Sensory coding and the causal impact of mouse cortex in a visual decision
Figures
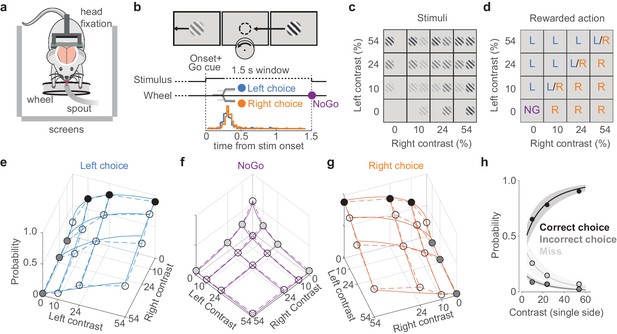
A visual discrimination task affording multiple types of error.
(a) Behavioral setup, with the mouse surrounded by three screens. A spout delivers water rewards. (b) Example stimulus, with higher contrast on the right than left. The correct movement is to turn the wheel anticlockwise to bring the right stimulus to the middle (arrow and circle, not visible to the mouse). Middle: task timeline. Trials began after the mice held the wheel still for 0.2–0.6 s. Grating onset was accompanied by an auditory Go cue. Mice could then make a choice (Left/Right) or hold the wheel for 1.5 s (NoGo). Trials were separated by a 1 s interval. Bottom: distribution of movement onset times (‘reaction time,’ see Materials and methods) for correct Left (blue) and Right (orange) choices from one example mouse. (c) The 16 stimulus conditions. Gratings on each side could have one of four contrasts. (d) Rewarded actions depended on stimulus condition: Left (L), Right (R), NoGo (NG). When the contrasts were equal, Left and Right choices were rewarded randomly (L/R). (e) Probability of Left choices as a function of left and right contrast, averaged over 34 sessions in six mice (circles and dashed lines). Colored circles highlight unilateral stimulus conditions summarized in (h), where choices are Correct (black) or Incorrect (dark gray). Blue curves indicate the mean fit of a psychometric model fitted with hierarchical priors (see Materials and methods; Burgess et al., 2017). (f, g) Same format as in (e), showing NoGo trials and Right choice trials. Light gray dots indicate unilateral stimulus conditions where NoGo counts as a Miss. (h) Summary of these choices for unilateral stimulus conditions. Circles (with color fills as in e–g) show the probability of a Correct choice (black), Incorrect choice (dark gray), and incorrect NoGo (Miss; light gray). Curves and shaded regions are the mean and 95% credible intervals of the mean fit from the psychometric model.
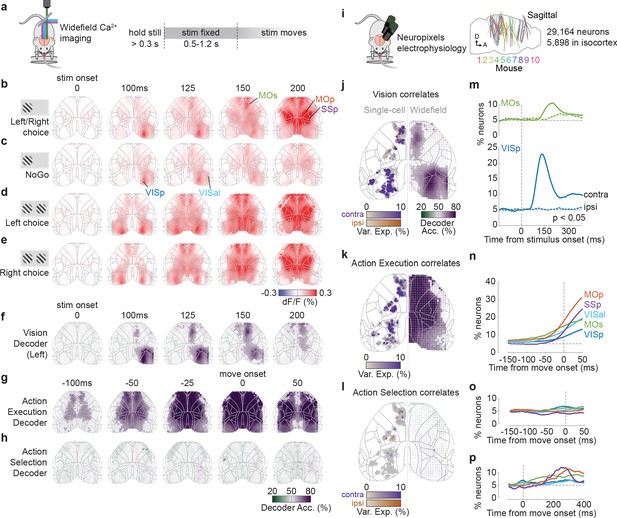
Activity related to stimuli and movement in the dorsal cortex.
(a) Schematic of widefield calcium imaging. Mice expressed GCaMP6 in excitatory neurons (n = 7 mice) or all neurons (n = 2). Right: task timeline. Stimuli were fixed in place for 0.5–1.2 s to dissociate wheel turns from visual motion in recorded activity. (b) Cortical fluorescence (dF/F) for successive times relative to stimulus onset, averaged across 39 sessions in nine mice. Fluorescence is shown for trials with stimuli on the left (avg. 66% contrast) and a Left or Right choice. Gray lines: cortical areas defined from the Allen Common Coordinate Framework (CCF, image cropped to this region; Wang et al., 2020). The image is built of dots (one for each image pixel), whose shade reflects dF/F and whose size reflects statistical significance (larger dot: p<0.001, nested ANOVA). (c) Average dF/F for the same stimulus conditions as in (b) but for Miss (NoGo) trials. (d, e) Average dF/F for trials with equal non-zero contrast on both sides and a Left (d) or Right (e) choice. (f) Average Vision decoder accuracy, decoding whether a stimulus was present on the left screen from each pixel of the widefield image (see Materials and methods). Dot size reflects statistical significance as in (b). (g, h) Average Action Execution decoder accuracy (g; Go vs. NoGo) and Action selection decoder accuracy (h; Left vs. Right), aligned to movement onset. (i) Schematic of Neuropixels recordings. Right: sagittal map of probe insertion sites over 39 sessions in 10 mice (5898 cortical neurons). (j) Vision correlates. Comparison between contralateral Visual decoding maps obtained from widefield data (right hemisphere; 125 ms after stimulus onset) and a map of single neurons (left hemisphere jittered dots) that significantly encoded contralateral (purple) and ipsilateral (orange) stimuli based on kernel analysis. Dot brightness for neurons scales with the cross-validated variance explained of the stimulus kernel. Gray dots indicate neurons with no significant stimulus kernel. (k) Action Execution correlates. Same plotting scheme as in (j) but comparing widefield Action Execution decoding (Go vs. NoGo) at 25 ms before movement onset with the variance explained of a movement kernel. (l) Action Selection correlates. Same plotting scheme as in (j) but comparing widefield Action Selection decoding at 25 ms before movement onset with the variance explained of a choice kernel for contralateral (purple) and ipsilateral (orange) choices. (m) Proportion of VISp (blue) and MOs (green) neurons with significant contralateral (solid line) and ipsilateral (dashed colored line) stimulus decoding (p<0.05; binary decoder). Dashed gray lines: stimulus onset (vertical) and false-positive rate (horizontal). (n) Proportion of neurons that show significant Action Execution decoding (p<0.05; binary decoder) relative to movement onset. Colored lines indicate neurons grouped by CCF area. Vertical dashed line: movement onset. (o) Proportion of neurons with significant choice decoding (p<0.05; binary decoder). (p) Same as (o) but showing later times after movement onset.
© 2017, Steinmetz et al. https://creativecommons.org/licenses/bync/4.0/ Panel i adapted from Steinmetz et al., 2017, published under the Creative Commons Attribution - Non Commercial 4.0 International Public License (CC BY-NC 4.0).
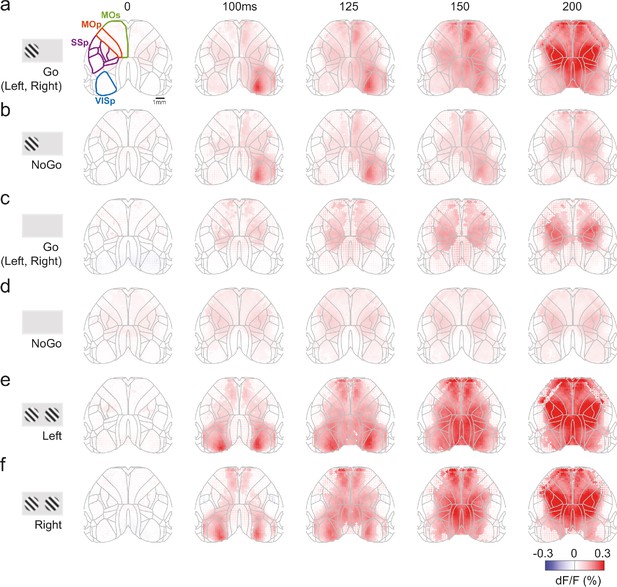
Average cortical fluorescence (dF/F) for different task conditions.
(a) Time course of cortical fluorescence, averaged over Go trials (left or right choice), with positive contrast present on the left side and zero contrast on the right. Columns indicate successive time steps after stimulus onset. Each map is overlaid with an outline of cortical regions defined by the Allen Common Coordinate Framework (CCF), and widefield fluorescence is cropped to the outer edges. Four different cortical regions are highlighted in the left panel (VISp, SSp, MOp, MOs). Maps are averaged over 39 sessions in nine mice, with dot size indicating significance across sessions (p<0.001; nested ANOVA). Even though the animal turns the wheel, the stimulus does not move on the screen in this time period (see Figure 2—figure supplement 2a). (b) Average cortical fluorescence for NoGo trials with positive contrast on the left screen. The maps in (a) and (b) are averaged over trials with matched contrast values (c) Average cortical fluorescence for Go trials (Left or Right choice) with zero contrast on both sides. (d) Average cortical fluorescence for NoGo trials with zero contrast on both sides. (e) Average cortical fluorescence for Left choice trials with equal non-zero contrast on both screens. (f) Average cortical fluorescence for Right choice trials with equal non-zero contrast on both screens, and mice made a Right choice. The maps in (e) and (f) are averaged over trials with matched contrast values. Panels (a, b, e, f) are reproductions of Figure 2b–e, added here for completeness.
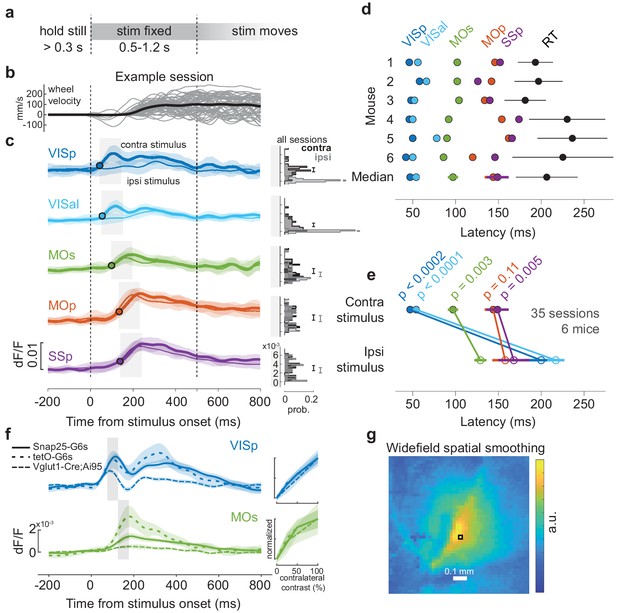
Widefield sequential activation.
(a) Task timeline during widefield calcium imaging. To distinguish activity associated with initial wheel movement from activity driven by visual motion, we introduced an open-loop period 0.5–1.2 s after grating onset, when wheel movements did not move the grating. Trials were excluded post-hoc if choices were made after this period. (b) Stimulus-locked wheel velocity for one example session. Thin lines are individual trials for all correct Left or Right choices (velocity sign flipped for Left choices so that correct is always positive), smoothed with a 10 ms Gaussian window. Thick line: mean. (c) Stimulus-triggered mean calcium fluorescence at five regions of interest (ROIs) for one example session. Thick lines: mean response to correct trials with only contralateral stimuli. Thin lines: same for correct trials with only ipsilateral stimuli. Shaded regions: standard deviation of fluorescence across trials. Black circle: response latency, defined as time for mean fluorescence to reach 30% of the peak. Right: distribution of calcium fluorescence for each ROI across all sessions, taken over a 100 ms time window aligned to response latency (shaded regions on left), separated for contralateral (black) and ipsilateral (gray) stimulus trials. Error bar is 95% confidence interval for the mean fluorescence. (d) Summary of fluorescence response latencies to contralateral stimuli for six mice (see Materials and methods for genotype information; three mice were excluded due to having too few trials to reliably measure onset latency). Rows show data from individual mice, with dots showing each ROI’s response latency for trials pooled across sessions. Bottom row: response latencies and reaction time (RT) averaged across mice (dots and lines: median ± m.a.d.). (e) Response latencies to contralateral and ipsilateral stimuli for each region, averaged across mice (closed and open circles). Significance was determined by a two-tailed paired t-test, t(5) = –10.38, –12.00, −5.46, –1.97, −4.72. Colors as in (c). (f) Stimulus-locked calcium fluorescence at VISp (blue) and MOs (green) ROIs for three different GCaMP6 mouse genotypes (see Materials and methods). Lines indicate the session-averaged fluorescence for trials with only contralateral stimuli, pooling sessions from Snap25-G6s (solid), tetO-G6s (light dashed), and Vglut1-Cre;Ai95 (heavy dashed) mice. Shaded regions indicate the 95% confidence interval. Right inset: fluorescence averaged over time windows depicted by the gray bars on the left, separated for different contralateral contrast values and normalized to the same maximum and minimum across all genotypes. (g) Widefield data were preprocessed by singular value decomposition (SVD), which compresses, smooths, and denoises the calcium signals (Peters et al., 2021). The spatial smoothing that SVD causes depends on the signal statistics and is not spatially homogeneous or isotropic. This pseudocolor image shows the equivalent smoothing kernel resulting from SVD preprocessing, that is, the SVD-smoothed imaging that would result from a raw image with a single pixel over Right VISp (black square), and zero everywhere else. The kernel has ~0.1–0.2 mm diameter. White: scale bar.
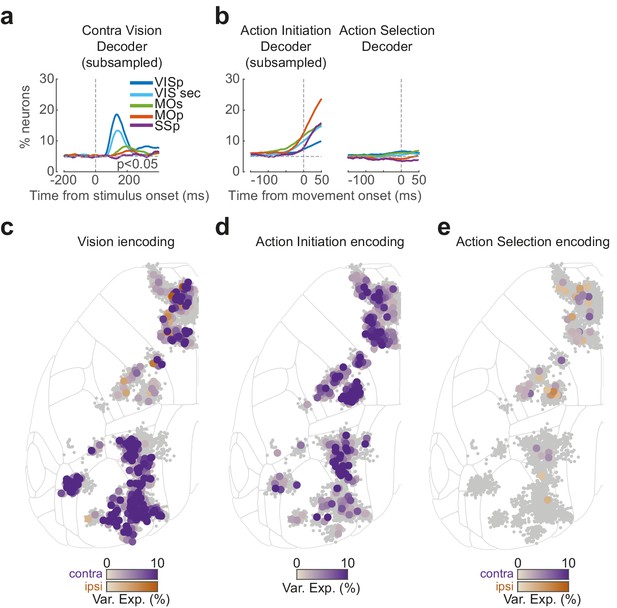
Neuropixels decoding analysis and encoding (kernel regression) analysis.
(a, b) Same binary decoding results as in Figure 2m–p but using a subsampled number of trials to match with the choice decoder. VIS sec represents the signal from multiple secondary visual areas. (c) Enlarged version of Figure 2j, showing single neurons with significant cross-validated variance explained using a contralateral (purple) or ipsilateral (orange) stimulus kernel. (d) Enlarged version of Figure 2k, showing single neurons with significant movement kernels. (e) Enlarged version of Figure 2l, showing single neurons with significant kernels for contralateral (purple) or ipsilateral (orange) choice.
Widefield fluorescence in VISp, MOs, and MOp/SSp for different contralateral and ipsilateral contrast conditions.
Each panel shows the time course of fluorescence in one cortical region, for all 16 contrast conditions. Within each panel, the two black grids show the 40th and 60th percentile (across sessions) of widefield fluorescence (dF/F), averaged over all possible behavioral choices. Different frames of the video correspond to different times after stimulus onset. Time is indicated in the title of each panel.
Average widefield fluorescence in VISp, MOs, and MOp/SSp for different contralateral and ipsilateral contrast conditions, and choices.
Similar to Figure 2—video 1, but dividing responses by behavioral choice. The three grids in each panel show mean cortical fluorescence as a function of contrast condition, separated for contraversive (away from the region of interest [ROI] hemisphere; blue) actions, NoGo (purple), and ipsiversive (towards the ROI hemisphere; orange) actions. Conditions where there are fewer than 0.5 trials averaged across sessions (e.g., trials with high contrast on one side but the mouse moved the opposite direction) are excluded from plotting.
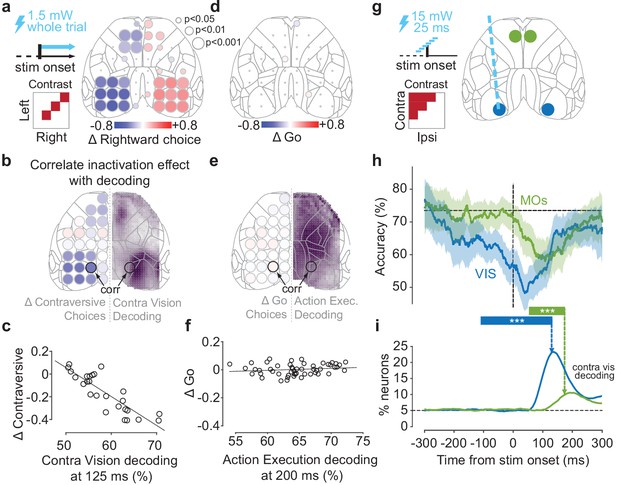
Optogenetic inactivation of sensory-coding regions biases choices.
(a) Scanning inactivation in mice expressing channelrhodopsin-2 (ChR2) in parvalbumin-expressing inhibitory neurons (91 sessions in five mice; see Materials and methods). On ~75% of trials, a blue laser (1.5 mW, 40 Hz sine wave) illuminated one of 52 locations, from stimulus onset until a choice or NoGo was registered. Right: change in probability of rightward choices for 52 stimulation sites for trials with equal non-zero contrast on each side. Dot size indicates statistical significance (permutation test, see Materials and methods). (b) Comparison of the maps for contralateral Vision decoding (right hemisphere, from Figure 2f at 125 ms, dot size not scaled by significance) and for effect of inactivation (left hemisphere; from panel (a) combining across hemispheres). (c) Relationship between the choice bias caused by optogenetic inactivation across 26 sites, and the strength of contralateral stimulus decoding from widefield imaging at 125 ms post-stimulus, averaged over a region around the inactivated coordinate (‘corr’ in panel b). (d) Same as in (a) for the change in proportion of Go (Left or Right) choices for equal non-zero contrast trials. (e, f) Same as in (b, c), for the relationship between Action Execution (Go vs. NoGo) decoding at 200 ms after stimulus onset and the change in Go choices induced from inactivation. (g) Pulse inactivation experiments (65 sessions in six mice). On ~66% of trials, a 25 ms laser pulse was presented randomly between −300 and +300 ms relative to the stimulus onset (vertical dashed line), in one of four locations, chosen randomly (map on right). (h) Trial accuracy as a function of pulse time, averaged over trials where contralateral contrast > ipsilateral contrast. Blue curve: VIS inactivation; green curve: MOs' inactivation; black horizontal dashed line: mean accuracy in no-laser trials. Curves show the average pooled over all sessions and mice, smoothed with a 100 ms boxcar window. Shaded regions: 95% binomial confidence intervals. *** indicates the intervals in which accuracy differs significantly from control trials (p<0.0001; χ2 test). (i) Time course of contralateral Vision decoding (solid lines from Figure 2m). Vertical dashed arrows indicate the end of the critical intervals in (h). Black horizontal dashed line indicates chance level.
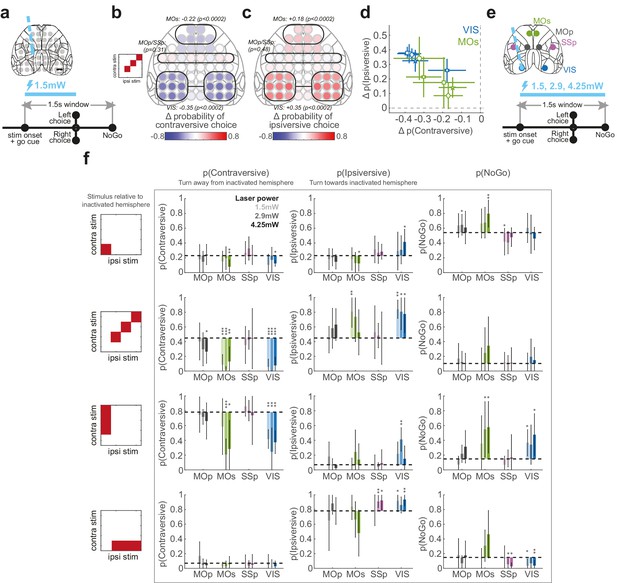
52-coordinate and mixed-power optogenetic inactivation.
(a) Schematic of the 52-coordinate inactivation experiment (91 sessions in five mice). On ~75% of trials, a laser (473 nm, 40 Hz, 1.5 mW) was switched on during stimulus presentation and ceased when a choice (or NoGo) was registered. The location of the laser varied randomly trial-to-trial over 52 cortical sites. (b) Summary map of the effect of laser inactivation on contraversive choices, on trials with equal non-zero contrast on each side. Colors indicate the change in the probability of making a contraversive choice (Left choices for inactivation of right hemisphere locations and Right choices for left hemisphere locations), averaged across 91 sessions in five mice. Data are plotted symmetrically across the hemispheres. Black lines mark regions for which statistical significance was assessed (black italic text) by pooling these regions and shuffling the identities of laser and non-laser trials within each session. (c) Summary map of inactivation effect on ipsiversive choices, plotted as in (b). (d) Mouse-to-mouse variability in inactivation results. Each marker represents the mean change in contraversive and ipsiversive choices for a single mouse on inactivation of any site in VIS (blue) or MOs (green). Each mouse represented by a distinct glyph shape. Error bars represent 95% confidence interval. Dashed gray lines: zero change in choice proportions. (e) Schematic of mixed-power fixed-duration inactivation (34 sessions in six mice), focused on VIS, MOs, MOp, and SSp. Inactivation was performed at several laser powers (1.5, 2.9, 4.25 mW), and the inactivation duration was fixed at 1.5 s, starting at visual stimulus onset. (f) The behavioral effect of inactivation on contraversive, ipsiversive, and NoGo responses (columns) for zero contrast, bilateral, and unilateral stimulus conditions (rows), separated for the different laser powers (shading). Movement direction and stimulus side is expressed with respect to the inactivated hemisphere. Bars indicate the mouse-averaged choice proportions, and error bars indicate 95% confidence intervals on the mean estimate. Dashed gray lines indicate the average choice proportions with no inactivation. Statistical significance across mice was determined using a t-test. Significance results are not corrected for multiple comparisons. ***p<0.001, **p<0.01, *p<0.05.
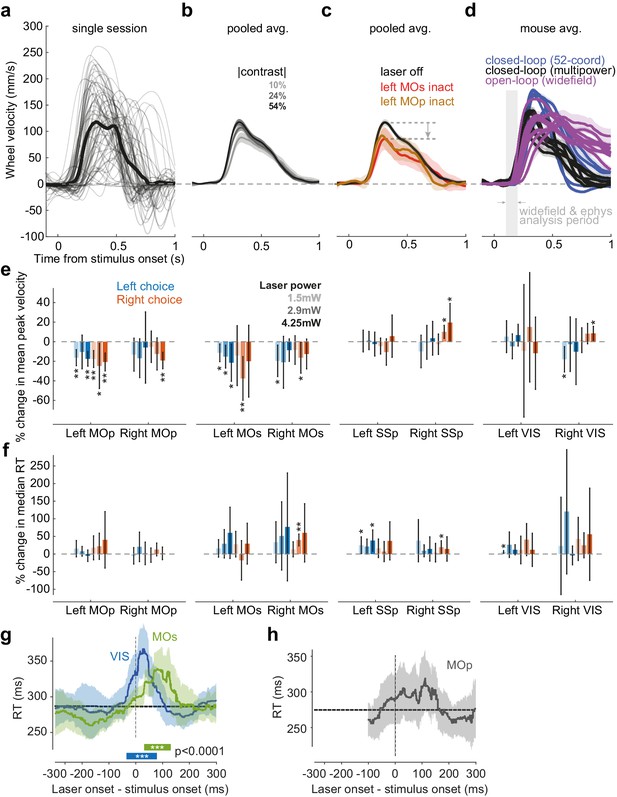
Effect of visual contrast and optogenetic inactivation on wheel movements.
(a) Wheel velocity for a single session, for all correct choice trials with a single stimulus on one side (velocity sign flipped for Left choices so that correct is always positive). Thin lines: single trials smoothed with a 10 ms Gaussian window. Thick line: mean across trials. (b) Mean wheel velocity pooled over all correct non-inactivated single-stimulus trials (34 sessions in six mice), separately for different absolute stimulus contrast. Lines indicate average wheel velocity, and shaded regions indicate 95% confidence interval. (c) Same analysis as in (b) but separated for high-contrast trials (54% contrast) with mixed-power optogenetic inactivation in left MOs (red), left MOp (orange), or with no inactivation (black). Gray arrow illustrates the decrease in peak velocity introduced by the laser inactivation. (d) Mean wheel velocity for open-loop (widefield imaging) and closed-loop (inactivation, laser off trials) experiments performed across separate experimental rigs. Colored lines indicate the average wheel velocity for each mouse, averaged over high-contrast (54% contrast) correct trials, and shaded regions indicate 95% confidence intervals. Gray shaded region indicates the time window used for analyzing widefield and electrophysiological signals (Figure 2). (e) Summary of the change in peak velocity across all mice, for Left (blue) and Right (orange) choices, for mixed-power inactivation in VIS, MOs, MOp, and SSp in left or right hemispheres (text under plot indicates inactivated region). For each choice and region inactivated, the percentage change in peak velocity is computed within each contrast condition and then averaged across conditions. Bars indicate the mean percentage change in peak velocity across mice, and error bars indicate 95% confidence intervals. Significance across mice is determined by a t-test. Significance results are not corrected for multiple comparisons. ***p<0.001, **p<0.01, *p<0.05. (f) Same analysis as in (e) but showing the percentage change in median reaction time for Left and Right choices. (g) Effect of high-power pulsed inactivation (15 mW, 25 ms) in VIS (blue) and MOs (green) on median reaction time (RT, pooling over 65 sessions in six mice) for correct trials. Same plotting convention as Figure 3h. *** indicates time interval when median RT is significantly increased relative to control trials (p<0.0001; one-tailed Wilcoxon rank-sum test). Horizontal dashed gray line: non-laser median RT. (h) Same as in (g) but for inactivation in MOp. The laser onset time on each trial was chosen randomly between −100 ms and +300 ms relative to stimulus onset on ~12% of trials (pooling data from 31 sessions in six mice). No significant effect of MOp inactivation was found for any time.
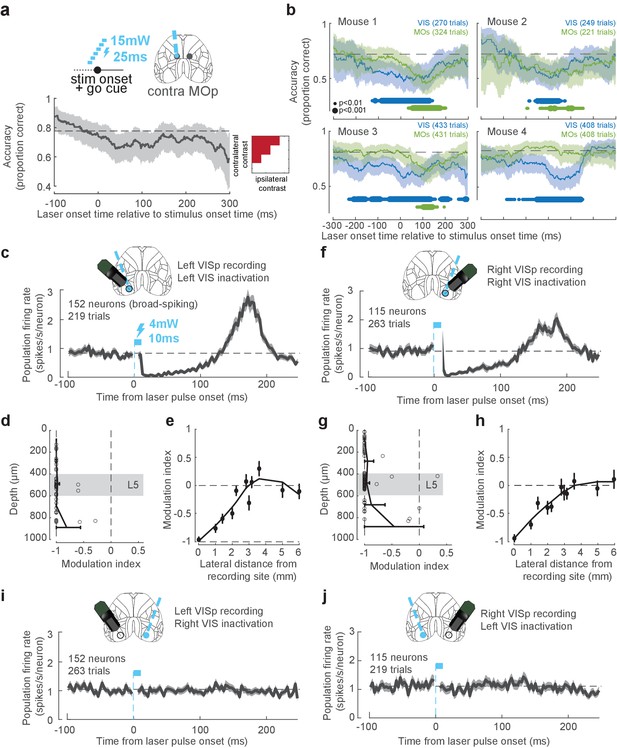
Pulsed inactivation and electrophysiological recording.
(a) Pulse inactivation experiment in MOp. Graph shows performance (percentage correct, averaged over 100 ms boxcar window) as a function of laser onset time in MOp relative to stimulus onset. The laser onset time on each trial was chosen randomly between -100 ms and +300 ms relative to stimulus onset on ~12% of trials (25 ms pulse duration, 15 mW power, pooling data from 31 sessions in six mice). Dashed gray line: non-laser performance. No significant effect of MOp inactivation was found for any time. (b) Pulse inactivation experiment in VIS and MOs, shown for individual mice with at least 60 trials per inactivated region. Same plotting convention as Figure 3h except using a 150 ms boxcar smoothing window. Colored dots indicate times when performance is significantly impaired relative to control trials (small dot: p<0.01, large dot: p<0.001; χ2 test). Number of inactivation trials in each region is shown inset. (c) Firing rate of 152 broad-spiking VISp neurons measured electrophysiologically in the left hemisphere, following a laser pulse (4 mW 10 ms) in the same region (grouped from multiple coordinates), in an awake mouse (Ai32 × PV-cre) not performing a task. The period during the light pulse is masked because recorded voltage deflections during this period may have corresponded to light artifacts. Error bars represent standard error across 219 trials. (d) Effect of inactivation by depth from the cortical surface. Modulation index , where is baseline firing (–100 to –25 ms) and is post-laser firing (+25 to +100 ms), is plotted for neurons in Left VISp spanning different cortical depths, following a laser pulse at the same location. Open circles represent single neurons, and error bars indicate the confidence interval of the modulation index for neurons binned by depth. Shaded region: approximate position of layer 5 based on the density of neurons. (e) Spatial resolution of pulsed inactivation across cortical distance. Modulation index is plotted for Left VISp neurons recorded during pulsed laser illumination centered at different distances from the recording electrode. Dots and error bars indicate mean and 95% confidence interval of the modulation index across neurons. Black line is a LOESS smoothed curve. (f–h) Same as (c–e) but for 115 broad-spiking VISp neurons in the right hemisphere during pulsed inactivation in the right hemisphere. (i, j) Same as in (c, f) but shown for pulsed inactivation in the hemisphere opposite to the recorded hemisphere.
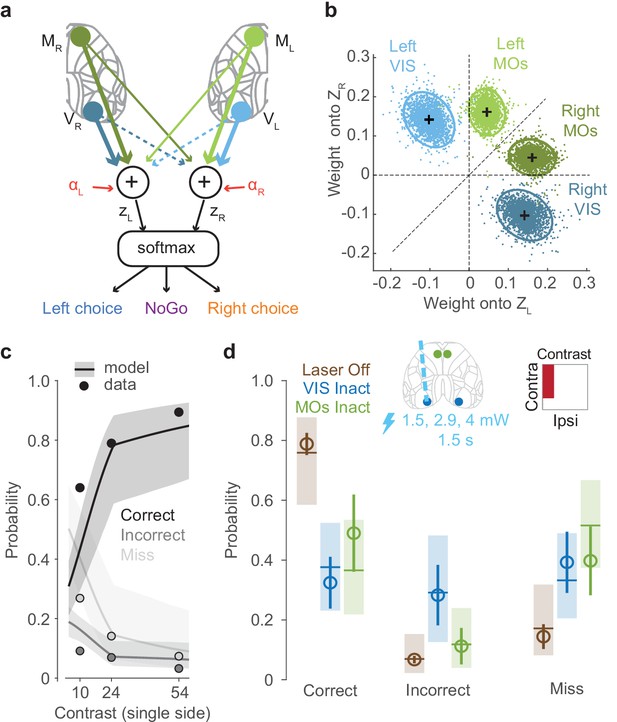
A lawful relationship between cortical activity and decisions predicts the effects of inactivation.
(a) Schematic of the neurometric model. Activity in VISp and MOs is measured by widefield calcium imaging and used to estimate population firing rates on each trial (Figure 4—figure supplement 1). A weighted sum of activity in both hemispheres determines decision variables and , and a softmax function generates the probability of each behavioral choice. (b) Posterior distributions of mouse-averaged model weights from the four regions to the two decision variables. Dots are draws from the posterior distribution of weights (see Materials and methods). Ellipses show 95th percentile of the posterior draws. Diagonal line illustrates the model weight symmetry. (c) Fit of the neurometric model to mouse choices (34 sessions in six mice, non-laser trials), using the same plotting scheme as in Figure 1h. (d) Model predicts effects of inactivation on Correct, Incorrect, and Miss trials, averaging over unilateral stimulus conditions. Open circles show the empirical probability for trials with laser off (brown), and for trials with optogenetic inactivation (1.5 s duration, multiple laser powers; see Materials and methods) of VIS (blue) and MOs (green), averaged across sessions (34 sessions in six mice). Error bars indicate the 95% confidence interval of the average estimate. Brown horizontal lines indicate the neurometric model fit to the average contrast value (~30%). Blue and green horizontal lines indicate mean predictions from the neurometric model, obtained by setting the activity of VISp (blue) and MOs (green) in the model to zero. Shaded regions indicate the 95% credible intervals of the model predictions.
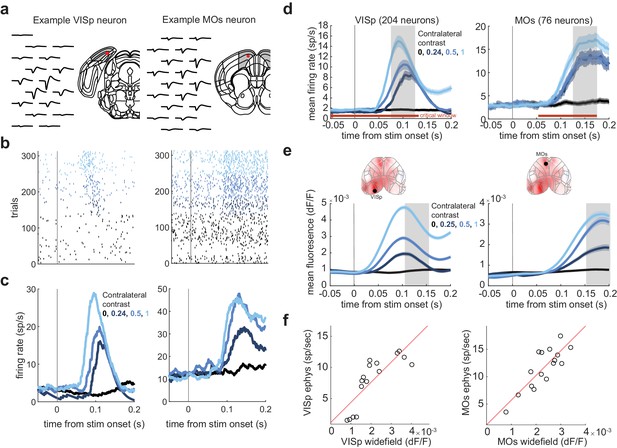
Calibrating widefield activity to spike rate.
(a) Example neuron in Left VISp and Left MOs measured electrophysiologically. Mean waveforms are shown in black, and the red dot marks the location of the neuron within an aligned Allen Common Coordinate Framework (CCF) atlas. (b) Raster plots showing spiking activity aligned to stimulus onset. Color reflects the contrast level presented to the contralateral hemifield (color code in panel c). (c) PSTHs for these example neurons. (d) Population peristimulus time histograms (PSTHs), averaged over 204 neurons in VISp and 76 neurons in MOs. Shaded color region marks the standard error across trials. The gray shaded regions mark the time window when the firing rate is averaged for subsequent analyses (VISp: 75–125 ms; MOs: 125–175 ms). Horizontal red line marks the time of the critical interval identified in the pulse inactivation experiment (Figure 3h). (e) Same plotting convention as in (d) but showing trial-averaged widefield calcium fluorescence of Left VISp (Left) and Left MOs (right) regions of interest (ROIs) in response to stimuli present on the contralateral side. Shaded regions mark the intervals used for averaging in subsequent analyses. This window is 30 ms after the window associated with electrophysiological data to compensate for the slower kinetics of GCaMP6. Insets: ROI locations superimposed on mean fluorescence at 150 ms after stimulus onset. (f) Mean calcium fluorescence vs. population firing rates for Left VISp (left) and Left MOs (right). The 16 open circles correspond to the 16 contrast conditions. Red line corresponds to the fit of a simple linear model f(x) = b0 + b1 * x. The linear model is used to transform widefield fluorescence data into estimates of population firing rate in the neurometric model (Figure 4).
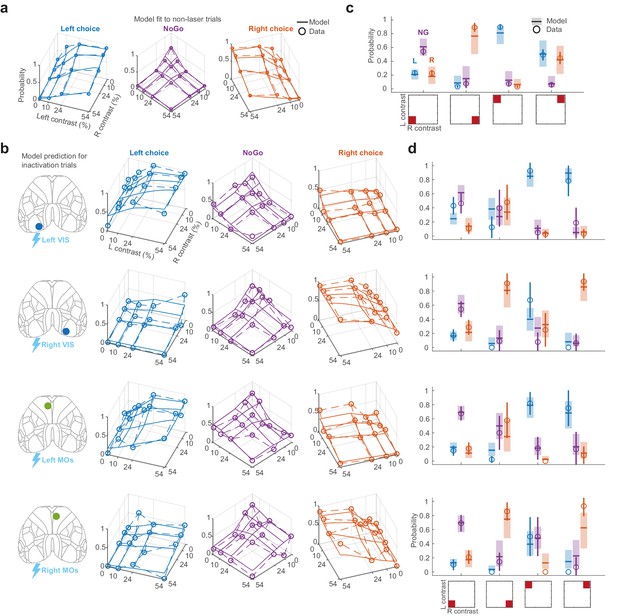
Neurometric model fit and prediction.
(a) Fit of the neurometric model to non-laser trials from the mixed-power inactivation sessions, showing all contrast conditions on the left and right sides. Same plotting scheme as in Figure 1e–g. Empirical data are represented as colored dots for Left (blue), NoGo (purple), and Right (orange) choices, averaged across 34 sessions in six mice. Solid lines correspond to the posterior mean of the neurometric model fit. For each contrast condition, the probability of Left, NoGo, and Right is computed using the fitted weights and average firing rate for each contrast condition (Figure 4—figure supplement 1) with interpolation between the contrast values tested. (b) Same plotting scheme as in (a) but showing the data and the prediction of the neurometric model when inactivating Left VIS, Right VIS, Left MOs, and Right MOs for 1.5 s from stimulus onset, averaged over all laser powers. Model predictions are generated by setting the activity for each region to zero in the model. (c) Alternative visualization of the neurometric model fit to non-laser trials, focusing on trials with zero contrast, high-contrast left or right stimuli, or high-contrast bilateral stimuli (shown inset). Open circles indicate the empirical session-averaged proportion of Left (blue), NoGo (purple), and Right (orange) choices. Error bars are 95% confidence intervals. Colored lines and shaded region indicate the mean and 95% credible intervals of the posterior fit of the neurometric model. (d) Same as in (c) but showing the data and neurometric model prediction for each inactivated region corresponding to same row in panel (b).
Additional files
-
Supplementary file 1
Mouse genotype and session count for each figure.
Table lists the mice used in this study, sex/genotype information, and the number of sessions used for the data in each figure panel.
- https://cdn.elifesciences.org/articles/63163/elife-63163-supp1-v1.docx
-
Transparent reporting form
- https://cdn.elifesciences.org/articles/63163/elife-63163-transrepform-v1.docx