Somatostatin-expressing parafacial neurons are CO2/H+ sensitive and regulate baseline breathing
Figures
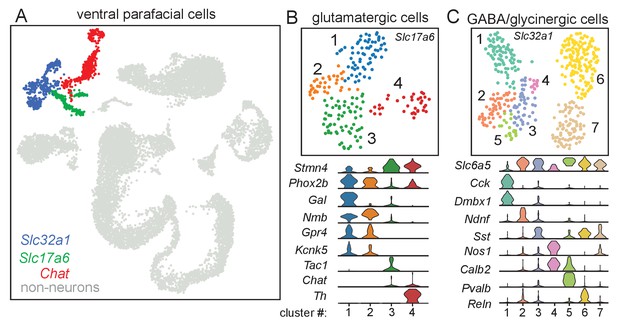
Molecular signatures of ventral parafacial glutamatergic and inhibitory neurons.
(A) Through a normalized dispersion analysis for dimension reduction, a t-distributed stochastic neighbor embedding (t-SNE) was created of ventral parafacial single-cell transcriptome, with cells color coded by cluster. Neurons were differentiated from non-neurons (gray) based on expression of Snap25, Syp, Tubb3, and Elavl2. Cells expressing either Slc17a6 or Slc32a1 were used for sub-cluster analysis of glutamatergic and inhibitory neurons. A fairly large population of Chat expressing neurons was also detected but since neither glutamatergic (B) nor Vgat+ neurons express Chat, this population was not analyzed further. (B) UMAP plot depicting four sub-clusters of glutamatergic neurons and corresponding violin plots showing cluster-specific differential gene expression. Cluster number is noted on the x axis and gene expression (from 0 to 4 counts/cell) on the y axis. Slc17a6 clusters 1–2 are presumed to be subsets of RTN chemoreceptors based on expression of Phox2b, Nmb, Gpr4, and Kcnk5 that differ in expression of galanin. Slc17a6 cluster 3 differentially expressed Tac1 (gene encoding substance P), suggesting that these cells may be parapyramidal raphe neurons, whereas cluster four differentially expressed tyrosine hydroxylase (Th) indicative of adrenergic C1 pre-sympathetic neurons. (C) UMAP plot showing seven sub-clusters of inhibitory (Slc32a1) neurons and corresponding violin plots showing cluster-specific differential gene expression. Cluster number is noted on the x axis and gene expression (from 0 to 4 counts/cell) on the y axis. Discrete subtypes of inhibitory neurons were identified based on non-overlapping expression of cholecystokinin (Cck; Slc32a1 cluster 1), neuron-derived neurotrophic factor (Ndnf, Slc32a1 cluster 2) and parvalbumin (Pvalb; Slc32a1 cluster 5). Three somatostatin (Sst+) clusters could be differentiated based on expression of calretinin (Calb2; Slc32a1 cluster 3), reelin (Reln, Slc32a1 cluster 6) and neuronal nitric oxide synthase 1 (Nos1, Slc32a1 clusters 3, 7).
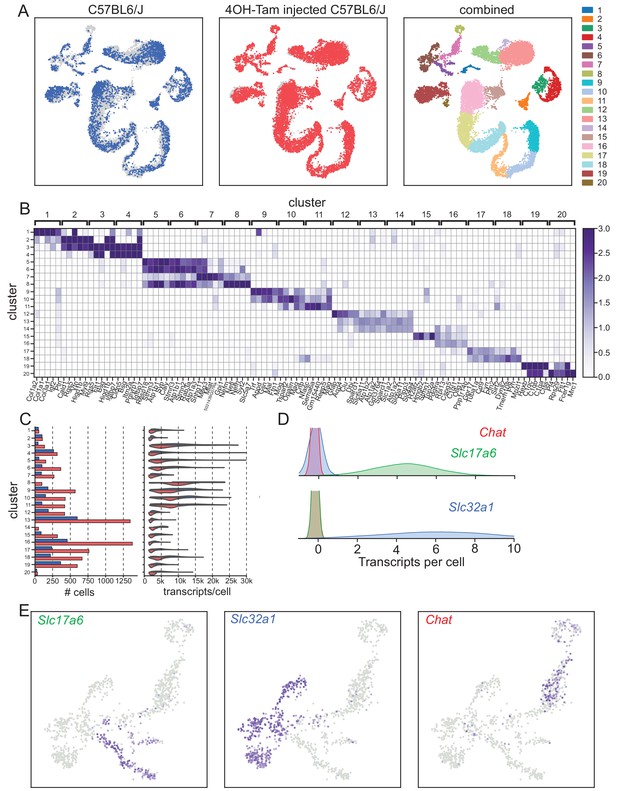
Bioinformatic Pipeline used for single-cell RNA-seq analysis.
(A) Global analysis showing the distribution of cells between the two libraries, C57BL/6J pups (left) and 4OH-tamoxifen-treated C57BL/6J pups (center), and global clustering (right). (B–C) marker gene expression (B), each box representing the mean expression of each gene in each cluster; total number of cells and distribution of transcripts per cell (C), across the 20 global clusters. (D) A distribution of Slc17a6 and Slc32a1 expression of cells in each class of the three-state Gaussian mixture model used to classify neuronal cells; blue, green, and red colors of distribution correspond with Slc32a1, Slc17a6, and Chat, respectively in Figure 1A. (E) Expression of Slc17a6 (left), Slc32a1 (center), Chat (right) in cells classified as neurons for sub-clustering analysis.
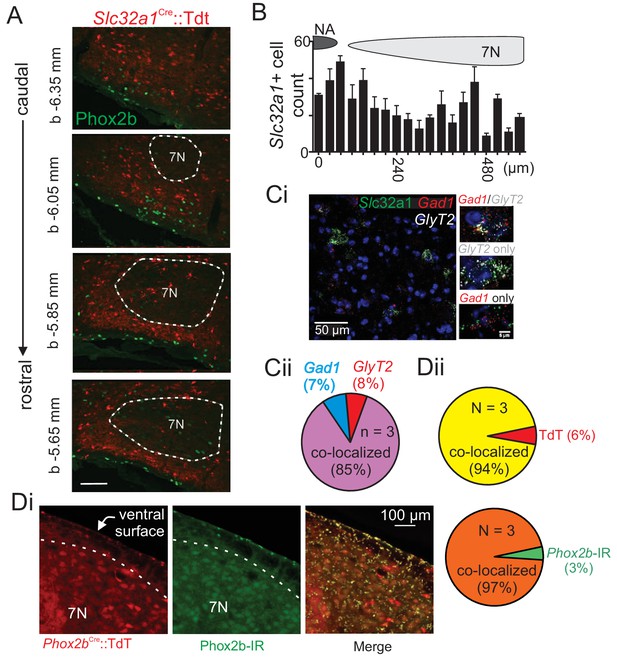
Ventral parafacial inhibitory neurons are located in close proximity to RTN chemoreceptors.
(A) Photomicrographs of coronal sections from a Slc32a1Cre::TdT mouse show TdT-labeled inhibitory neurons (red; Slc32a1Cre) and Phox2b-immunoreactive chemosensitive RTN neurons (green) across the rostrocaudal extent of the RTN region and in the caudal portion of the RTN where most chemosensitive neurons are located. Approximate millimeters behind bregma (Ascoli et al., 2008) indicated by numbers in lower left of each section. (B) Top, schematic showing brainstem regions used as landmarks to align sections for cell counts. Bottom, quantification of the total number of Slc32a1Cre::TdT labeled neurons in each section (four mice). (Ci) Images of RNA in situ hybridization results show Vgat cells (green) Gad1 (red), GlyT2 (white), and DAPI (blue). (Cii) Quantification of Gad1 and GlyT2 co-localization (three animals/5 brain slices). (D) Representative images (Di) and summary data from three Phox2bCre::TdTomato mice (Dii) show that most Phox2b-immunoreacive (IR) neurons (green) near the ventral surface express TdTomato fluorescence (red). Specifically, 97% of all ventral parafacial TdT+ cells are Phox2b-IR, and 94% of all Phox2b-IR cells are TdT+. NA, nucleus ambiguous; 7N, facial motor nucleus, b-bregma.
-
Figure 1—figure supplement 2—source data 1
Raw rostral to Caudal Slc32a1Cre::TdT cell count.
- https://cdn.elifesciences.org/articles/60317/elife-60317-fig1-figsupp2-data1-v2.xlsx
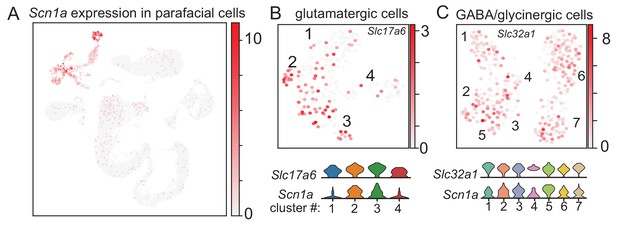
Scn1a transcript is expressed by sub-clusters of glutamtergic and GABA/glycinergic parafacial neurons.
(A) Same t-SNE plot of parafacial cell clusters described in Figure 1 shows that Scn1a transcript is preferentially expressed by Slc17a6+ (Vglut2), Slc32a1+ (Vgat), and Chat+ (cholinergic) neurons compared to non-neural cell types. (B–C) UMAP plots (as described in Figure 1B–C) show sub-clusters of glutamatergic (B) and inhibitory (C) neurons and corresponding violin plots depict relative levels of Scn1a transcript in each group. Cluster number is noted on the x axis and gene expression (from 0 to 4 counts/cell) on the y axis. These results are consistent with the possibility that Scn1a is expressed by both excitatory and inhibitory cell types in this brainstem region (Kuo et al., 2019), albeit at relatively higher levels in inhibitory neurons compared to glutamatergic neurons at this developmental time point (10 days postnatal).
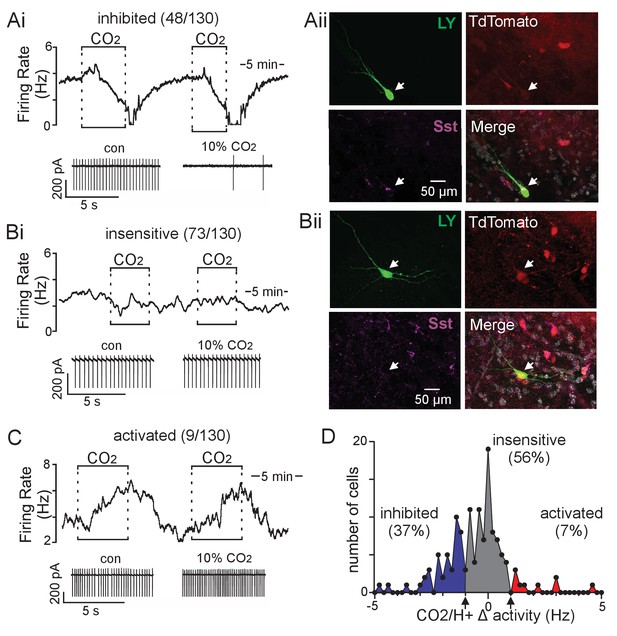
Somatostatin neurons in the ventral parafacial region are CO2/H+-sensitive.
(A-C) Traces of firing rate and segments of holding current from ventral parafacial inhibitory neurons in slices from Slc32a1Cre::TdT mice show that exposure to 10% CO2 suppressed activity by 2.0 ± 0.2 Hz in 43% of neurons tested (Ai), whereas the majority of Slc32a1+ neurons in this region did not respond (Δ firing 0.11 ± 0.04 Hz) to this same level of CO2/H+ (Bi), and a small minority are activated by CO2/H+ (C). (Aii) Example of a CO2/H+-inhibited Lucifer Yellow filled parafacial neuron that was Sst-immunoreactive (IR). (Bii) Example of a CO2/H+-insensitive Lucifer Yellow filled parafacial neuron that was not Sst-IR. In sum, 5 of 5 CO2/H+-inhibited parafacial neurons were Sst-IR, whereas 0 of 5 CO2/H+-insensitive neurons in this region were Sst-IR. (D) Summary data (N = 130 neurons from 45 mice) plotted as number of cells vs mean firing response to 10% CO2. Note that all neurons included in this analysis showed a similar baseline level of activity in 5% CO2 (data not shown).
-
Figure 2—source data 1
Parafacial inhibitory neuron responses to CO2/H+.
- https://cdn.elifesciences.org/articles/60317/elife-60317-fig2-data1-v2.xlsx
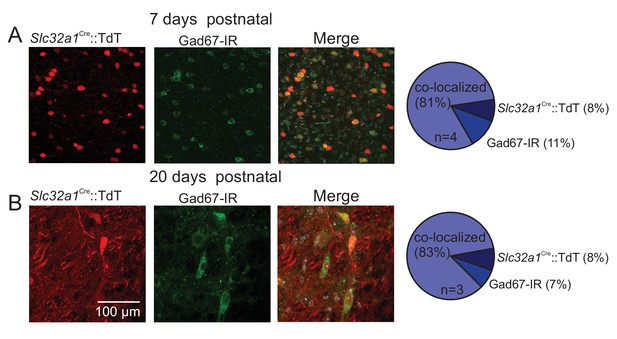
Validation of the Slc32a1Cre mouse line.
(A-B) Summary data and representative images of brainstem slices (75 µm) from Slc32a1Cre::TdT pups at 7 (A) and 20 days (B) postnatal show that TdTomato fluoresce (red) and Gad67-immunoreactity (IR; green) co-label 81% and 83% of parafacial neurons, respectively. At both developmental time points 8% of TdTomato fluoresce cells were not Gad67-IR, suggesting cells other than inhibitory neurons may express Cre recombinase. This is not entirely unexpected since astrocytes are also known to express can express Slc32a1 (Wang et al., 2013b). In any case, this is a minor issue for this study since we focused our in vitro experiments exclusively on neurons and we confirmed in vivo chemogenetic experiments using more specific mouse lines.
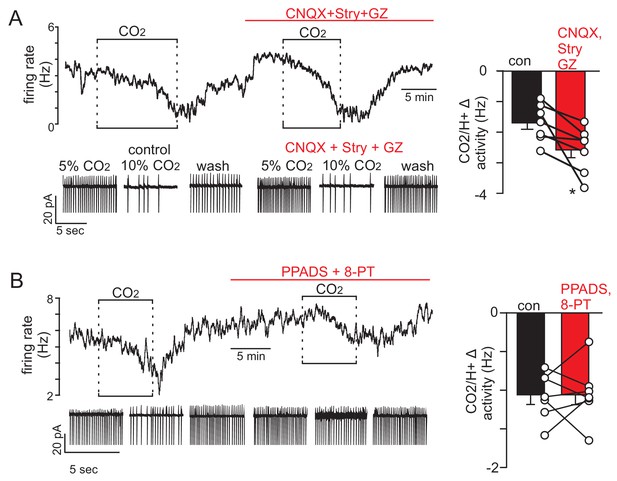
Slc32a1+ parafacial neurons are intrinsically inhibited by CO2.
(A-B) Traces of firing rate and segments of holding current from ventral parafacial inhibitory neurons in slices from Slc32a1Cre::TdT mice show that exposure to 10% CO2 suppressed activity under control conditions and when fast neurotransmission was blocked CNQX (10 μM), gabazine (10 μM) and strychnine (2 μM) (A) or when purinergic signaling was blocked with PPADS (100 μM) and 8-PT (10 µM). Right, summary data plotted as CO2/H+-induced change in activity under control conditions and in each blocker cocktail. Not the combination of CNQX, gabazine, and strychnine actually augmented the inhibitory effects of CO2/H+. Paired t-test, *p<0.05.
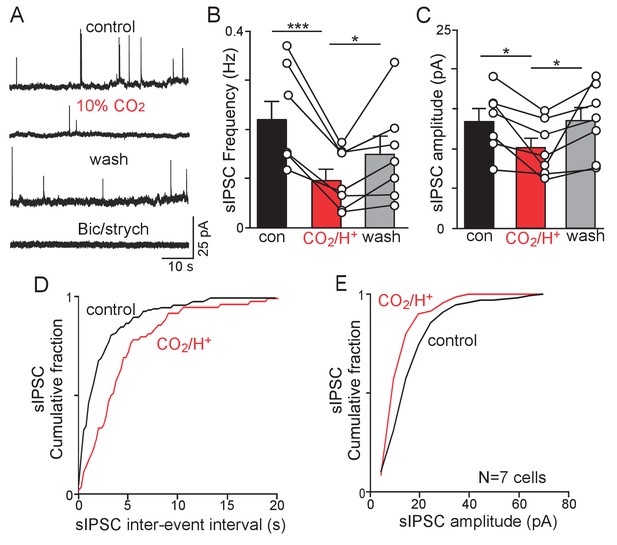
CO2/H+-dependent suppression of inhibitory synaptic input to RTN chemoreceptors.
(A) Traces of holding current (Ihold = 0 mV) from an RTN chemoreceptor in a slice from a Slc32a1Cre::TdT mouse shows sIPSC events under control conditions and during exposure to 10% CO2 or bicuculline (10 µM) and strychnine (2 µM). (B–C) Summary data (N = 7) show the average effect of CO2/H+ on sIPSC freq (B) and amplitude (C). (D–E) Effect of CO2/H+ on sIPSC frequency and amplitude are also reflected in cumulative distribution plots of sIPSC inter-event interval (D; bin size 250 ms) and amplitude (E; bin size 5 pA). Data was analyzed by one-way RM ANOVA followed by Tukey multiple comparison test. *p<0.05, **p<0.01 ***p<0.001.
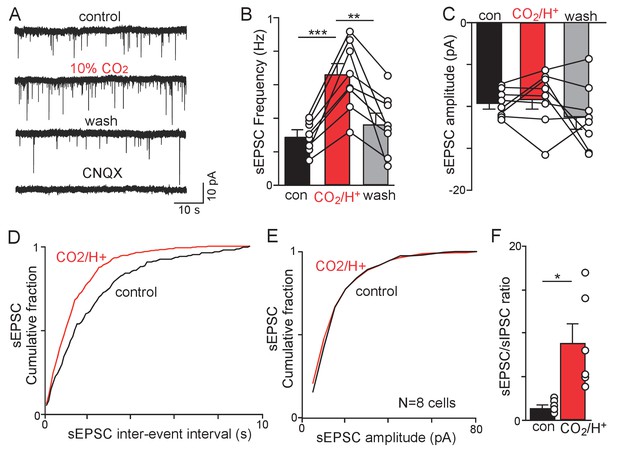
CO2/H+-dependent facilitation of excitatory glutamatergic input to RTN chemoreceptors.
(A) Traces of holding current (Ihold = −60 mV) from a chemosensitive RTN neuron in a slice from a Slc32a1Cre::TdT mouse shows sEPSC events under control conditions and during exposure to 10% CO2 or CNQX (10 µM). (B-C) summary data (N = 8) show the average effect of CO2/H+ on sEPSC freq (B) and amplitude (C). Note that two outlier data points were excluded from analysis. (D–E) Effect (or lack thereof) of CO2/H+ on sEPSC frequency and amplitude are also shown in cumulative distribution plots of sIPSC inter-event interval (D; bin size 100 ms) and amplitude (E; bin size 5 pA). (F) CO2/H+-induced suppression of sIPSC frequency in conjunction with increased sEPSC frequency resulted in enhancement of the sEPSC/sIPSC ratio. *p<0.05, **p<0.01 ***p<0.001.
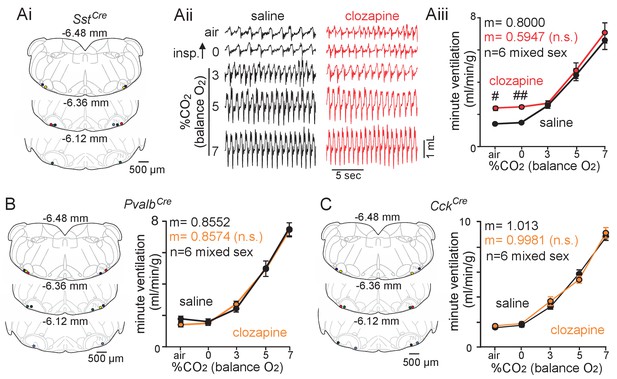
Chemogenetic suppression of Sst+ but not Pvalb+ or Cck+ parafacial neurons increased baseline breathing.
(Ai) Computer-assisted plots show centers of bilateral AAV2-hSyn-DIO-hM4D-mCherry injections in SstCre animals. The number above each section indicates the relative position of each slice behind bregma (Paxinos and Franklin, 2013). (Aii) Traces of respiratory activity from SstCre mice that received bilateral parafacial injections of AAV2-hSyn-DIO-hM4D-mCherry following systemic (I.P.) injection of clozapine (1 mg/kg) or saline (control). Aiii, summary data (n = 6) shows effects of chemogenetic suppression Sst+ parafacial neurons with clozapine on minute ventilation under room air conditions and in 0–7% CO2 (balance O2). (B–C) Left side shows computer-assisted plots of AAV2-hSyn-DIO-hM4D-mCherry injection centers in PvalbCre (B) and CckCre (C) mice, and summary data to the right shows effects of chemogenetic suppression of parafacial Pvalb+ (B) and Cck+ (C) neurons on minute ventilation under room air conditions and in 0–7% CO2 (balance O2). N = 6 for each genotype (mixed sex). Slopes between 0% and 7% CO2 were compared by analysis of covariance (ANCOVA). #, different between genotypes (two-way ANOVA with Tukey’s multiple comparison test; one symbol p<0.05, two symbols p<0.01).
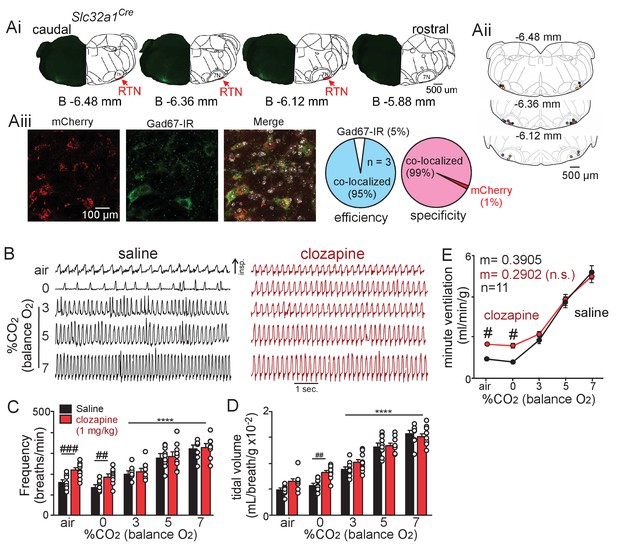
Chemogenetic suppression of all ventral parafacial neurons increased baseline breathing.
(Ai) Confocal images of coronal sections and corresponding brainstem schematic show the placement a typical parafacial injection and its diffusion of ~100 µm along the rostral-caudal axis. (Aii) Computer-assisted plots show the center of all bilateral AAV2-hSyn-DIO-hM4D-mCherry injections (n = 11 animals). Approximate millimeters behind bregma is indicated by numbers next to each section. (Aiii) Left, examples of mCherry and Gad67-immunoreactivity (IR) and summary data to the right confirm both specificity and efficiency of this viral targeting approach. (B) Traces of respiratory activity from Slc32a1Cre mice that received bilateral parafacial injections of AAV2-hSyn-DIO-hM4D-mCherry following systemic (I.P.) injection of clozapine (1 mg/kg) or saline (control). (C–E) Summary data shows effects of chemogenetic suppression of parafacial inhibitory neurons with clozapine on respiratory frequency (C), tidal volume (D) and minute ventilation (E) under room air conditions and in 0–7% CO2 (balance O2). *, different from 0% CO2 as assessed by Tukey’s post-hoc multiple comparison test. ####, different between genotypes (two-way ANOVA with Tukey’s multiple comparison test, p<0.0001).
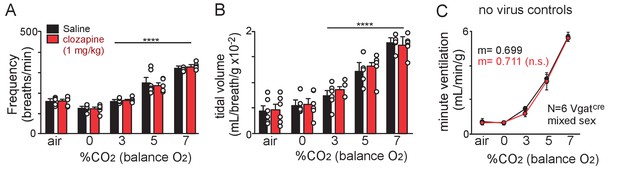
Clozapine alone minimally effects baseline breathing or the chemoreflex.
(A-C) Summary data shows that injection of clozapine (1 mg/kg; I.P) into Slc32a1Cre mice that did not receive AAV injections had negligible effects on respiratory frequency (A), tidal volume (B), and minute ventilation (C) under room air conditions or in 0–7% CO2 (balance O2). These results confirm that the concentration of clozapine used here minimally effects breathing in naïve mice. ****, different from 0% CO2 as assessed by Tukey’s post-hoc multiple comparison test, p<0.0001.
Tables
Reagent type (species) or resource | Designation | Source or reference | Identifiers | Additional information |
---|---|---|---|---|
Strain, strain background (M. musculus, Vgat-iris-Cre, mixed 129/SvJ and C57BL6/J background) | Slc32a1tm2(cre)Lowl/J | Jackson Laboratories | RRID:IMSR_JAX:016962 | |
Strain, strain background (TdTomato reporter Ai14, C57BL6/J background) | B6.Cg-Gt(ROSA)26Sortm9(CAG-tdTomato)Hze/J | Jackson Laboratories | RRID:IMSR_JAX:007909 | |
Strain, strain background (Phox2b Cre, C57Bl6/J background) | B6(Cg)-Tg(Phox2b-cre)3Jke/J | Jackson Laboratories | RRID:IMSR_JAX:016223 | |
Strain, strain background (Sst Cre, C57Bl6/J background) | Ssttm2.1(cre)Zjh/J | Jackson Laboratories | RRID:IMSR_JAX:013044 | |
Strain, strain background (Pvalb Cre, C57Bl6/J background) | Pvalbtm1(cre)Arbr/J | Jackson Laboratories | RRID:IMSR_JAX:017320 | |
Strain, strain background (Cck-IRES Cre, C57Bl6/J background) | Ccktm1.1(cre)Zjh/J | Jackson Laboratories | RRID:IMSR_JAX:012706 | |
Transfected construct (M. musculus) | AAV2-hSyn-DIO-hM4D(Gi)-mCherry | PMID:21364278 | RRID:Addgene_44362 | |
Antibody | (goat polyclonal) anti-mouse Phox2b antibody | R and D Systems | RRID:AB_10889846 | (1:100 dilution) |
Antibody | (rat monoclonal) anti-mouse GAD67 antibody,close 1G10.2 | Millipore Sigma | RRID:AB_2278725 | (1:250 dilution) |
Antibody | (mouse monoclonal) anti-mouse Parvalbumin antibody | Millipore Sigma | RRID:AB_2174013 | (1:250 dilution) |
Antibody | (rabbit polyclonal) anti-mouse CCK-AR antibody | R and D Systems | RRID:AB_2275486 | (1:250 dilution) |
Antibody | (mouse monoclonal) anti-Somatostatin antibody | Santa Cruz Biotechnology | RRID:AB_831726 | (1:200 dilution) |
Antibody | (rabbit polyclonal) anti-lucifer yellow antibody | ThermoFisher | RRID:AB_2536190 | (1:500 dilution) |
Antibody | (donkey polyclonal) anti-rabbit AlexaFluor 488 | Jackson Immunoresearch | 711-545-152 | (1:500 dilution) |
Antibody | (donkey polyclonal) anti-mouse AlexaFluor 647 | Jackson Immunoresearch | 715-605-150 | (1:500 dilution) |
Sequence-based reagent | RNAScope Probe-Gad1 | ACDBio | 400951-C3 | 1:50 |
Sequence-based reagent | RNAScope Probe-Slc6a5 | ACDBio | 425351-C2 | 1:50 |
Sequence-based reagent | RNAScope Probe-Slc32a1 | ACDBio | 319191 | 50:1 |
Commerical assay or kit | RNAscope Fresh Frozen Multiplex Fluorescent Kit | ACDBio | 320851 | |
Commerical assay or kit | Chromium Single Cell 3’ Reagent Kit | 10X Genomics | PN 120237 | Version 2 |
Chemical Compound, drug | Lucifer Yellow | Sigma | L0259 | 0.2% |
Chemical compound, drug | Clozapine | Sigma | 1142107 | 1 mg/mL |
Chemical compound, drug | Strychnine | Sigma | S0532 | 2 µM |
Chemical compound, drug | Gabazine | Tocris | 1262 | 10 µM |
Chemical compound, drug | CNQX | Tocris | 0190 | 10 µM |
Chemical compound, drug | PPADS | Tocris | 0625 | 100 µM |
Chemical compound, drug | 1,3-dimethyl-8-phenyl-xantine (8-PT) | Sigma | P2278 | 10 µM |
Software, algorithm | Loupe Browser | 10X Genomics | RRID:SCR_018555 | Version 5.0.0 |
Software, algorithm | Ponemah | DSI | RRID:SCR_01701 | Version 5.32 |
Software, algorithm | Spike | Cambridge Electronic Design | RRID:SCR_00903 | Version 5.0 |
Software, algorithm | Prism 7 | GraphPad | RRID:SCR_002798 | Version 7.03 |
Software, algorithm | pCLAMP 10 | Molecular Devices | RRID:SCR_011323 | Version 10 |
Software, algorithm | ImageJ | NIH | RRID:SCR_003070 | Version 2.0.0 |
Software, algorithm | Synaptosoft | Mini Analysis Program | RRID:SCR_002184 |