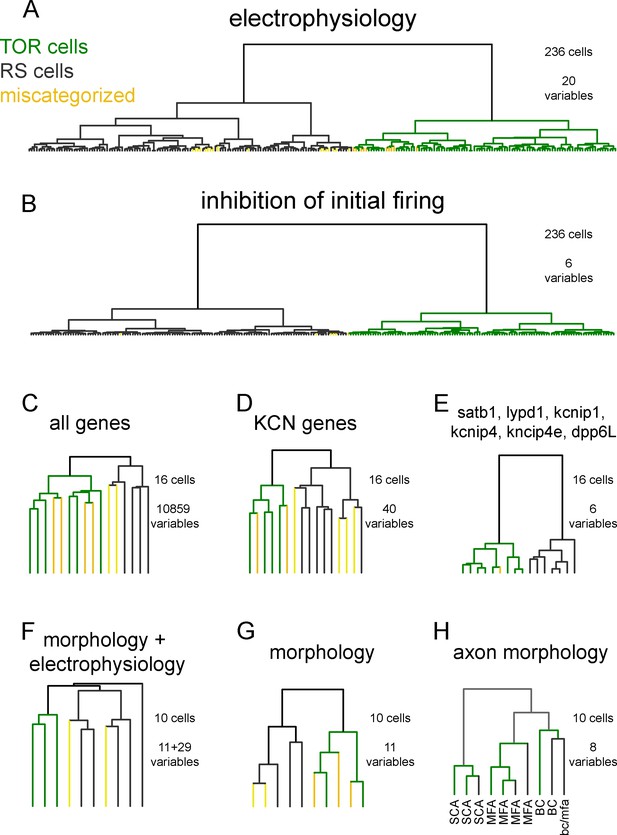
Hierarchical cluster analysis of CCK+INs.
(A) CCK+INs are categorized based on 20 general electrophysiological parameters and their membrane potential-dependent changes (for averages see Figure 1—source data 1). From the analyzed 236 CCK+INs, 27 cells (TOR and 10 RS) fell to the other cluster (labelled as miscategorized cells). In all cluster analyses, Ward method was used to determine Euclidean distances from normalized variable values. (B) When a narrower analysis was performed in the same 236 CCK+INs based on six parameters, which describes the inhibition of initial spiking, the number of miscategorized cells was 6. (C) Cluster analysis of 16 cells based on their SC-RNAseq determined active genes (10859 genes). (D) Cluster analysis of voltage-gated potassium channel genes of 16 CCK+INs. (E) Cluster analysis of the same 16 CCK+INs based on six genes and splicing isoforms, which emerged later in the study to correlate with TOR and RS identity. (F) Cluster analysis based on 11 morphological and 29 electrophysiological properties of ten 3-dimensionally reconstructed CCK+INs. (G) Cluster analysis considering only the morphology of the same cells shown in F. (H) Cluster analysis based on the axonal morphology of the reconstructed CCK+INs provides a control for the robustness of the method, as the same cells were correctly grouped them into well-established morphological categories. Notice that TOR and RS cells were found in each anatomical groups.
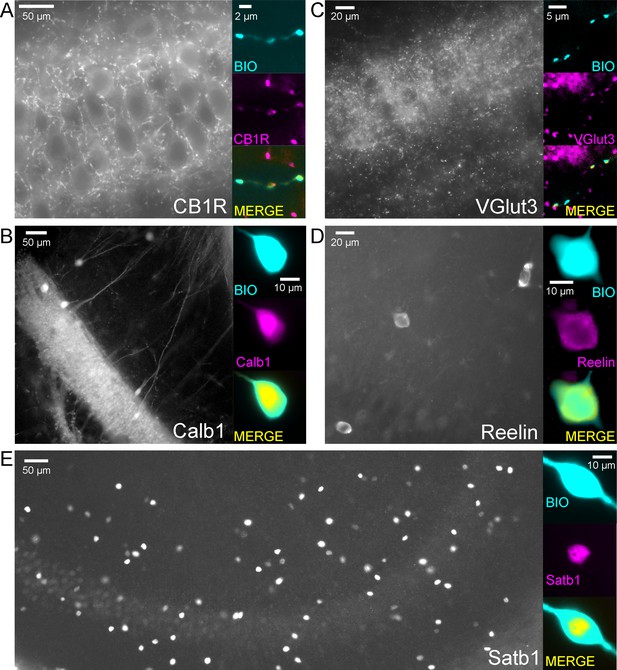
Example cells that were immunopositive for CB1R (A), Calb1 (B), VGluT3 (C), Reelin (D) and Satb1 (E) and the low magnification overview of the labelling pattern of these molecules within the CA3 region.
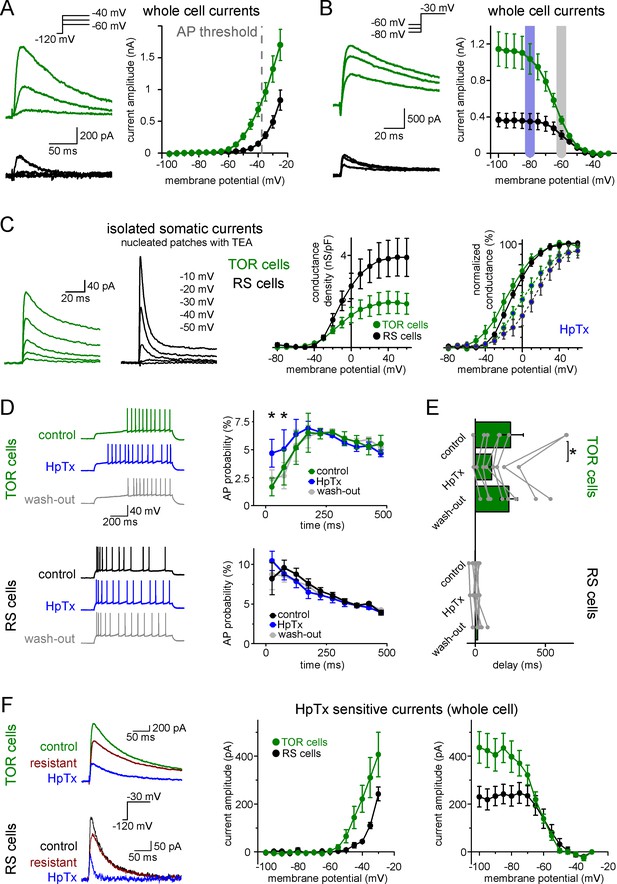
Differences in the Kv4-mediated ISA currents underlie the heterogeneity of CCK+IN firing.
(A) Representative traces from two single cells and average voltage dependence of activation of ISA in TOR (n = 17) and RS (n = 11) cells. The grey dotted line indicates AP threshold (−37.52 ± 0.3 mV) measured before TTX application. Notice the large amount of outward current in TOR cells at subthreshold MPs. (B) Representative traces and voltage dependence of inactivation of ISA at −30 mV from different holding potentials (n = 14 and 10 for TOR and RS cells, respectively). Blue and grey shaded areas indicate the voltage ranges from which state-dependent firing was tested (see Figure 1). (C) Representative traces and average voltage dependence of ISA conductance in nucleated patches from TOR and RS cells (n = 7 and 8, respectively). The activation curves are shown both as the average of raw and normalized data to highlight the differences both in the amount and voltage dependence of the conductance. Blue points connected in the right panel represent ISA currents in the presence of HpTX (1 µM). (D) Representative CCK+INs firing patterns recorded before, during and after HpTX application (in response to identical current steps) and the average AP probabilities in TOR (n = 6) and RS cells (n = 9) from hyperpolarized MP ranges (−82 to −77 mV). (E) Average delay of the first AP and reversible effect of HpTX. Connected symbols represent individual measurements (paired t-test: p=0.01). (F) HpTx-sensitive ISA in representative TOR and RS cells (left) and the average voltage dependence (n = 7 for both TOR and RS cells).
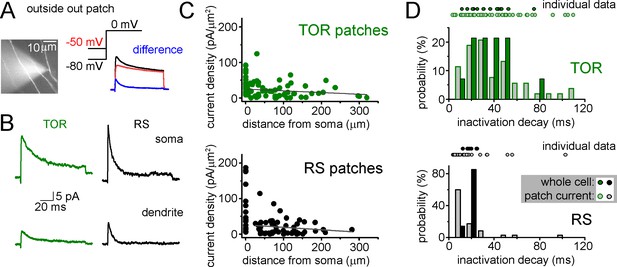
Representative outside-out patch recording of dendritic ISA current from a RS cell, which was somatically loaded with Alexa594 dye to visualize dendrites in epifluorescent illumination and to record firing pattern.
ISA current (blue) was evoked at 0 mV and isolated by subtracting currents evoked with −80 mV prepulse voltage from −50 mV prepulse. (B) Average ISA currents from somata and dendrites of RS and TOR cells (n = 22 somatic TOR-, n = 17 somatic RS-, n = 56 dendritic TOR and n = 53 dendritic RS patches). (C) Dendritic current densities did not show correlation with distance (TOR: R2 = 0.011, p=0.21, n = 56; RS: R2 = 0.01, p=0.22, n = 53, linear fit). Current densities were calculated by dividing the peak of ISA current with the membrane surface of the patch, which was calculated from the capacitance difference measured with the patch membrane and after pushing the pipette in an insulator gel (Sylgard). (D) Comparison of inactivation decay time constants of ISA measured in individual whole-cell and outside-out recordings. Symbols above the graphs show individual measurements and their distributions are represented by bar graphs. Notice the wider distribution of patch data compared to whole-cell data from TOR cells.
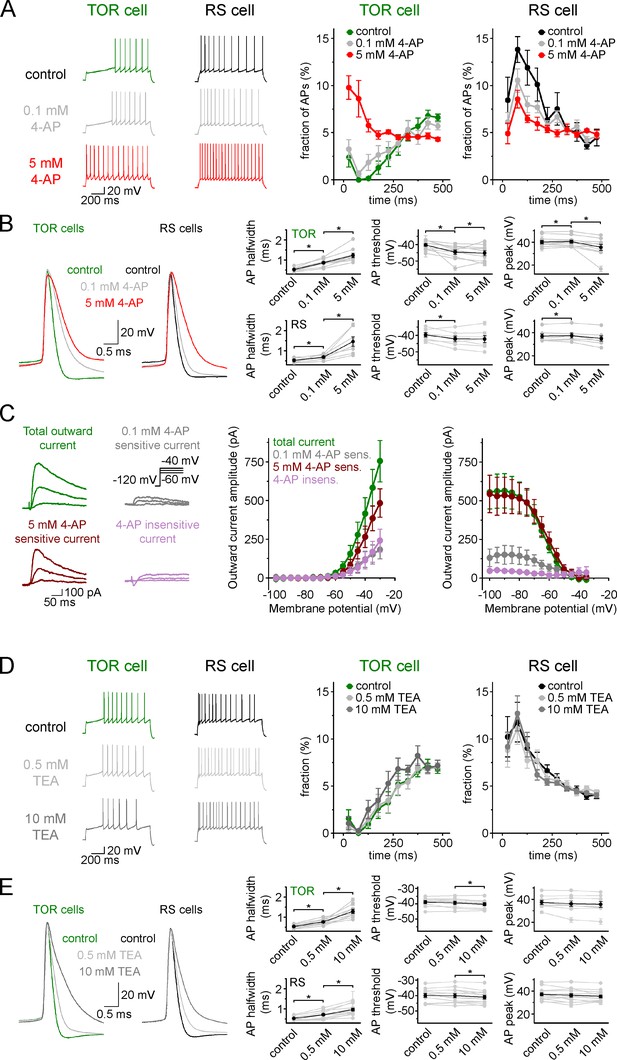
TOR phenomenon is not affected when Kv1 and Kv3 channels are inhibited.
(A) Inhibition of spiking in TOR cells was eliminated by only a high concentration of 4-aminopyridine (4-AP, 5 mM, red traces), but not by low concentration (0.1 mM, grey traces). (B) As expected, both low and high concentrations of 4-AP affect AP width and threshold due to blockade of various potassium channels (grey symbols represent individual experiments, black symbols show average results). (C) Representative traces on the left show control and 4-AP-sensitive and insensitive currents from a TOR cell and the graphs show the average voltage dependence of these components (n = 5 TOR cells). Majority (64.2% at −30 mV) of outward currents in TOR cells are sensitive to high, but not to low concentration 4-AP. (D) Firing patterns of TOR and RS cells are not affected by low (0.5 mM, light grey) and high concentrations (10 mM, dark grey) of tetraethylammonium (TEA, n = 9 TOR and n = 13 RS cells). (E) The effect of TEA on AP shape of TOR and RS cells (*: p<0.05, paired sample t-test).
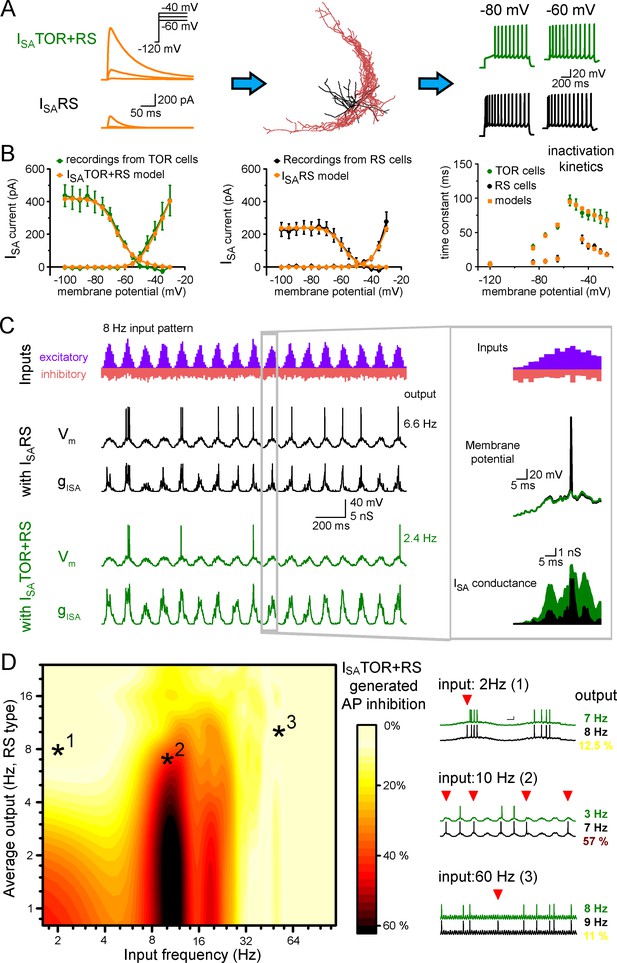
Different ISA currents in TOR and RS cells tune them for different network states.
(A) ISATOR+RS and ISARS were added to 10 reconstructed CCK+INs, which possess every known voltage-dependent conductance (Bezaire et al., 2016). Changing ISARS to ISATOR+RS in the same cells transformed the firing from RS to TOR phenotype. These simulated cells were equipped with synaptic conductance to simulate input drives in various network states. (B) Voltage clamp simulations with complete morphology and realistic conductances reproduced whole-cell ISA currents of TOR and RS cells, including voltage-dependence, the total current measured at the soma (left and middle graphs), and inactivation and the kinetics of inactivation and recovery from inactivation (right graph, where recovery was measured at −120,–85, −75 and −65 mV). (C) Representative traces showing the number and temporal distribution of 8 Hz-modulated synaptic inputs to simulated CCK+INs and its MP and ISA conductance in two conditions with either ISARS or ISATOR+RS. (D) Average effects of exchanging ISARS to ISATOR+RS on the output of 10 CCK+INs during various input frequency ranges (x-axis) and baseline output activity (i.e. with ISARS conductance, y-axis). Yellow color shows small change in firing when ISATOR+RS replaced ISARS, whereas red color indicates robust reduction in AP output. Representative traces on the right depict three examples with different input frequencies. Red triangles highlight inhibited spikes.
-
Figure 4—source data 1
Average conductance densities and membrane properties of ten simulated cells.
Each cell (n = 10) was simulated both as TOR and RS type by exchanging ISA only. With the exception of ISA, all parameters were identical in the two conditions for individual cells. Some parameters were fixed to decrease computational demand (gray), whereas other parameters were variable between cells to optimally reproduce the average firing properties. Fixed conductance values derived from previous publications.
- https://cdn.elifesciences.org/articles/58515/elife-58515-fig4-data1-v1.docx
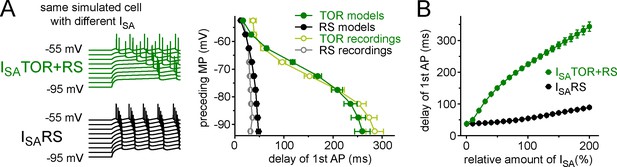
Realistic CCK+INs in-silico.
(A) Left, representative current-clamp simulations from a single model cell with either ISATOR+RS or ISARS currents. Notice the characteristic MP-dependence of spike onset with ISATOR+RS. The graph on the right summarizes the MP-dependence of timing of the first APs from 10 model cells with either ISATOR+RS or ISARS. The results from recordings using the same protocols are shown for comparison (from Figure 1C, open symbols). (B) Conductance density has little effect on the presence or absence and the extent of the TOR phenomenon suggesting that the kinetic and voltage-dependent properties of the two sets of ISA currents play a more important role in this phenomenon than the amounts of inhibitory conductance (measured from −80 mV preceding MP).
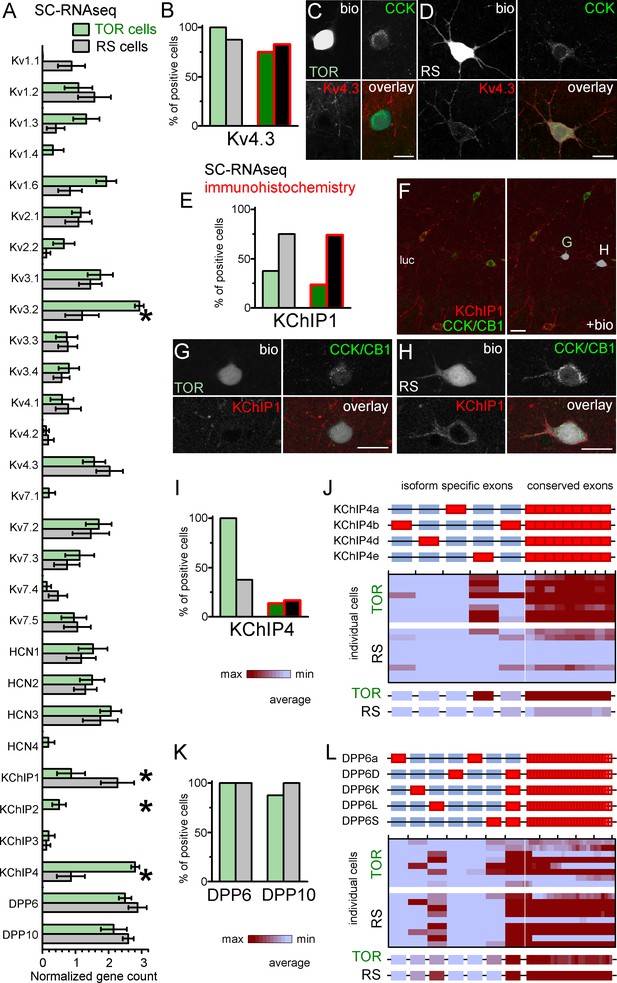
Similar Kv4.3-expression and different auxiliary subunit, KChIP and DPP-content in CCK+INs.
(A) Normalized gene count of primary and auxiliary subunits of voltage-gated potassium channels from single cell RNAseq data of TOR (n = 8) and RS (n = 8) cells. (B) Percentage of recorded cells with detectable levels of Kv4.3 mRNA (left bars, n = 8 and 9 cells) and protein (right bars, n = 20 and 23 tested cells). (C–D) Immunofluorescent co-localization of CCK and Kv4.3 in a TOR (C), and a RS cell (D) in CA3 stratum lucidum. (E) Percentage of recorded cells with detectable levels of KChIP1 mRNA (n = 8 and 8 cells) and proteins (n = 20 and 27 cells). (F–H) Immunofluorescent co-localization of CCK with CB1 (green) and KChIP1 in a TOR (G), and a RS cell (H) from the same slice shown in low magnification image (F). (I) Percentage of recorded cells with detectable levels of KChIP4 mRNA (left bars, n = 8 and 8 cells) and protein (right bars, n = 29 and 30 tested cells). (J) Major KChIP4 splicing isoforms consist of different exons in the N-terminal region (represented as red boxes). Each row represents a single cell in the color-mapped data and columns correspond to individual exons aligned to the schematic illustration of isoforms above. Red and blue colors code high and low mRNA levels, respectively. The average exon counts from the two types of CCK+INs (n = 8 and 8) are shown at the bottom using the same color code scheme. (K) Percentage of recorded cells with detectable levels of DDP6 and DPP10 mRNA. (L) Assembly of major DPP6 isoforms and exon levels in individual CCK+INs are shown as above (J).
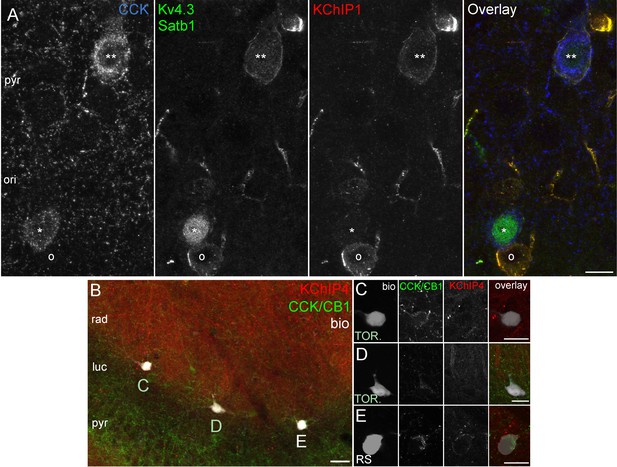
Kv4.3 and KChIP immunohistochemistry in perfusion or immersion fixed CA3 slices.
(A) CCK+ cells in CA3 obtained from perfusion fixed brain. One CCK+ cell (**) is outlined by intense Kv4.3 and KChIP1 immunolabelling. In the other CCK+ cell (*) that is strongly labelled for Satb1 in the nucleus, the Kv4.3 and KChIP1 signals are only weakly detectable, whereas they both intensely decorate a neighboring CCK-negative neuron (o). (B) Low magnification image showing simultaneous immunolabelling of KChIP4 (red) and CCK/CB1 (green) in an acute CA3 slice, in which a RS cell and two TOR cells were filled with biocytin (left) at the border of strata radiatum/lucidum in CA3a. Intense KChIP4 immunolabelling is present in the neuropil of stratum radiatum mainly associated with dendrites of pyramidal cells (Rhodes et al., 2004). (C–E) High magnification images showing an enlarged view of the biocytin filled cells shown in (B). KChIP4 is not detectable in the two TOR cells (C, D), but its weak presence was detected in the neighboring the RS cell (E), but. Scale bars: 10 µm (A) 20 µm (B–E).