Gap junction-mediated glycinergic inhibition ensures precise temporal patterning in vocal behavior
Figures
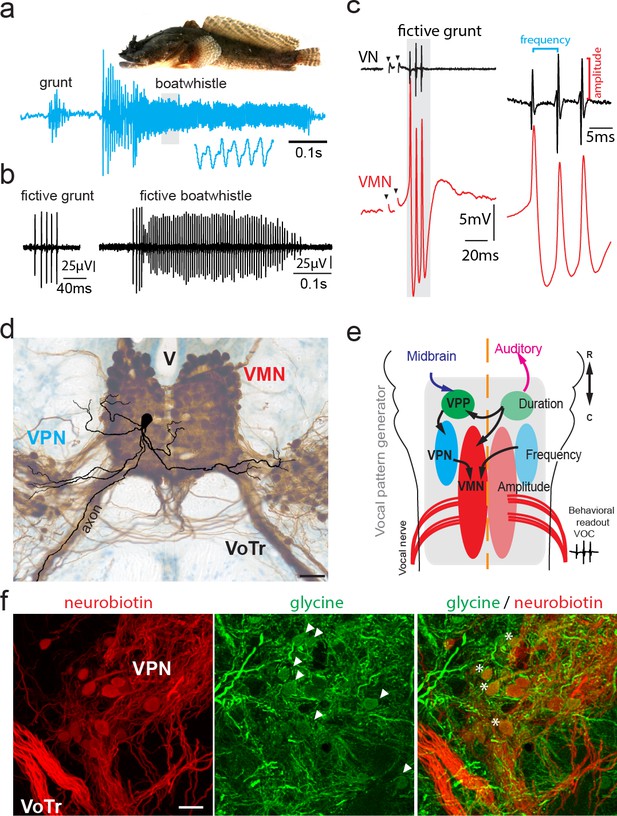
Toadfish vocalization and underlying vocal motor circuity, which includes electrotonically coupled glycinergic neurons.
(a) Photograph of Gulf toadfish (courtesy of Aaron Rice, Cornell Lab of Ornithology) and waveform of toadfish vocalization (blue) composed of a grunt and a boatwhistle recorded with a hydrophone. Lower waveform is magnified from region outlined by gray box. (b) Spontaneous fictive vocalizations (fictive grunt and boatwhistle) recorded from the vocal nerve (VN). (c) VN compound potential (fictive call VOC, here a grunt) and intracellular vocal motor nucleus (VMN) motoneuron recording show highly time-locked activity. Right side shows higher magnification and close correlation between motoneuronal action potentials and VN spikes. (d) Photograph of transverse hindbrain section showing reconstructed VMN motoneuron superimposed over neurobiotin-filled VMN and vocal pacemaker nucleus (VPN) (dark brown); cresyl violet counterstain. Scale bar in (d) represents 100 µm. (e) Dorsal schematic view of caudal hindbrain showing toadfish vocal motor circuit comprising three anatomically separate nuclei coding for different attributes: duration coding vocal prepacemaker nucleus (VPP), frequency (pulse repetition rate) coding VPN and amplitude coding VMN. VNs innervate muscles used in sound production. (f) Photomicrographs of neurobiotin-labeled (red) VPN neurons and vocal tract (VoTr) (left), glycinergic-immunoreactive (green) neurons (somata indicated by white arrowheads) and fibers (middle), and overlay of both (right). A subset of glycinergic neurons in the VPN are co-labeled with neurobiotin (asterisks); see Rosner et al., 2018 for detailed quantification. The scale bar is 20 µm.
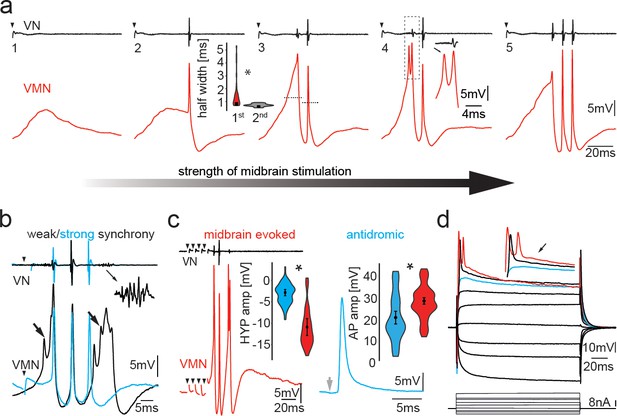
Activity in vocal motoneurons reveals prominent differences in action potential shape depending on how action potentials are elicited.
(a) Intracellular recordings of a single vocal motor nucleus (VMN) motoneuron showing different stages of activity in the generation of vocal nerve (VN) motor volley, the fictive vocalization, elicited by midbrain electrical stimulation. Violin plot shows the half width (hatched lines in trace 3) of the first and second VMN motoneuron action potentials (APs, for eight neurons). Note the double spikelets that occasionally occur on first APs (inset in 4). (b) Weak (black traces) and strong (blue traces) synchrony of vocal activity is reflected in VN compound potentials (top) and the voltage of intracellularly recorded VMN motoneuron APs (bottom). Electrical artifacts of midbrain stimulation are indicated by black arrowheads. Black arrows indicate spikelets. Inset shows magnification of unsynchronized motor output. (c) Amplitude of VMN motoneuron hyperpolarization (HYP) and AP amplitudes are higher during fictive vocal behavior (red trace) than during antidromic stimulation (blue trace) via the VN. (d) Current voltage responses of a VMN neuron show rapid AP adaptation. Inset shows color-coded traces just before AP initiation (blue), with one (black) and two (red) APs. Note the decrease in second AP height in the red trace. Violin plots show distribution of data from all recordings with estimated means and standard error from the nested mixed model. Asterisks here and in Figures 4, 5, and 7 indicate significant differences.
-
Figure 2—source data 1
Comparison between motoneuron action potential and hyperpolarization amplitude during midbrain-evoked vocal and antidromic stimulation.
- https://cdn.elifesciences.org/articles/59390/elife-59390-fig2-data1-v1.xlsx
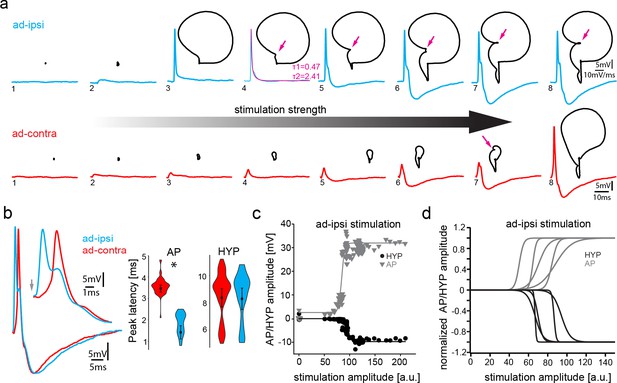
Antidromic stimulation activates motoneuronal hyperpolarization (HYP) that depends on stimulation amplitude.
(a) Intracellular record of antidromically activated motoneuron upon ipsi- (blue) and contralateral (red) activation with increasing stimulation amplitude (schematized by big horizontal arrow). Black lines show phase plane plots, and magenta arrows indicate additional depolarization prior to HYP onset. Magenta line in fourth trial represents exponential fit for this recording, with the constants indicated. Scale bars on top row for phase plane plots, bottom row for color traces. (b) Overlay of ipsi- and contralateral stimulation of neuron shown in (a). Violin plots show HYP and action potential (AP) peak latencies. (c) AP (gray) and HYP (black) peak amplitude of VMN neuron response upon ipsilateral antidromic stimulation of variable amplitude (arbitrary units) and corresponding sigmoid fits (color coded lines). (d) Normalized sigmoid fits of different neurons showing differences in recruitment threshold by antidromic stimulation, but similar time courses.
-
Figure 3—source data 1
Comparison between motoneuron action potential and hyperpolarization latency during midbrain-evoked vocal and antidromic stimulation.
- https://cdn.elifesciences.org/articles/59390/elife-59390-fig3-data1-v1.xlsx
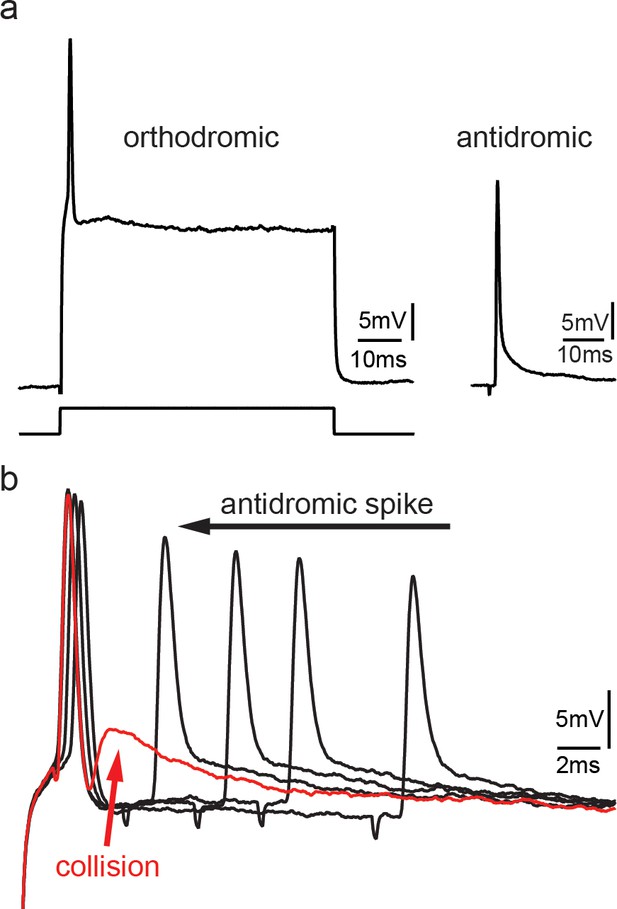
Collision experiments reveal gap junctional coupling in vocal motoneurons.
(a) Action potential firing in a vocal motoneuron after intracellular current injection (left) and antidromic stimulation via the vocal nerve (right). (b) Combination of intracellular current injection and antidromic stimulation at different latencies (black arrow) reveal a depolarizing potential when action potentials collide (red trace and arrow).
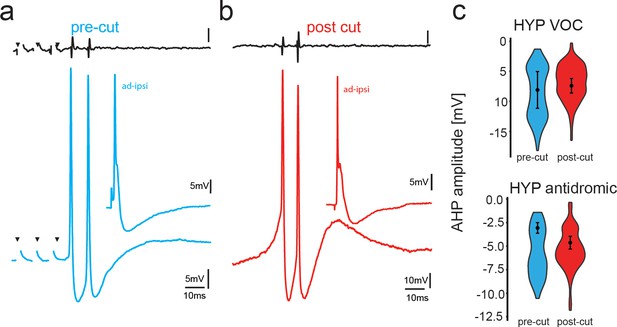
Potential reafferent input via the dorsal roots does not contribute to the motoneuronal hyperpolarization (HYP).
(a,b) Midbrain-evoked VOC recording from vocal nerve (top trace, black) and intracellular recording in a vocal motoneuron before (blue) and in another motoneuron in the same fish after transection of the dorsal root input to the hindbrain (red). Inset shows antidromic stimulation of the respective motoneurons. (c) Box and whisker plots of hyperpolarization amplitude during vocal activity (VOC) and antidromic stimulation. Pre-cut (blue) and post-cut (red) conditions are indicated.
-
Figure 3—figure supplement 2—source data 1
Comparison between motoneuron action potential and hyperpolarization amplitude during baseline conditions and after cutting the dorsal roots.
- https://cdn.elifesciences.org/articles/59390/elife-59390-fig3-figsupp2-data1-v1.xlsx
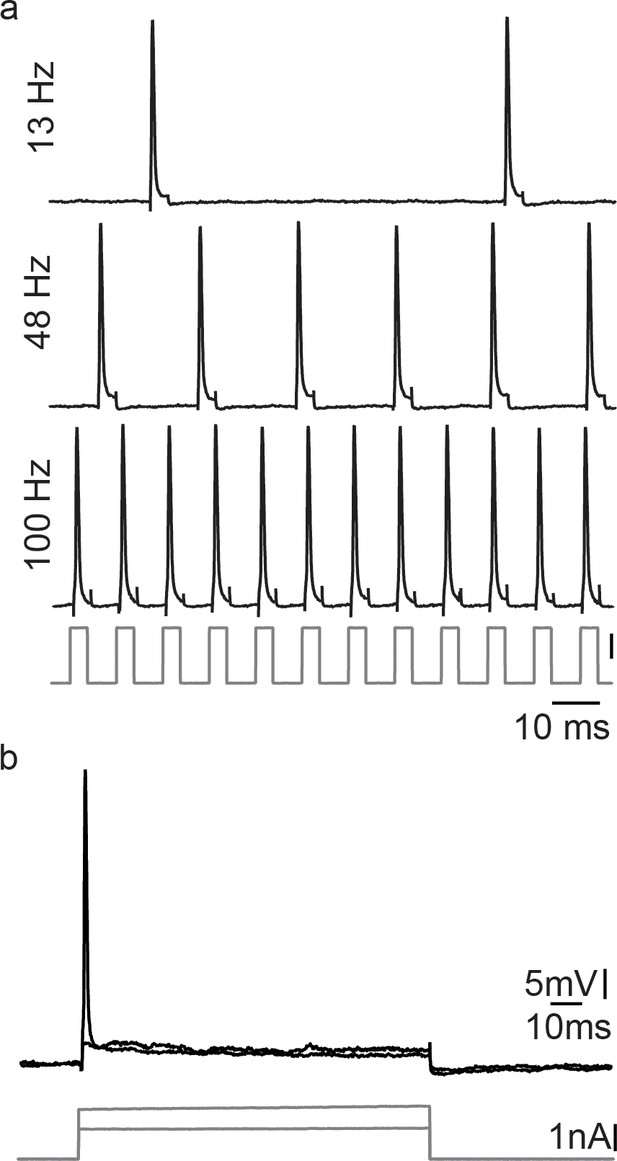
Motoneurons are able to repetitively fire under a pulse train condition, but fail in response to a permanent current injection, revealing the necessity of a hyperpolarization for correct vocal patterning.
(a) Action potential firing after short intracellular current pulse injection into a vocal motoneuron (at different stimulation frequencies). (b) Intracellular long-lasting current injection into the same vocal motoneuron shows the necessity of repeated pulse stimulation to repetitively elicit action potentials in vocal motoneurons.
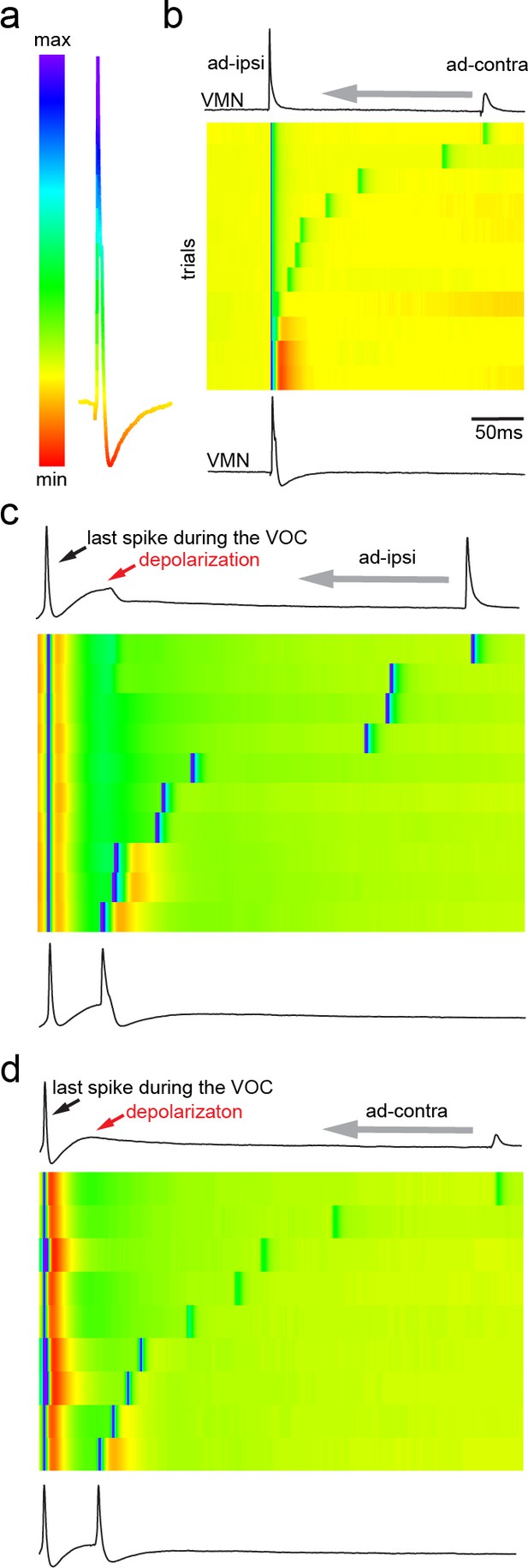
Antidromic activation of vocal motor nucleus (VMN) motoneurons revealing network-dependent hyperpolarization (HYP).
(a) Antidromic-evoked action potential (AP) color-coded to relative voltage amplitude and corresponding color bar. (b) Stimulation-dependent voltage matrix (SDVM; color code given in [a]) of ipsilateral antidromic activation followed by contralateral antidromic activation (that generated only a depolarizing potential) of decreasing latency revealing the appearance of a HYP when ipsilateral and contralateral stimulation overlap. In this situation, ipsilateral antidromic stimulation was set to elicit an AP without a HYP. With decreasing distance of the contralateral stimulation, a HYP started to appear (inset in SDVM). Top and bottom black lines represent first and last rows of the SDVM in this and in the following panels. (c) SDVM showing last compound potential of a fictive vocalization (VOC) with associated depolarization (depol) and antidromic stimulation (set to elicit only a depolarization) of ipsilateral vocal nerve with decreasing latency. Ipsialateral potential generated an AP with decreasing distance to the depolarization that was accompanied by a HYP with further decrease in latency. (d) As in (c) but with contralateral antidromic stimulation.
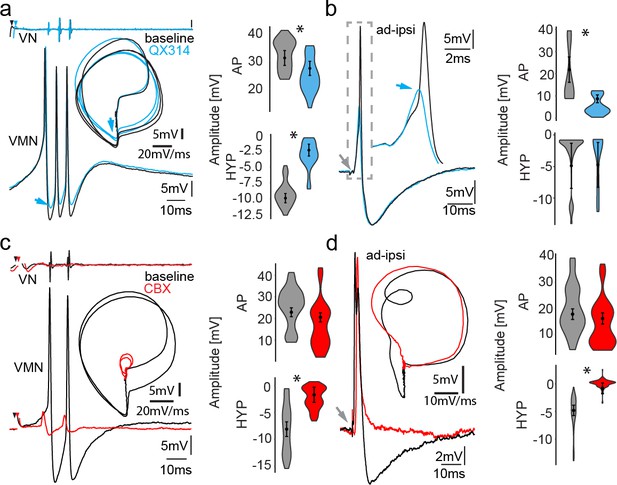
Network activity and gap junctional coupling are essential to generate the motoneuron hyperpolarization (HYP).
(a, b) Baseline (black) intracellular recording of vocal motor nucleus (VMN) neuron action potentials (APs) and with QX314-filled electrodes (blue) during fictive vocal activity (top trace vocal nerve, VN) (a) and ipsilateral antidromic stimulation (b). Inset in (a) shows phase plane plot and in (b) higher magnification of antidromically evoked APs. Violin plots show percent change from baseline level for AP and HYP during either condition. Blue arrows point to the differences in AP and HYP levels. (c, d) Intracellular recording of one VMN neuron before (black) and one VMN neuron after (red) blocking gap junctions by application of carbenoxolone (CBX). Inset shows phase plane plot. Violin plots show amplitude decrease in baseline for AP and HYP during either condition. Plot shape shows distribution of data from all recordings. Center dot and error bars show estimated means and standard error derived from the nested mixed model. Gray arrow in(b) and (d) indicates stimulus onset.
-
Figure 5—source data 1
Comparison between motoneuron action potential and hyperpolarization amplitude during midbrain-evoked vocal and antidromic stimulation for QX314 treatment and carbenoxolone treatment.
- https://cdn.elifesciences.org/articles/59390/elife-59390-fig5-data1-v1.xlsx
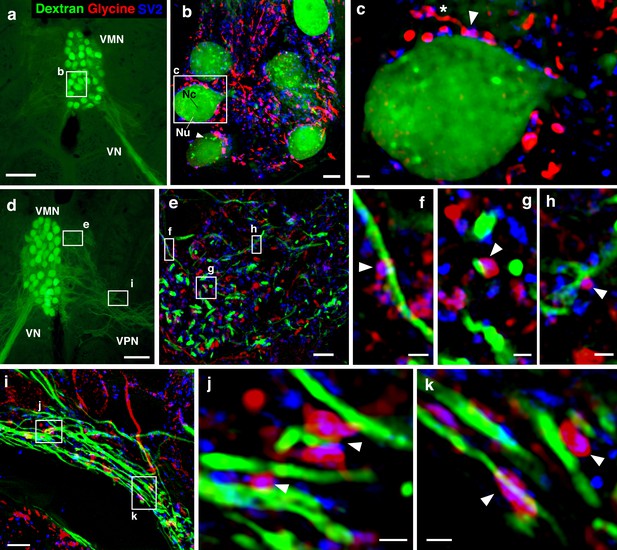
Glycinergic input on motoneuron somata and dendrites reveals an anatomical substrate for the motoneuronal hyperpolarization.
(a, d) Widefield micrographs show dextran-filled motoneurons (green) in the vocal motor nucleus (VMN) of two different fish. Dextran labeling of one vocal nerve (VN) results in filling of ipsilateral VMN somata and dendrites that extend into the contralateral VMN (d, box e) and bilateral into adjacent VPN columns (d, box i; also see Figure 1c). White boxes correspond to maximum projections of super-resolution structured illumination microscopy (SR-SIM) z-stacks (b, e, i) that show glycine (red) and synaptic vesicle protein 2 (SV2, blue) immunoreactive (-ir) label in proximity to VMN somata (b) and dendrites (e, i). (b) VMN somata are surrounded by dense glycinergic and SV2-ir puncta. A large nucleus (Nu) with nucleolus (Nc) is evident in several somata; punctate dextran labeling within the cytoplasm (white arrowhead) likely indicates sequestration of dextran into vesicles. (c) A single optical section (0.1 µm) from SR-SIM z-stack in (b) shows a glycinergic-ir fiber (white asterisk) forming a bouton (white arrowhead) on a motoneuron soma. Overlap of glycine and SV2-ir (magenta, white arrowhead) in the bouton indicates a site of neurotransmitter release and a likely synapse. Additional optical sections show glycinergic release sites (white arrowheads) on motoneuron dendrites extending into the contralateral VMN (f, g, h, corresponding to boxes in e) and bilaterally into VPN columns (j, k, corresponding to boxes in i). Scale bars represent 100 µm in widefield micrographs (a, d), 5 µm in SR-SIM maximum projections (b, e, i) ,and 1 µm in SR-SIM optical sections (c, f, g, h, j, k).
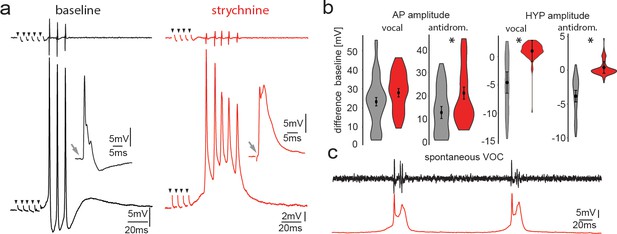
Pharmacological blocking of glycinergic input with strychnine shows its importance in vocal patterning.
(a) Intracellular recordings from a motoneuron before (black) and another motoneuron after (red) strychnine injection into vocal motor nucleus (top trace vocal nerve). Insets show antidromic-evoked action potentials for the respective neurons. (b) Violin plots showing the change in amplitude of the action potential (AP) and the hyperpolarization (HYP) for control (gray) and strychnine injected (red). (c) Intracellular recordings of a motoneuron (red trace) during two spontaneous fictive vocalizations (VOC, black trace).
-
Figure 7—source data 1
Comparison between motoneuron action potential and hyperpolarization amplitude during midbrain-evoked vocal and antidromic stimulation for strychnine treatment.
- https://cdn.elifesciences.org/articles/59390/elife-59390-fig7-data1-v1.xlsx
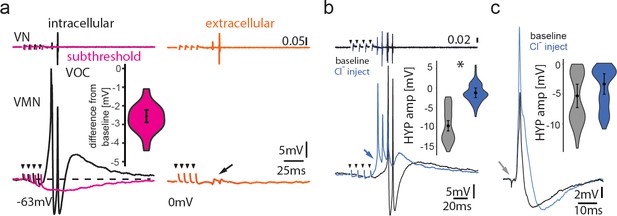
Inhibitory input is present in the motoneuronal network.
(a) Intracellular recording of vocal motor nucleus (VMN) motoneuron during midbrain subthreshold stimulation (top trace vocal nerve, VN) shows decrease in membrane potential (magenta trace) compared to during vocal activity (black trace) and extracellular-recorded activity (red trace). (b,c) Intracellular chloride injection into one motoneuron to modify chloride reversal potential shows prominent difference in amplitude during vocal activity (b), but not during antidromic stimulation (c). Box plots show change in amplitude of hyperpolarization (HYP; percent change from baseline levels).
-
Figure 7—figure supplement 1—source data 1
Motoneuron hyperpolarization amplitude at subthreshold to vocal activity and before and after chloride injection.
- https://cdn.elifesciences.org/articles/59390/elife-59390-fig7-figsupp1-data1-v1.xlsx
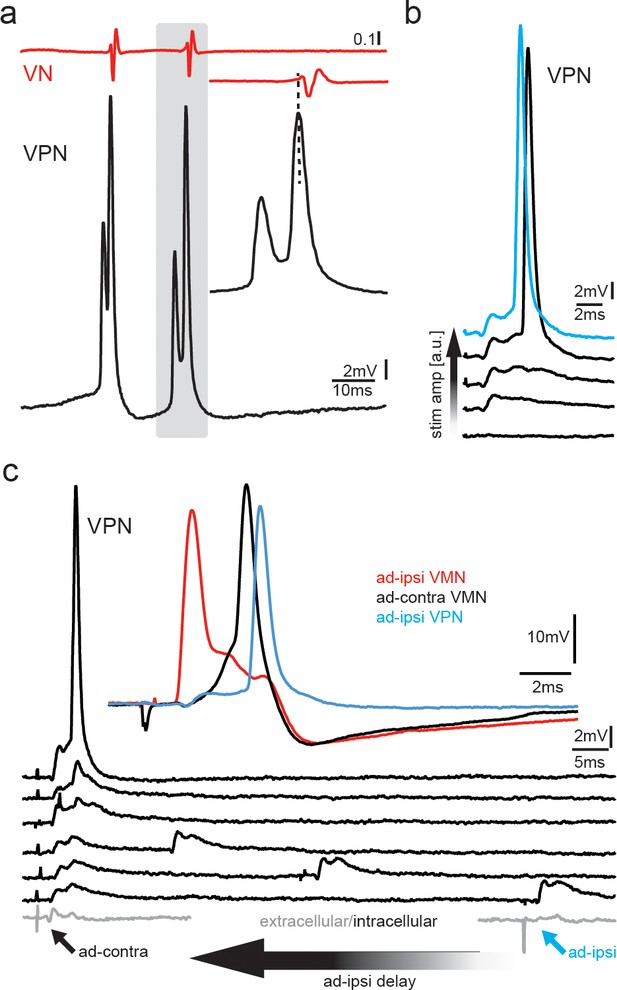
Premotoneurons can be activated via gap junctional coupling, thus revealing their potential to contribute to the motoneuron hyperpolarization.
(a) Intracellular recording of vocal pacemaker nucleus (VPN) neuron during fictive vocal activity shows the characteristic double spiking of VPN neurons. Inset shows timing between vocal nerve (VN) and VPN neuron activity. (b) Waterfall plot of intracellular VPN recordings during ipsilateral antidromic vocal nerve stimulation leads to depolarizing potentials in VPN neurons that eventually lead to action potential (AP) firing with increasing stimulation amplitude. (c) Waterfall plot of intracellular recordings from a VPN neuron during antidromic stimulation of the ipsi (ad-ipsi) and contralateral (ad-contra) vocal nerve. With decreasing latency between the ad-contra and ad-ipsi stimulation (at identical stimulation amplitudes), an AP can be elicited, showing the capability of gap junctional coupling to induce AP firing. Inset shows recordings from a VMN neuron during antidromic stimulation of the contralateral and ipsilateral sides and from a VPN neuron during ipsilateral antidromic stimulation (not simultaneously recorded).

Summary of proposed action of glycinergic vocal pacemaker nucleus (VPN) neurons in the activation sequence generating vocal behavior.
Temporal activation of the hyperpolarization strongly suggests a feed-forward inhibitory role. Antidromic experiments indicate the possibility of a feedback mechanism that might affect temporal patterning as seen in other motor systems. VPP: vocal prepacemaker nucleus; VMN: vocal motor nucleus; PRR: pulse repetition rate.
Videos
Intracellular recording of a vocal motoneuron during sequences of repeated antidromic stimulation via the vocal nerve on the ipsilateral and contralateral sides (both subthreshold to network activation of glycinergic input).
The latency between the ipsi- and contralateral stimulation was diminished, which eventually led to the generation of a hyperpolarization via glycinergic input.
Intracellular recording of a vocal motoneuron during sequences of repeated antidromic stimulation via the vocal nerve on the ipsilateral and contralateral sides.
The latency between the ipsi- and contralateral stimulation was diminished, which eventually abolished the activation of the glycinergic input as motoneurons were hyperpolarized.
Tables
Reagent type (species) or resource | Designation | Source or reference | Identifiers | Additional information |
---|---|---|---|---|
Strain, strain background | Opsanus beta | Gulf specimens (https://gulfspecimen.org/) | Specimens taken from the wild | |
Antibody | Anti-rabbit AlexaFluor 568, polyclonal | Thermo Fisher | RRID:AB_143157 | 1:200 |
Antibody | Anti-rabbit AlexaFluor 568, polyclonal | Thermo Fisher | RRID:AB_2535805 | 1:200 |
Antibody | Anti-glycine, rabbit, polyclonal | MoBiTec | RRID:AB_2560949 | 1:200 |
Antibody | Anti-synaptic vesicle protein 2, monoclonal | Developmental Studies Hybridoma Bank | RRID:AB_2315387 | 1:50 |
Peptide, recombinant protein | 488 anti-streptavidin | Jackson ImmunoResearch Labs | RRID:AB_2337249 | 1:500 |
Software, algorithm | IGOR PRO | Wavemetrics | RRID:SCR_000325 | |
Software, algorithm | pClamp | Molecular devices | RRID:SCR_011323 | |
Software, algorithm | NeuroMatic | http://www.neuromatic.thinkrandom.com | RRID:SCR_004186 |
Additional files
-
Supplementary file 1
Summary of statistical results.
- https://cdn.elifesciences.org/articles/59390/elife-59390-supp1-v1.xlsx
-
Transparent reporting form
- https://cdn.elifesciences.org/articles/59390/elife-59390-transrepform-v1.docx