Absent phasing of respiratory and locomotor rhythms in running mice
Figures
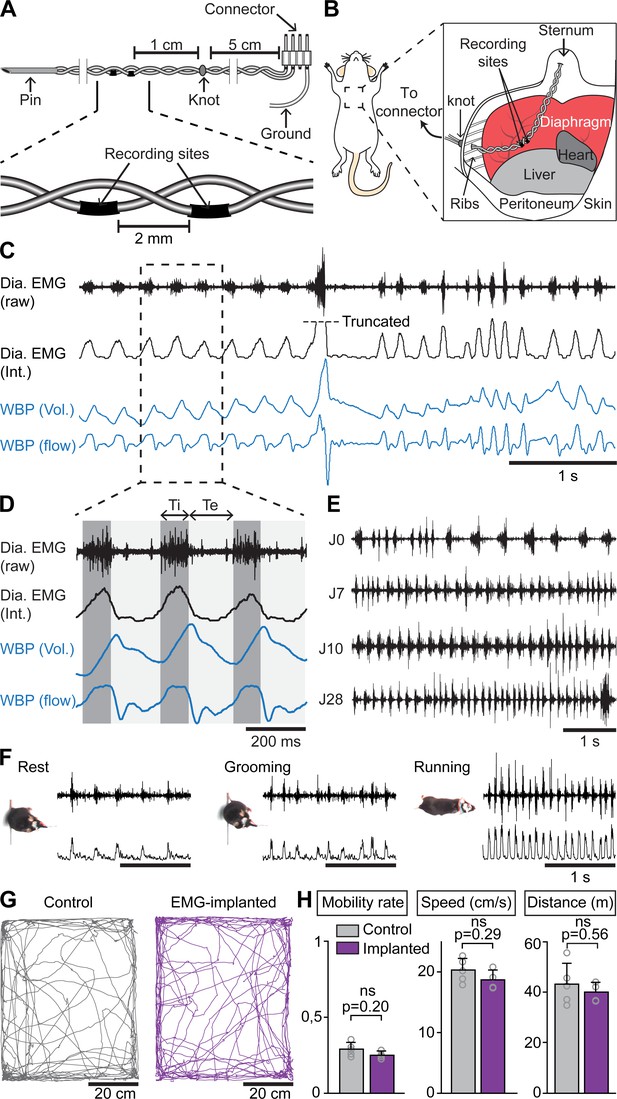
Chronic electromyographic recordings of the diaphragm to monitor inspiration in freely moving mice.
(A, B) Schematics of the EMG recording electrodes and of the diaphragm implantation. (C) Simultaneous diaphragm EMG recordings (raw and integrated traces in black) and whole-body plethysmography (WBP, volume and flow, in blue) showing that the diaphragm neurogram estimates inspiratory flow. (D) Enlarged view of three inspiratory bursts highlighting the following respiratory parameters: inspiratory time (Ti) defined as a bout of diaphragm activity, and expiratory time (Te) as the silent period between bouts. (E) Diaphragm activity recorded on the day of the surgery (J0) and at 7, 10, and 28 days post-surgery (representative of four mice). (F) Raw and integrated diaphragm neurograms in an open field test during rest, grooming and spontaneous running showing preserved recording quality in spite of movements. (G) Locomotor trajectories of one representative non-implanted mouse (gray) and one representative EMG-implanted mouse (purple) for 10 min in the open field. (H) Bar-graphs showing the mean ± SD mobility rate, locomotor speed during mobility and total distance traveled in control (n = 5) and EMG-implanted mice (n = 4). p values in (H) are obtained from Mann-Whitney U tests and considered not significant when p>0.05.
-
Figure 1—source data 1
Locomotor parameters in the open field.
- https://cdn.elifesciences.org/articles/61919/elife-61919-fig1-data1-v1.xlsx
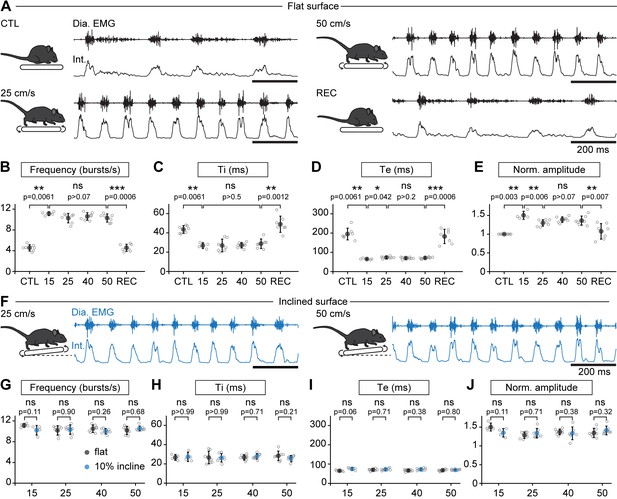
Breathing rate augments during trotting independently of limb velocity or surface inclination.
(A) Diaphragm activity recordings in control condition (CTL), trotting at 25 and 50 cm/s and during recovery after the run (REC). Raw (Dia. EMG) and integrated (Int.) signals are illustrated in each condition. (B–E) Analysis of respiratory parameters in control condition, and during trot at 15, 25, 40, and 50 cm/s, and during recovery: frequency (B), inspiratory (Ti, C) and expiratory (Te, D) times and normalized amplitude (E). Data are mean ± SD from n = 7 mice per condition for all speeds except at 15 cm/s (n = 4) where three animals had unstable running and were discarded. p values indicated are obtained from Mann-Whitney U tests and considered not significant (ns) when p>0.05. (F) Raw (Dia. EMG) and integrated (Int.) diaphragmatic activity recordings during trot at 25 and 50 cm/s on a treadmill with 10% incline. (G–J) Similar analyses as in (B–E) during running on the inclined treadmill (blue) compared to the values on the flat treadmill (black). p values indicated are obtained from Mann-Whitney U tests and considered not significant when p>0.05. See Figure 2—figure supplement 1 for a similar analysis during a 10-min run.
-
Figure 2—source data 1
Respiratory parameters on the flat (B–E) and inclined (G–J) treadmill.
- https://cdn.elifesciences.org/articles/61919/elife-61919-fig2-data1-v1.xlsx
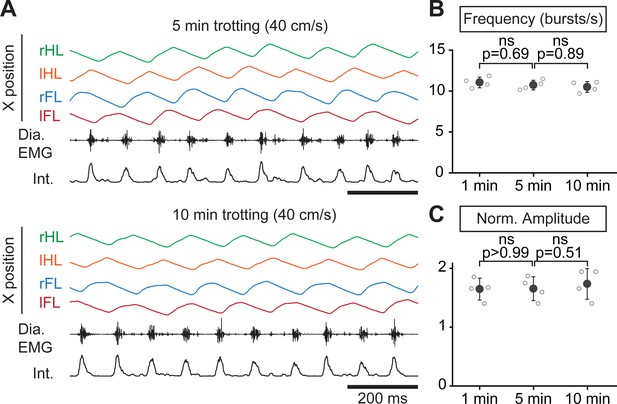
Respiratory changes during a prolonged treadmill running.
(A) Changes in the horizontal (X) axis of the right hindlimb (rHL, green), left hindlimb (lHL, orange), right forelimb (rFL, blue) and left forelimb (lFL, red) over time, as well as raw (Dia. EMG) and integrated (Int.) diaphragmatic neurograms, after 5 and 10 min of continuous running at 40 cm/s on the level treadmill. (B–C) Analyses of respiratory parameters changes after 1, 5, and 10 min of running at 40 cm/s on the level treadmill: frequency (B) and normalized amplitude (C). Note that respiratory parameters were stable throughout the 10-min run. Data are presented as mean ± SD from n = 4 mice and p values indicated are obtained from Mann-Whitney U tests and considered not significant (ns) when p>0.05.
-
Figure 2—figure supplement 1—source data 1
Respiratory parameters (panels B, C).
- https://cdn.elifesciences.org/articles/61919/elife-61919-fig2-figsupp1-data1-v1.xlsx
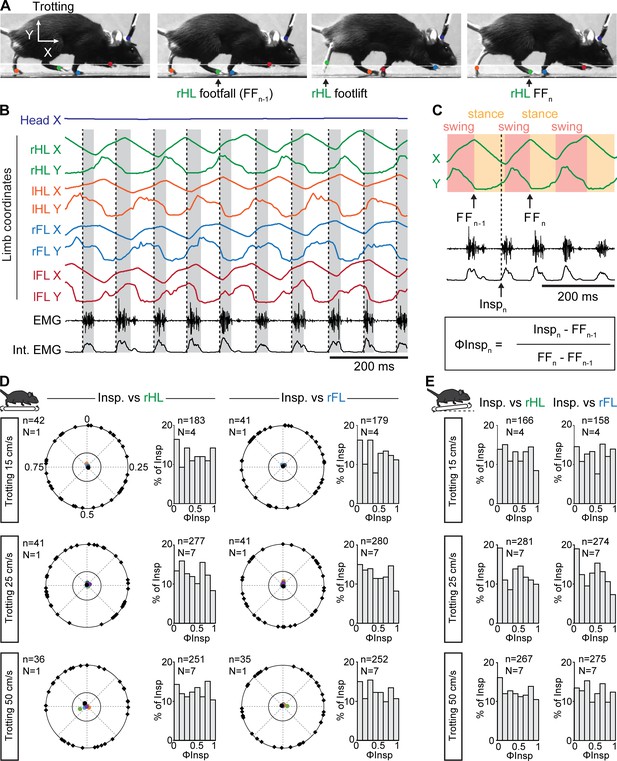
Breaths are not temporally-synchronized to strides during trotting on a treadmill.
(A) Side views of one representative trotting mouse on a flat treadmill. The four limbs and the head were tracked and color-labeled: right hindlimb (rHL, green), left hindlimb (lHL, orange), right forelimb (rFL, blue), left forelimb (lFL, red) and head (dark blue). One complete rHL locomotor cycle is shown, between two consecutive footfalls (FFn-1 and FFn). (B) Horizontal (X) and vertical (Y) coordinates of the tracked limbs as well as raw (EMG) and integrated (Int. EMG) diaphragmatic neurograms, during stable trot. Dotted black lines indicate the onsets of inspiratory bursts and shaded rectangles highlight inspiratory times. (C) Enlarged view of locomotor cycles of the rHL showing the swing and stance phases. The occurrences of inspiratory bursts (Inspn) within the locomotor cycle are expressed as a phase value (ΦInspn) from 0 (FFn-1) to 1 (FFn). (D) Circular plots diagrams showing the phase-relationship between individual inspiratory bursts and the indicated reference limb for one representative animal trotting at 15, 25, and 50 cm/s on a flat treadmill. Black diamonds on the outer circle indicate the phase of n individual inspirations. The black dot indicates the mean orientation vector for that animal and the colored dots indicate the mean orientation vector of 3 (at 15 cm/s) or 6 (at 25 and 50 cm/s) other animals. The positioning of these mean values within the inner circle illustrates the absence of a significantly oriented phase preference (R < 0.3, with R being the concentration of phase values around the mean as defined in Kjaerulff and Kiehn, 1996). Bar-graphs to the right are distribution histograms of the phases of inspiratory bursts and the same reference limb for all n events from N animals. (E) Phase distribution histograms between inspiratory bursts and the indicated reference limb for all n events from N animals running at 15, 25, or 50 cm/s on the inclined treadmill. Note that inspiratory bursts in (D) and (E) are evenly distributed across the entire locomotor cycle in each condition and that distribution histograms do not show a phase-preference. See also Figure 3—figure supplement 1 for a similar analysis during a 10-min run, and Figure 3—figure supplement 2 for cross-correlograms between inspiratory and limb activity.
-
Figure 3—source data 1
Circular plots for the flat treadmill conditions (panel D).
- https://cdn.elifesciences.org/articles/61919/elife-61919-fig3-data1-v1.xlsx
-
Figure 3—source data 2
Distribution histograms for both the flat and inclined treadmill (panels D and E).
- https://cdn.elifesciences.org/articles/61919/elife-61919-fig3-data2-v1.xlsx
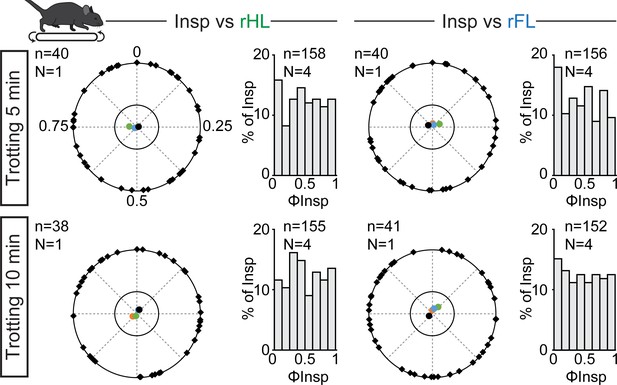
Correlation of breaths to strides during prolonged treadmill running.
Circular plots showing the phase-relationship between inspiratory bursts and the indicated reference limb for one representative animal trotting continuously for 5 (top) or 10 min (bottom). Black diamonds on the outer circle indicate the phase of n individual inspirations. The black dot indicates the mean orientation vector for that animal and the colored dots the mean orientation vector of three other animals (each in a specific color). The positioning of these mean values within the inner circle illustrates the absence of a significantly-oriented phase preference (R < 0.3, with R being the concentration of phase values around the mean as defined in Kjaerulff and Kiehn, 1996). To the right of the circular plots are phase distribution histograms between inspiratory bursts and the same reference limb for all n events from four animals. Note that all inspiratory bursts are evenly distributed across the entire locomotor cycle in each condition.
-
Figure 3—figure supplement 1—source data 1
Circular plots.
- https://cdn.elifesciences.org/articles/61919/elife-61919-fig3-figsupp1-data1-v1.xlsx
-
Figure 3—figure supplement 1—source data 2
Distribution histograms.
- https://cdn.elifesciences.org/articles/61919/elife-61919-fig3-figsupp1-data2-v1.xlsx
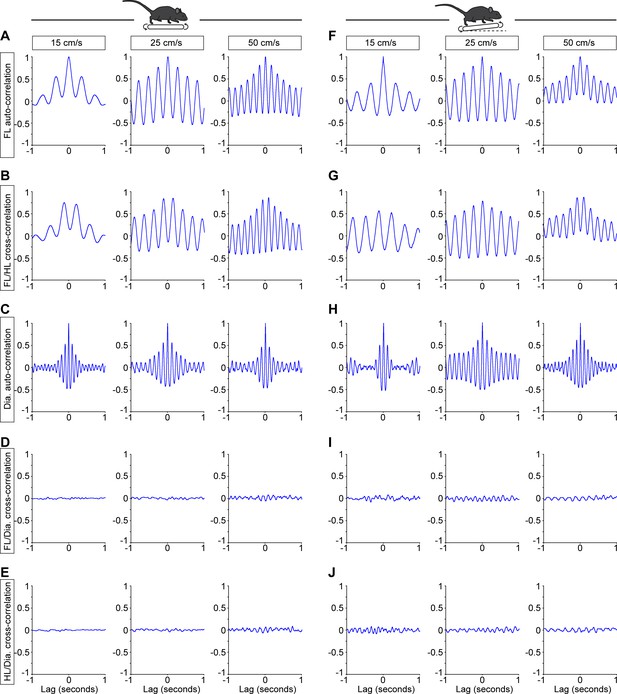
Example cross-correlations of breathing and locomotor rhythms showing no common modulation.
(A–E) Correlograms during stable trotting at 15, 25, and 50 cm/s on a level treadmill for one representative animal, with a lag of 1 s. For the limbs, the horizontal coordinates were used (see Figure 3). For the diaphragm (Dia.) the integrated EMG activity was used. Data are presented as the correlation function estimate (from −1 to 1) at each lag (in s). (A) Auto-correlogram of the right forelimb showing the regularity of the rhythm and the increase step cycle as the treadmill speed increases. (B) Cross-correlogram of the homolateral forelimb and hindlimb showing their alternating activity. (C) Auto-correlogram of the integrated diaphragmatic activity showing the regularity of the rhythm and the comparable frequency at the different treadmill speeds. (D) Cross-correlogram of the forelimb movements and diaphragmatic EMG activity, showing no common modulation at all tested speeds. (E) Cross-correlogram of the hindlimb movements and diaphragmatic EMG activity, showing no common modulation at all tested speeds. (F–I) Similar representations for the same animal trotting on a 10% inclined treadmill, showing no common modulation between locomotor and respiratory rhythms at all tested speeds.
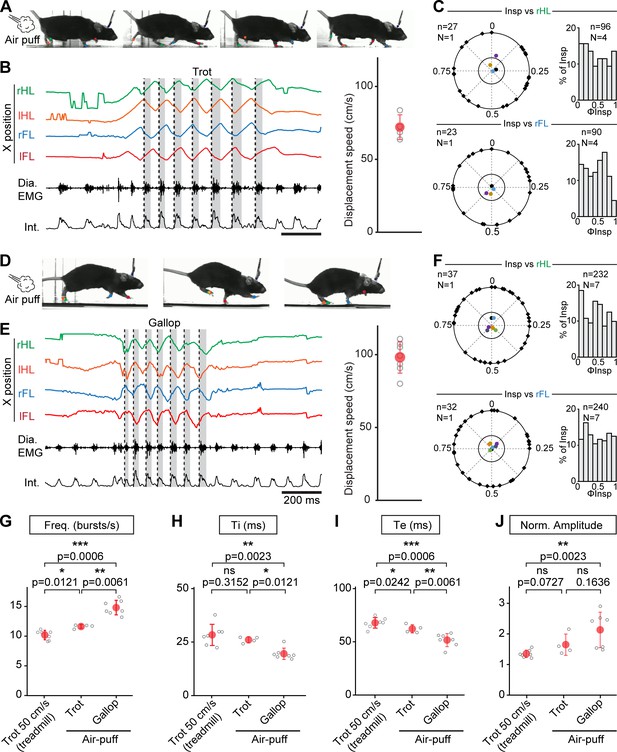
Further augmentation of breathing rate yet without temporal correlation of breaths to strides during escape running, including at gallop.
(A) Side views of one representative mouse during air puff induced trot where right and left hindlimbs (rHL, green; lHL, orange) and forelimbs (rFL, blue; lFL, red) are alternating. (B) Changes in the horizontal (X) axis of the limbs over time as well as raw (Dia. EMG) and integrated (Int.) diaphragmatic neurograms are shown before and during four cycles of trot induced by air puff. Dotted black lines indicate the onsets of inspiratory bursts and shaded areas highlight inspiratory times. On the limb coordinates, rapid deflections before the onset of movement are occasional failures in detecting the paws at rest. The graph to the right shows the average running speed of each animal (gray open circles) and the mean ± SD across animals (in red) during air puff induced trot. (C) Circular plots showing the phase-relationship between n inspiratory bursts and the indicated reference limb for one representative animal (black diamonds on the outer circle). The black dot indicates the mean orientation vector for that animal and the colored dots indicate the mean orientation vector for three other animals. The positioning of these mean values within or close to the inner circle illustrates the absence of a significantly oriented phase preference (R < 0.3, with R being the concentration of phase values around the mean as defined in Kjaerulff and Kiehn, 1996). Bar-graphs to the right are distribution histograms of the phases of inspiratory bursts and the same reference limb for all n events from N = 4 animals. (D–F) Same representation as (A–C) during air puff induced gallop where right and left hindlimbs are now synchronized and right and left forelimbs are alternating. The average displacement speed of the animals at gallop (98 ± 11 cm/s) is significantly higher than at trot (72 ± 8 cm/s, p=0.0121, Mann-Whitney U test). Circular plots and distribution histograms are obtained from N = 7 animals. (G–J) Changes in respiratory frequency (G), inspiratory (Ti, H) and expiratory (Te, I) times and normalized amplitude (J) between trot at 50 cm/s on the treadmill, and air puff induced trot and gallop. Note that breathing rate is most significantly increased at gallop. Data are presented as mean ± SD (trot treadmill: N = 7 mice; trot air puff: N = 4 mice; gallop air puff N = 7 mice) and p values indicated are obtained from Mann-Whitney U tests.
-
Figure 4—source data 1
Circular plots (panels C and F).
- https://cdn.elifesciences.org/articles/61919/elife-61919-fig4-data1-v1.xlsx
-
Figure 4—source data 2
Distribution histograms (panels C and F).
- https://cdn.elifesciences.org/articles/61919/elife-61919-fig4-data2-v1.xlsx
-
Figure 4—source data 3
Respiratory parameters (panels G–J).
- https://cdn.elifesciences.org/articles/61919/elife-61919-fig4-data3-v1.xlsx
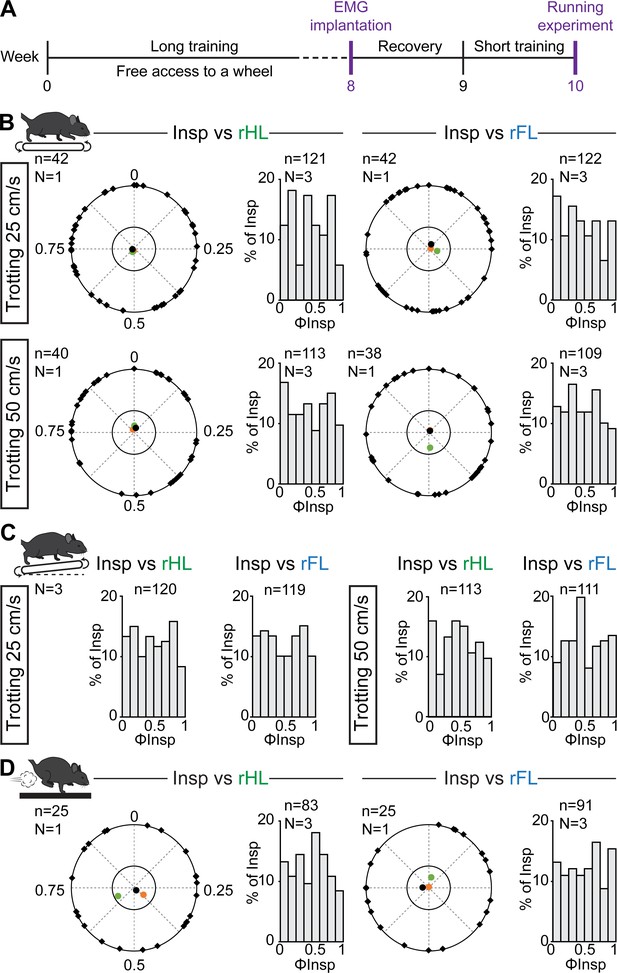
Respiratory changes during running in trained mice and phase-relationship analysis between breaths and strides.
(A) Experimental timeline. Mice were trained for 8 weeks before being implanted for EMG recordings of the diaphragm. (B) Circular plots showing the phase-relationship between inspiratory bursts and the indicated reference limb for one representative animal trotting at 25 (top) and 50 cm/s (bottom). Black diamonds on the outer circle indicate the phase of n individual inspirations. The black dot indicates the mean orientation vector for that animal and the colored dots the mean orientation vector of two other animals. The positioning of these mean values within the inner circle illustrates the absence of a significantly-oriented phase preference (R < 0.3, with R being the concentration of phase values around the mean as defined in Kjaerulff and Kiehn, 1996). Bar-graphs to the right are distribution histograms of the phases of inspiratory bursts and the same reference limb for all n events from three animals. (C) Phase distribution histograms between inspiratory bursts and the indicated reference limb for all n events from three animals running at 25 (left) or 50 cm/s (right) on the inclined treadmill. (D) Circular plot showing the phase-relationship between inspiratory bursts and the indicated limb for one representative trained animal during gallop. Black diamonds on the outer circle indicate the phase of n individual inspirations. The black circle indicates the mean orientation vector for that animal and the colored circles the mean orientation vector of two other animals. To the right are phase distribution histograms between inspiratory bursts and the same reference limb for all n events from three animals. Note that all inspiratory bursts are evenly distributed across the entire locomotor cycle in all conditions.
-
Figure 5—source data 1
Circular plots (panels B and D).
- https://cdn.elifesciences.org/articles/61919/elife-61919-fig5-data1-v1.xlsx
-
Figure 5—source data 2
Distribution histograms (panels B–D).
- https://cdn.elifesciences.org/articles/61919/elife-61919-fig5-data2-v1.xlsx
Tables
Reagent type (species) or resource | Designation | Source or reference | Identifiers | Additional information |
---|---|---|---|---|
Strain strain background Mus musculus | C57BL/6J | Janvier Labs | IMSR Cat# JAX:000664, RRID:IMSR_JAX:000664 | https://www.janvier-labs.com/en/fiche_produit/c57bl-6jrj_mouse/ |
Software, algorithm | Labscribe NI | iWorxs | https://www.iworx.com/products/biomedical-engineering/labscribeni/ | |
Software, algorithm | Clampfit (pCLAMP 11) | Molecular Devices | RRID:SCR_011323 | https://www.moleculardevices.com/products/axon-patch-clamp-system/acquisition-and-analysis-software/pclamp-software-suite#Resources |
Software, algorithm | Deeplabcut | Mathis Lab | http://www.mousemotorlab.org/deeplabcut | |
Software, algorithm | ToxTrac | Rodriguez et al., 2017 | https://sourceforge.net/projects/toxtrac/ | |
Software, algorithm | GraphPad Prism 7 | RRID:SCR_002798 | https://www.graphpad.com/ | |
Software, algorithm | 2nd Look | IO Industries | https://shop.ioindustries.com/products/2ndlook |