Tripartite suppression of fission yeast TORC1 signaling by the GATOR1-Sea3 complex, the TSC complex, and Gcn2 kinase
Figures
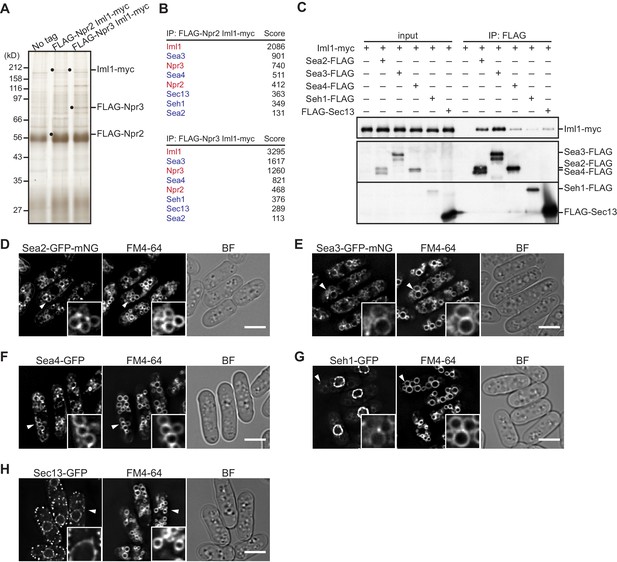
Identification of GATOR2 components as GATOR1-binding proteins.
(A) Affinity purification of the GATOR complex in fission yeast. The GATOR1 complex was purified from cells co-expressing FLAG-Npr2 and Iml1-myc, as well as those co-expressing FLAG-Npr3 and Iml1-myc, by two successive immunoprecipitation steps using anti-FLAG and anti-myc beads. The immunoprecipitates were resolved on SDS–PAGE followed by silver staining. The protein bands corresponding to the tagged Iml1, Npr2, and Npr3 are indicated by black dots. Wild-type cells expressing untagged proteins were used as a control (No tag). (B) Proteins co-purified with the GATOR1 subunits in (A) were subjected to mass spectrometric analysis. For each protein listed in the tables, two or more peptides were identified. The sum of peptide scores that exceed the 95% confidence level (p<0.05) is also shown for each of the identified proteins. GATOR1 and GATOR2 components are indicated in red and blue, respectively. (C) Physical interactions between Iml1 and the GATOR2 components were confirmed. Crude lysates (input) were prepared from the iml1:myc strain expressing one of the GATOR2 components tagged with FLAG for immunoprecipitation. The anti-FLAG immunoprecipitates (IP: FLAG) were analyzed by immunoblotting. (D–H) The cellular localization of GATOR2 proteins. The indicated fluorescence-tagged strains were grown in Edinburgh minimal medium (EMM) at 30°C for microscopy. Vacuolar membranes were visualized by the fluorescent dye FM4-64. Z-axial images were collected, and mid-section images after deconvolution are shown. Insets show the magnified views of the marked areas. BF, bright-field image. Bars, 5 μm.
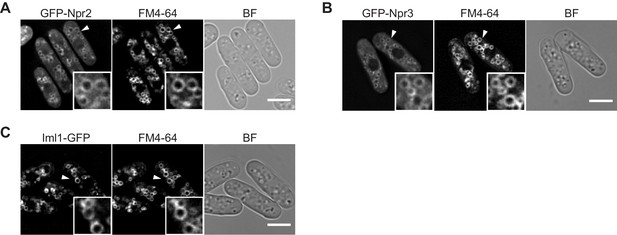
The GATOR1 subunits Iml1, Npr2, and Npr3 are localized to vacuolar membranes.
(A–C) The indicated GFP-tagged strains were grown in EMM at 30°C for microscopy. Vacuolar membranes were visualized by the fluorescent dye FM4-64. Z-axial images were collected, and mid-section images after deconvolution are shown. Insets show the magnified views of the marked areas. BF, bright-field image. Bars, 5 μm.
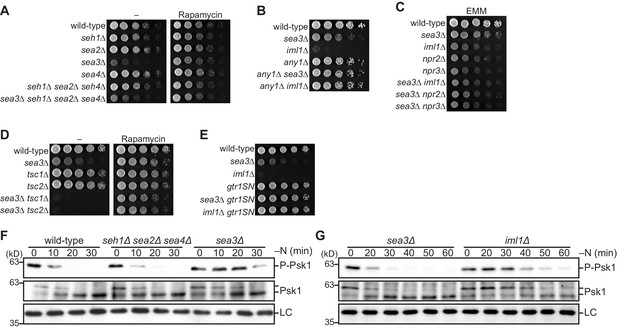
Sea3-GATOR1 attenuates TORC1 signaling via the Gtr1–Gtr2 Rag GTPases.
(A–E) Wild-type and the indicated mutant strains were grown in liquid EMM medium and their serial dilutions were spotted onto the plates. (A) Sea3 is required for normal cell growth. The indicated strains were spotted onto solid YES medium with (Rapamycin) or without (–) 100 ng/ml rapamycin. (B) Loss of Any1 rescues the growth defect of the sea3∆ and iml1∆ mutants. The indicated strains were spotted onto solid YES medium. (C) Sea3 and the GATOR1 complex function in the same pathway. Growth of the indicated strains was compared on solid EMM medium. (D) Sea3 attenuates TORC1 in parallel with the TSC complex. The indicated strains were spotted onto solid YES medium with (Rapamycin) or without (–) 100 ng/ml rapamycin. The growth defect of cells lacking Sea3 was accentuated in the absence of the TSC complex. (E) Genetic interactions between Sea3/Iml1 and Gtr1–Gtr2. The indicated strains were spotted onto solid YES medium. The expression of GDP-bound Gtr1 (gtr1SN) rescues the growth defect of the sea3∆ mutant. (F, G) Inactivation of TORC1 upon nitrogen starvation is delayed in cells lacking Sea3. TORC1 activity was monitored by detecting the TORC1-dependent phosphorylation of Psk1 (P-Psk1). The indicated strains were grown in EMM at 30°C, followed by shifting to the same medium without nitrogen source (–N). Cells were collected at the indicated time points after shifting to nitrogen starvation medium for immunoblotting. The samples were also probed with the anti-Psk1 antibody (Psk1), as well as the anti-Spc1 MAPK antibody for a loading control (LC).
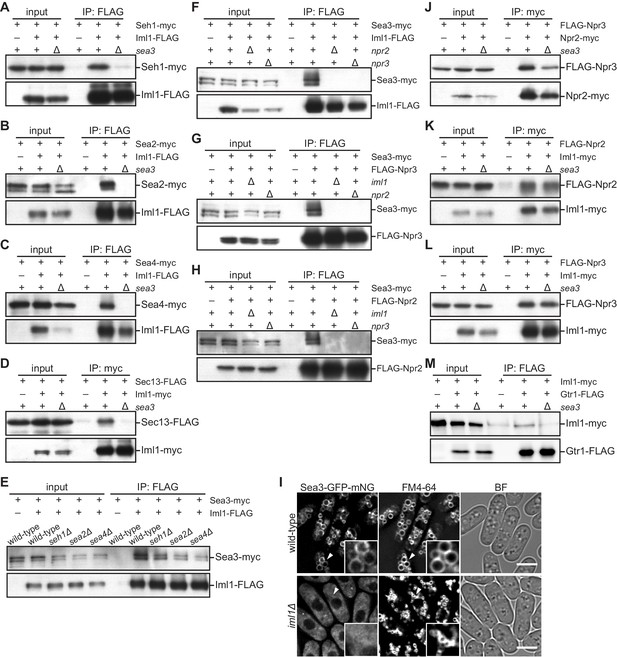
Sea3 connects the GATOR2 components to GATOR1.
(A–C) Sea3 mediates the physical interaction of other GATOR2 proteins with GATOR1. Crude lysates (input) were prepared from cells expressing one of the GATOR2 components tagged with myc, with (+) or without (–) co-expression of Iml1-FLAG, in the presence (+) or absence (∆) of Sea3. The anti-FLAG immunoprecipitates (IP: FLAG) were analyzed by immunoblotting. (D) Sea3 mediates the physical interaction of Sec13 with GATOR1. Crude lysates (input) were prepared from sec13:FLAG cells, with (+) or without (–) co-expression of Iml1-myc, in the presence (+) or absence (∆) of Sea3. The anti-myc immunoprecipitates (IP: myc) were analyzed by immunoblotting. (E) The interaction of Sea3 with GATOR1 does not require other GATOR2 components. Crude lysates (input) were prepared from sea3:myc cells, with (+) or without (–) co-expression of Iml1-FLAG, in wild-type and the GATOR2 mutant backgrounds. The anti-FLAG immunoprecipitates (IP: FLAG) were analyzed by immunoblotting. (F–H) The binding of Sea3 to GATOR1 requires an intact GATOR1 complex. Crude lysates (input) were prepared from sea3:myc cells, with (+) or without (–) co-expression of Iml1-FLAG (F), FLAG-Npr3 (G), or FLAG-Npr2 (H), in the presence (+) or absence (∆) of the GATOR1 components (iml1, npr2, or npr3). The anti-FLAG immunoprecipitates (IP: FLAG) were analyzed by immunoblotting. (I) The vacuolar localization of Sea3 is impaired in cells lacking Iml1. The wild-type and iml1∆ strains expressing Sea3 tagged with GFP-mNeonGreen were grown in EMM at 30°C for microscopy. The vacuolar membranes were visualized by the fluorescent dye FM4-64. Z-axial images were collected and mid-section images after deconvolution are shown. Insets show the magnified views of the marked areas. BF, bright-field image. Bars, 5 μm. (J–L) Sea3 is dispensable for the integrity of the GATOR1 complex. Crude lysates (input) were prepared from FLAG:npr3 cells with (+) or without (–) co-expression of Npr2-myc (J) or Iml1-myc (L), as well as from FLAG:npr2 cells with (+) or without (–) co-expression of Iml1-myc (K). The anti-myc immunoprecipitates (IP: myc) were compared between the presence (+) or absence (∆) of Sea3. (M) Loss of Sea3 reduces the interaction of Iml1 with Gtr1. Crude lysates (input) were prepared from iml1:myc cells with (+) or without (–) co-expression of Gtr1-FLAG. The anti-FLAG immunoprecipitates (IP: FLAG) were compared between the presence (+) or absence (∆) of Sea3.
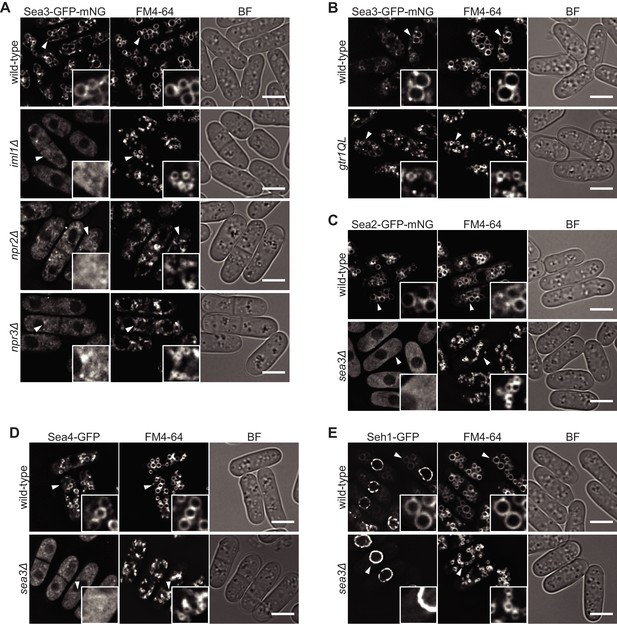
The GATOR2 components are tethered to the vacuolar surface by GATOR1 complex through Sea3.
(A–E) Wild-type and the indicated mutant cells expressing fluorescence-tagged Sea2, Sea4, Seh1, or Sea3 were grown in EMM at 30°C for microscopy. Vacuolar membranes were visualized by the fluorescent dye FM4-64. Z-axial images were collected, and mid-section images after deconvolution are shown. Insets show the magnified views of the marked areas. An intact GATOR1-complex is required for the localization of Sea3 on vacuoles (A). The gtr1QL mutant carried the GTP-locked form of Gtr1 (B). The Sea3 localization defect in cells lacking intact GATOR1 is not due to deregulated TORC1 signaling because hyper-activation of TORC1 in the absence of Gtr1GDP did not cause the defect (B). Vacuolar localization of Sea2, Sea4, and Seh1 is dependent on Sea3 (C–E). BF, bright-field image. Bars, 5 μm.
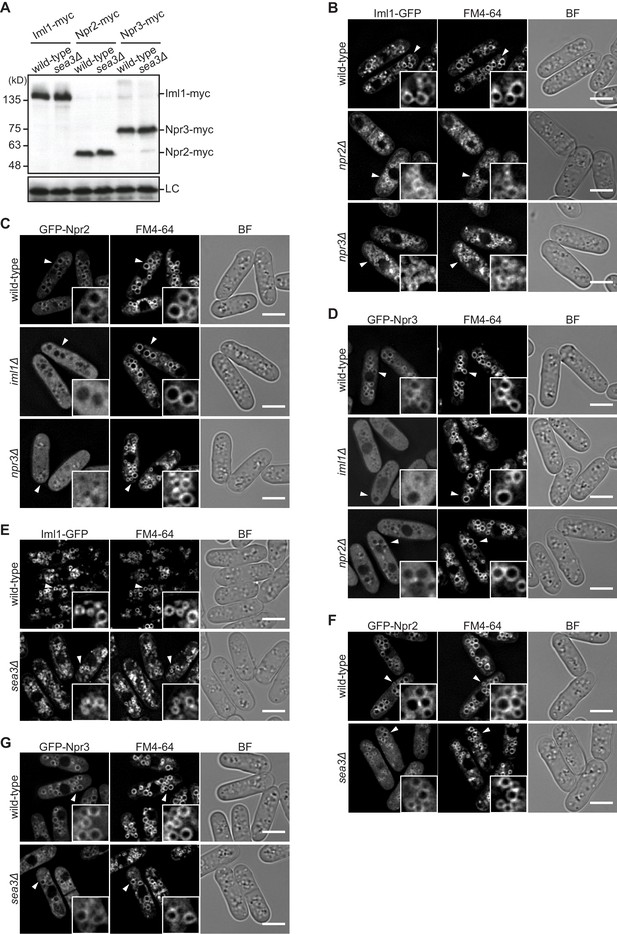
Sea3 is dispensable for the stability and vacuolar localization of GATOR1.
(A) The protein level of the GATOR1 components is not affected by the absence of Sea3. Wild-type and sea3∆ cells expressing myc-tagged Iml1, Npr2, or Npr3 were grown in EMM at 30°C for immunoblotting. Samples were probed with the anti-myc antibody, as well as the anti-Spc1 MAPK antibody for a loading control (LC). (B) Vacuolar localization of Iml1 is independent of Npr2 and Npr3. The indicated cells expressing Iml1-GFP were grown in EMM at 30°C for microscopy. (C, D) Vacuolar localization of Npr2 and Npr3 requires the other GATOR1 components. Wild-type and the indicated mutant cells expressing GFP-tagged Npr2 or Npr3 were grown in EMM at 30°C for microscopy. Two hundred nanograms per milliliter rapamycin was added to the medium to suppress hyper-activation of TORC1 in the absence of intact GATOR1, which causes abnormal vacuolar morphology disturbing the observation. (E) Vacuolar localization of Iml1 is independent of Sea3. The indicated cells expressing GFP-tagged Iml1 were grown in EMM at 30°C for microscopy. (F, G) Vacuolar localization of Npr2 and Npr3 is independent of Sea3. The indicated cells expressing GFP-tagged Npr2 or Npr3 were grown in EMM at 30°C for microscopy. Two hundred nanograms per milliliter rapamycin was added to the medium as in (C, D). For microscopic analysis, vacuolar membranes were visualized by the fluorescent dye FM4-64. Z-axial images were collected and mid-section images after deconvolution are shown. Insets show the magnified views of the marked areas. BF, bright-field image. Bars, 5 μm.
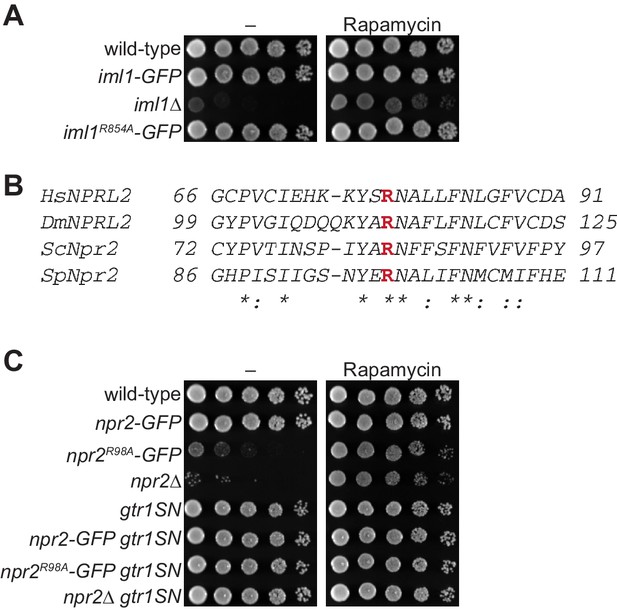
A conserved arginine in Npr2 is required for the GATOR1 function.
(A, C) The indicated strains were spotted onto solid YES medium with (Rapamycin) or without (–) 100 ng/ml rapamycin. Normal cell growth requires Arg98 of Npr2, but not Arg854 of Iml1. The expression of GDP-bound Gtr1 (gtr1SN) rescues the growth defect of the npr2R98A and npr2∆ mutants. (B) Sequence alignment of the NPRL2 orthologs is shown. Conserved arginine residues are indicated in red. Hs, Homo sapiens; Dm, Drosophila melanogaster; Sc, Saccharomyces cerevisiae; Sp, Schizosaccharomyces pombe.
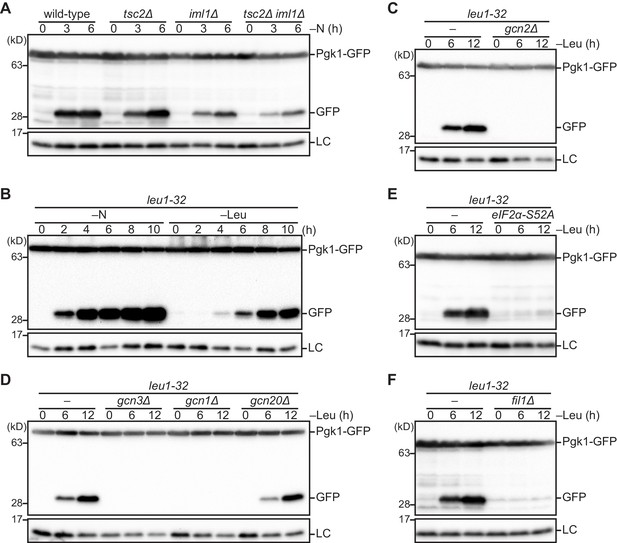
The Gcn2 signaling pathway regulates autophagy induction upon amino acid starvation.
(A) Nitrogen starvation-induced autophagy is reduced but not completely prevented in cells defective in the TSC complex (tsc2∆), GATOR1 (iml1∆), or both (tsc2∆ iml1∆). Autophagy was monitored by detecting the GFP moiety processed from Pgk1-GFP. The indicated strains were grown in EMM at 30°C, followed by shifting to the same medium without nitrogen source (–N). Cells were collected at the indicated time points after shifting to nitrogen starvation medium for immunoblotting against GFP. The samples were also probed with the anti-histone H3 antibody for a loading control (LC). (B) Autophagy is induced by leucine starvation or nitrogen starvation. Cells auxotrophic for leucine (leu1-32) were grown in EMM supplemented with 1.7 mM leucine at 30°C and shifted to the same medium without nitrogen source (–N) or EMM without the supplement (–Leu). Autophagy was monitored by immunoblotting as in (A). (C–F) Leucine starvation-induced autophagy requires the Gcn2 signaling pathway. Wild-type and the indicated mutant cells auxotrophic for leucine (leu1-32) were collected at the indicated time points after shifting from EMM supplemented with 1.7 mM leucine to EMM without the supplement (–Leu). Autophagy was monitored by immunoblotting as in (A). The eIF2α-S52A mutant (E) carries the Ser52 to Ala52 mutation in the tif211+ gene coding eIF2α.
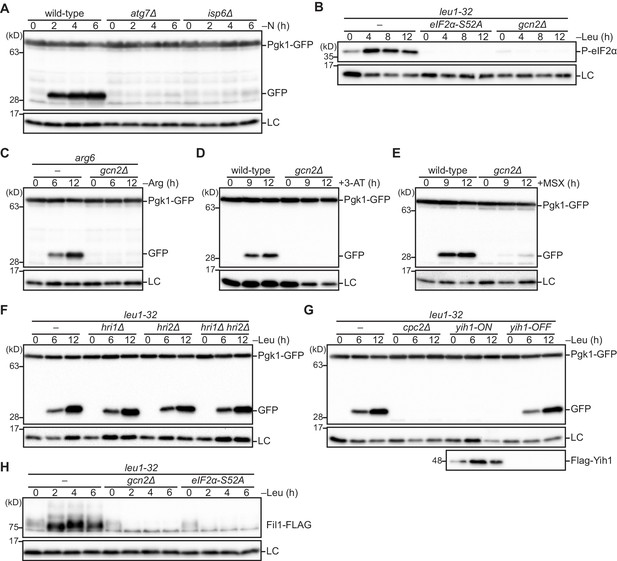
The Gcn2 signaling pathway is essential for autophagy induction upon amino acid starvation.
(A) The processing of GFP-Pgk1 depends on autophagy. Wild-type and the indicated mutant strains expressing GFP-tagged Pgk1 were grown in EMM at 30°C, followed by shifting to the same medium without nitrogen source (–N). Cells were collected at the indicated time points after shifting to nitrogen starvation medium for immunoblotting against GFP. The samples were also probed with the anti-histone H3 antibody for a loading control (LC). (B) Leucine starvation brings about phosphorylation of eIF2α at S52 in a Gcn2-dependent manner. Wild-type and the indicated mutant cells auxotrophic for leucine (leu1-32) were grown in EMM supplemented with 1.7 mM leucine at 30°C, and shifted to EMM without the supplement (–Leu). Cells were collected at the indicated time points after shifting to leucine starvation medium for immunoblotting. The samples were probed with the anti-phospho-eIF2α and anti-histone H3 (LC) antibodies. (C) Autophagy is induced upon arginine starvation. Wild-type and gcn2∆ cells auxotrophic for arginine (arg6) were grown in EMM supplemented with 1.3 mM arginine at 30°C, and shifted to EMM without the supplement (–Arg). Cells were collected at the indicated time points after shifting to arginine starvation medium and autophagy was monitored as in (A). (D, E) Gcn2 facilitates autophagy induction upon histidine or glutamine starvation. Wild-type and gcn2∆ cells grown in EMM at 30°C were treated with 25 mM histidine biosynthesis inhibitor 3-amino-1,2,4-triazole (3-AT) (D) or 10 mM glutamine synthetase inhibitor methionine sulfoximine (MSX) (E). Autophagy was monitored as in (A). (F) The eIF2α kinases other than Gcn2, such as Hri1 and Hri2, are not involved in leucine starvation-induced autophagy. Autophagy was induced as in (B) and monitored as in (A). (G) Loss of Cpc2 or over-expression of Yih1 prevents leucine starvation-induced autophagy. Autophagy was induced by leucine starvation as in (B) and monitored by immunoblotting as in (A). To over-express Yih1, the gene coding FLAG-tagged Yih1 was fused to the thiamine-repressible nmt1 promoter. Yih1 expression was controlled with (yih1-OFF) or without (yih1-ON) supplementation of 15 μM thiamine in the medium. (H) Fil1 expression is up-regulated in response to leucine starvation dependently on Gcn2 and eIF2α phosphorylation. Leucine starvation was induced as in (B). The samples were probed with the anti-FLAG and anti-histone H3 (LC) antibodies.
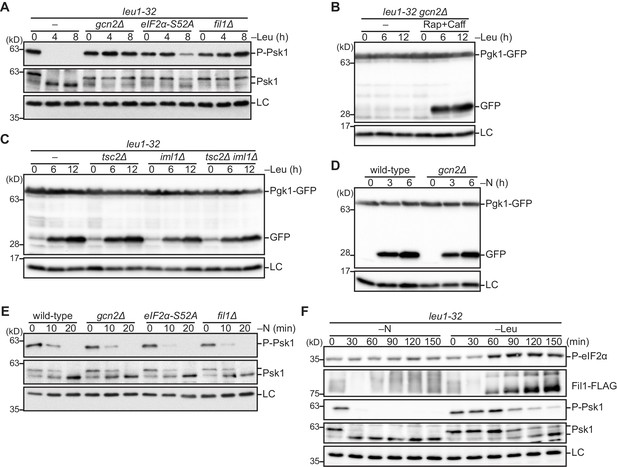
The Gcn2 signaling pathway facilitates amino acid starvation-induced autophagy by attenuating TORC1 signaling.
(A) Inactivation of TORC1 upon leucine starvation is impaired in cells lacking Gcn2 or Fil1 and the mutant carrying the non-phosphorylated form of eIF2α. TORC1 activity was monitored by detecting the TORC1-dependent phosphorylation of Psk1 (P-Psk1). The indicated strains auxotrophic for leucine (leu1-32) were grown in EMM supplemented with 1.7 mM leucine at 30°C and shifted to EMM without the supplement (–Leu). Cells were collected at the indicated time points after shifting to leucine starvation medium for immunoblotting. The samples were also probed with the anti-Psk1 antibody (Psk1), as well as the anti-Spc1 MAPK antibody for a loading control (LC). (B) Inactivation of TORC1 rescues the autophagy defect of the gcn2∆ mutant. Autophagy was monitored by detecting the GFP moiety processed from Pgk1-GFP. The gcn2∆ mutant auxotrophic for leucine (leu1–32) were grown in EMM supplemented with 1.7 mM leucine at 30°C and shifted to EMM without the supplement (–Leu) with (Rap+Caff) or without (–) addition of 200 nM rapamycin and 10 mM caffeine. Cells were collected at the indicated time points after shifting to leucine starvation medium and subjected to immunoblotting. The samples were also probed with the anti-histone H3 antibody for a loading control (LC). (C) The TSC complex and GATOR1 are dispensable for the induction of autophagy upon amino acid starvation. The indicated strains auxotrophic for leucine (leu1–32) were subjected to leucine starvation and immunoblotting as in (B). (D) Gcn2 is not essential for the induction of autophagy upon nitrogen starvation. Wild-type and gcn2∆ cells were grown in EMM at 30°C, followed by shifting to the same medium without nitrogen source (–N). Cells were collected and subjected to immunoblotting as in (B). (E) Inactivation of TORC1 upon nitrogen starvation is independent of the Gcn2 signaling pathway. The indicated strains grown in EMM at 30°C were shifted to the same medium without nitrogen source (–N). Immunoblotting was carried out as in (A). (F) Phosphorylation of eIF2α and up-regulation of Fil1 are induced by leucine starvation to a higher extent than nitrogen starvation. Cells auxotrophic for leucine (leu1–32) were grown in EMM supplemented with 1.7 mM leucine at 30°C and shifted to the same medium without nitrogen source (–N) or EMM without the supplement (–Leu). Immunoblotting was carried out as in (A). The samples were also probed with the anti-phospho-eIF2α and anti-FLAG antibodies.
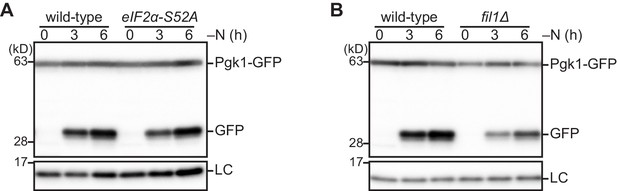
The Gcn2 signaling pathway is not necessary for autophagy induction upon nitrogen starvation.
(A, B) Wild-type and the indicated mutant cells expressing Pgk1-GFP were grown in EMM at 30°C and shifted to the same medium without nitrogen source (–N). Cells were collected at the indicated time points after shifting to nitrogen starvation medium. Autophagy was monitored by immunoblotting detection of the Pgk1-GFP processing. The samples were also probed with the anti-histone H3 antibody for a loading control (LC).
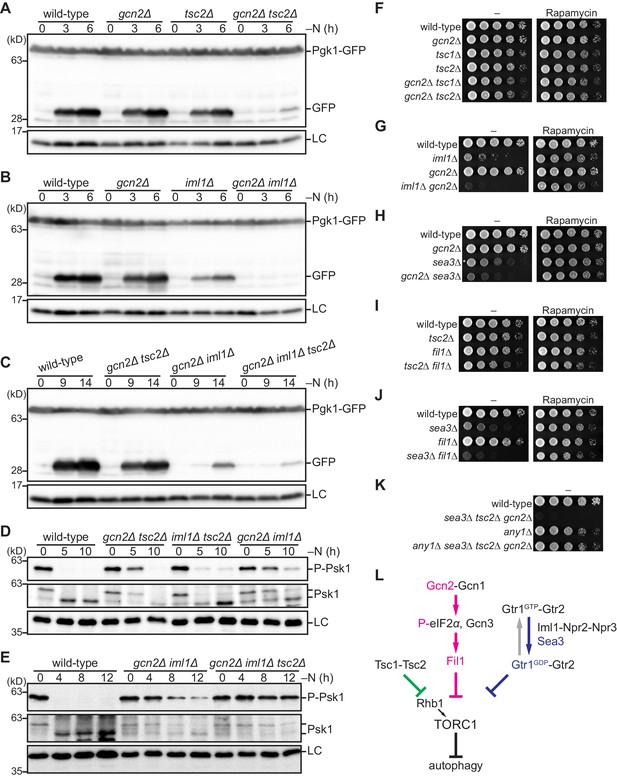
The Gcn2 signaling pathway negatively regulates TORC1 upon nitrogen starvation in the absence of the TSC complex and GATOR1.
(A–C) Gcn2, Tsc2, and Iml1 promote autophagy induction in parallel upon nitrogen starvation. Autophagy was monitored by detecting the GFP moiety processed from Pgk1-GFP. The indicated strains were grown in EMM at 30°C, followed by nitrogen starvation (–N). Cells were collected at the indicated time points after shifting to nitrogen starvation medium for immunoblotting against GFP. The samples were also probed with the anti-histone H3 antibody for a loading control (LC). (D, E) Gcn2, Tsc2, and Iml1 attenuate TORC1 in parallel upon nitrogen starvation. TORC1 activity was monitored by detecting the TORC1-dependent phosphorylation of Psk1 (P-Psk1). The indicated strains cultured in EMM at 30°C were shifted to the same medium without nitrogen source (–N) and collected at the indicated time points for immunoblotting. The samples were also probed with the anti-Psk1 antibody (Psk1), as well as the anti-Spc1 MAPK antibody for a loading control (LC). (F–K) Genetic interactions among the Gcn2 signaling pathway, the TSC complex, and GATOR1. The indicated strains were grown in EMM liquid medium and their serial dilutions were spotted onto solid YES medium with (Rapamycin) or without (–) 100 ng/ml rapamycin. (L) Schematic representation of a model for TORC1 regulation in fission yeast by the Gcn2 signaling pathway, the TSC complex, and GATOR1. Sea3 is involved in the GAP function of GATOR1 toward the Gtr1 GTPase. Gcn2 signaling pathway negatively regulates TORC1 in parallel with the TSC complex and GATOR1. TORC1 inactivation upon amino acid starvation is dependent exclusively on the Gcn2 signaling pathway, whereas that upon nitrogen starvation is mediated redundantly by the three pathways.
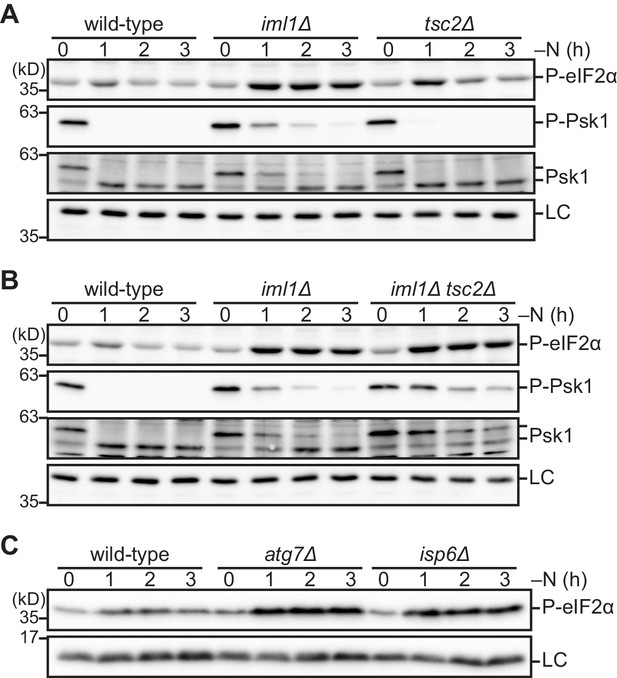
The absence of the GATOR1 and TSC complexes during nitrogen starvation up-regulates phosphorylation of eIF2α.
(A–C) Cells lacking Iml1, Tsc2, or autophagic degradation exhibit highly phosphorylated eIF2α during nitrogen starvation. The indicated strains were grown in EMM at 30°C, followed by shifting to the same medium without nitrogen source (–N). Cells were collected at the indicated time points after shifting to nitrogen starvation medium for immunoblotting. The anti-Spc1 MAPK (A, B) and anti-histone H3 (C) antibodies were used for loading controls (LC).

Gcn2, GATOR1, and the TSC complex redundantly regulate TORC1.
(A, B) The kinetics of autophagy induction in cells expressing Iml1, Gcn2, or Tsc2 as a main TORC1 inhibitor. The indicated strains were grown in EMM at 30°C, followed by shifting to the same medium without nitrogen source (–N). Cells were collected at the indicated time points after shifting to nitrogen starvation medium for immunoblotting. The samples were probed with the anti-GFP and anti-histone H3 (LC) antibodies. (C–H) The kinetics of TORC1 attenuation in cells expressing Iml1, Gcn2, or Tsc2 as a main TORC1 inhibitor. Nitrogen starvation was induced as in (A, B). The samples were probed for the indicated proteins and the Spc1 MAPK (LC).

TORC1 inhibitors are involved in cell survival and sexual differentiation during nitrogen starvation.
(A) Iml1 and Tsc2 are involved in cell survival during long-term starvation. The indicated strains cultured in EMM at 30°C were shifted to the same medium without nitrogen source and incubated at 30°C. At the indicated time points (d, days), cell viability was assessed by spotting serial dilutions of each culture onto solid EMM medium with (Rapamycin) or without (–) 100 ng/ml rapamycin. Rapamycin was added to minimize the effect of the growth defect on the plates. (B) Sexual differentiation is affected by the absence of the TORC1 inhibitors. The indicated homothallic h90 strains were incubated on SSA sporulation plates at 25°C. Mating and sporulation efficiencies were monitored after 48 hr. Mean values with error bars (SD; n = 3) are presented.
-
Figure 6—figure supplement 3—source data 1
Source data for Figure 6—figure supplement 3B.
- https://cdn.elifesciences.org/articles/60969/elife-60969-fig6-figsupp3-data1-v1.xlsx
Tables
Reagent type (species) or resource | Designation | Source or reference | Identifiers | Additional information |
---|---|---|---|---|
Genetic reagent (Schizosaccharomyces pombe) | Fission yeast strains used in this study | See Supplementary file 1 | See Supplementary file 1 | |
Antibody | Anti-phospho-p70 S6K (Thr389) (mouse monoclonal) | Cell Signaling Technology | Cat# 9206; AB_2285392 | (1:4000) |
Antibody | Anti-Psk1 (rabbit polyclonal) | DOI:10.7554/eLife.30880 | N/A | (1:5000) |
Antibody | Anti-Spc1 (rabbit polyclonal) | DOI:10.1128/MCB.23.15.5132-5142.2003 | N/A | (1:10,000) |
Antibody | Anti-FLAG (mouse monoclonal) | Sigma–Aldrich | Cat# F3165; RRID: AB_259529 | (1:4000) |
Antibody | Anti-c-myc (mouse monoclonal) | Covance | Cat# MMS150P; RRID: AB_291322 | (1:4000) |
Antibody | Anti-c-myc (rabbit polyclonal) | Santa Cruz Biotechnology | Cat# sc-789; RRID: AB_631274 | (1:4000) |
Antibody | Anti-GFP (mouse monoclonal) | Takara | Cat# 632380; RRID: AB_10013427 | (1:10,000) |
Antibody | Anti-histone H3 (rabbit polyclonal) | Abcam | Cat# ab1791; RRID: AB_302613 | (1:10,000) |
Antibody | Anti-phospho-eIF2alpha (Ser51) (rabbit polyclonal) | Cell Signaling Technology | Cat# 9721; RRID: AB_330951 | (1:2000) |
Antibody | Anti-DDDDK (rabbit polyclonal) | MBL | Cat# PM020; RRID: AB_591224 | (1:10,000) |
Antibody | Anti-mouse IgG, Peroxidase conjugated (goat polyclonal) | Merck Millipore | Cat# AP124P; RRID: AB_90456 | (1:10,000) |
Antibody | Anti-rabbit IgG, Peroxidase conjugated (goat polyclonal) | Jackson ImmunoResearch | Cat# 111-035-003; RRID: AB_2313567 | (1:10,000) |
Antibody | Anti-rabbit IgG, Peroxidase conjugated (goat polyclonal) | Promega | Cat# W4011; RRID: AB_430833 | (1:2000) |
Antibody | Anti-mouse IgG Peroxidase conjugated (goat polyclonal) | Promega | Cat# W4021; RRID: AB_430834 | (1:2000) |
Chemical compound, drug | R-5000 Rapamycin | LC Laboratories | Cat# 53123-88-9 | |
Chemical compound, drug | Caffeine, anhydrous | Nacalai Tesque | Cat# 06712–42 | |
Chemical compound, drug | Caffeine | Wako | Cat# 033–06791 | |
Chemical compound, drug | SynaptoRed C2 (FM4-64) | Biotium | Cat# 70021 | |
Chemical compound, drug | ViVidFluor Neuro Red (FM4-64) | FUJIFILM | Cat# 222–02121 | |
Chemical compound, drug | L-Methioninesulfoximine | Nacalai Tesque | Cat# 21730–74 | |
Chemical compound, drug | 3-Amino-1,2,4-triazole | Wako | Cat# 014–10911 | |
Commercial assay or kit | SuperSignal West Pico Chemiluminescent Substrate | Thermo Scientific | Cat# 34080 | |
Commercial assay or kit | SilverQuest staining kit | Invitrogen | Cat# LC6070 | |
Commercial assay or kit | EzWestLumi plus | Atto | Cat# WSE-7120 | |
Commercial assay or kit | Clarity Max Western ECL Substrate | Bio-Rad | Cat# 1705062 |
Additional files
-
Supplementary file 1
Fission yeast strains used in this study.
- https://cdn.elifesciences.org/articles/60969/elife-60969-supp1-v1.docx
-
Transparent reporting form
- https://cdn.elifesciences.org/articles/60969/elife-60969-transrepform-v1.docx