A conserved cell division protein directly regulates FtsZ dynamics in filamentous and unicellular actinobacteria
Figures
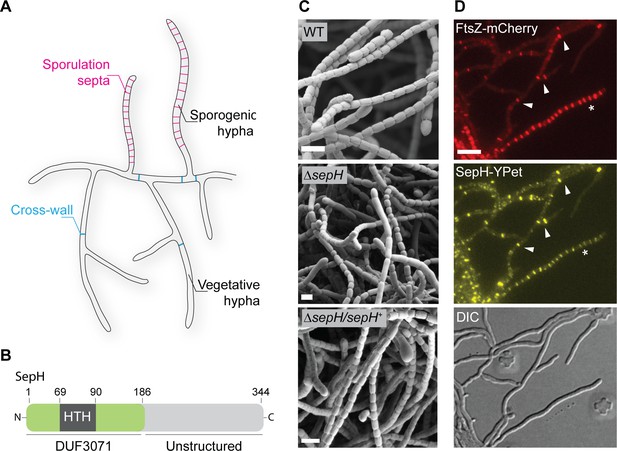
sepH is required for sporulation septation in Streptomyces venezuelae.
(A) Schematic illustrating the multicellular life style of Streptomyces including the two FtsZ-dependent modes of cell division that occur in vegetative and sporogenic hyphae: cross-wall formation and sporulation septation. (B) Schematic of the predicted SepH domain organization including the N-terminal DUF3071 domain containing a helix-turn-helix (HTH) motif and the unstructured C-terminal domain. Numbers indicate corresponding amino acid positions. (C) Cryo-scanning electron micrographs of sporogenic hyphae from wild-type (WT) S. venezuelae, the ΔsepH mutant (SV56), and the complemented mutant strain ΔsepH/sepH+ (MB747). Scale bars: 2 μm. (D) Subcellular co-localization of fluorescently labeled FtsZ (FtsZ-mCherry) and SepH (SepH-YPet) in vegetative and sporulating hyphae. Fluorescent gene fusions were expressed in the WT background (MB751). White arrow heads point at co-localization at cross-walls in vegetative hyphae and the asterisk denotes a sporogenic hypha undergoing sporulation septation. Scale bar: 5 µm.
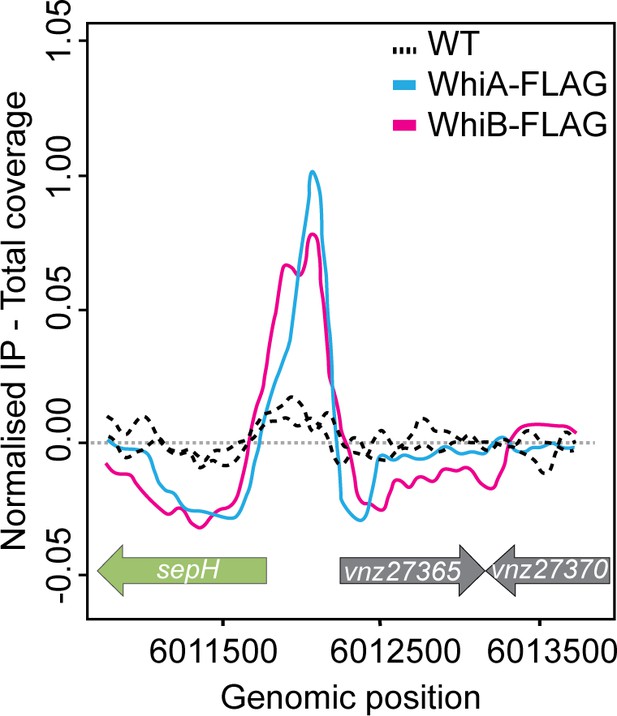
sepH is a direct target of the transcriptional regulators WhiA and WhiB.
ChIP-seq traces showing the enrichment of the FLAG-tagged developmental regulators WhiA and WhiB at binding sites upstream of sepH (vnz_27360) or their absence in the untagged wild-type (WT) control sample. Source data: Bush et al., 2013.
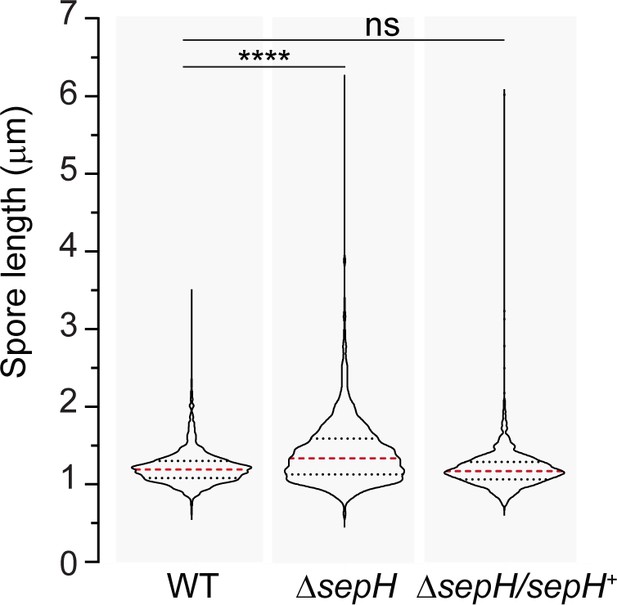
Spore length analysis of wild-type (WT) S. venezuelae, the ΔsepH mutant (SV56), and the complemented mutant strain ΔsepH/sepH+ (MB747).
A minimum of 347 spores were quantified for each biological replicate (n = 3) and strain. The dashed red lines indicate the median, and black dotted lines the 25/75th percentiles. Statistical comparisons were made using a one-way ANOVA test followed by a Dunnett’s multiple comparison test comparing the means to the WT mean. ****p<0.0001; ns, not significant.
-
Figure 1—figure supplement 2—source data 1
Spore size measurement data.
- https://cdn.elifesciences.org/articles/63387/elife-63387-fig1-figsupp2-data1-v1.xlsx
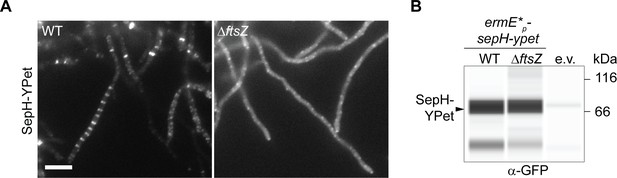
Localization and corresponding protein abundance of SepH-YPet in the wild-type (WT) and the ΔftsZ mutant.
(A) Localization pattern of constitutively produced SepH-YPet in the WT (MB858) and in an ΔftsZ mutant strain (MB859). Scale bar: 5 μm. (B) Virtual automated Western blot showing the accumulation of SepH-YPet produced from the constitutive ermE* promoter in MB858, MB859, and an untagged WT control carrying the empty vector (e.v., SS4). YPet fusions were detected with an anti-GFP antibody (1:200). Shown are representative results of duplicate experiments.
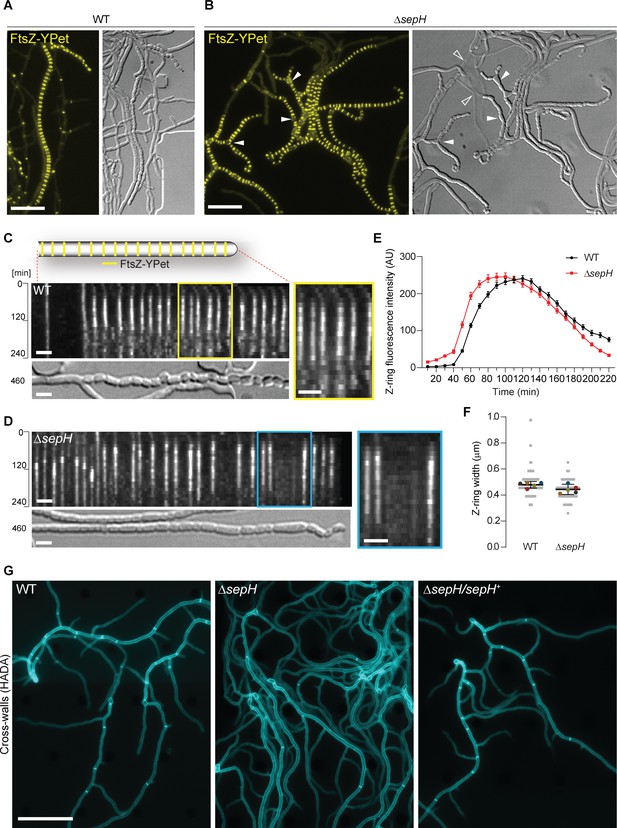
SepH is important for cell division leading to sporulation septa and vegetative cross-walls.
(A and B) Still images from Videos 1 and 2 showing the localization of FtsZ-YPet in sporulating (A) wild-type (WT; SS12) and (B) ΔsepH mutant hyphae (MB750). Arrow heads in (B) point at aberrant spores or gaps in FtsZ-YPet-ladders (filled arrow head) or indicate lysed hyphae (open arrow heads in DIC image). Note that DIC images correspond to a later time point than fluorescence micrographs to show the terminal sporulation phenotype. Scale bars: 10 µm. (C) and (D) Kymograph analysis of FtsZ-YPet dynamics during sporulation-specific cell division in WT (C) and ΔsepH hyphae (D), ectopically expressing an additional copy of ftsZ-ypet from the native promoter (SS12 and MB750). The DIC image below shows the terminal sporulation phenotype. Yellow and blue boxes indicate magnified regions of the kymograph. Scale bar: 2 µm. Additional examples of kymographs can be found in Figure 2—figure supplement 1. (E) Fluorescence intensity traces of FtsZ-YPet (Z-rings) over time derived from sporulating WT (SS12) and ΔsepH mutant hyphae (MB750). Shown are the mean fluorescence intensity traces (mean ± SEM) collected from Z-rings of five sporulating hyphae for each strain. (F) Width of Z-rings in sporulating hyphae of WT (SS12) and sepH-deficient hyphae (MB750). The same data set as in (E) was used and the mean width for reach replicate (colored dots, n = 5) ±95% CI was plotted. (G) HADA labeling of peptidoglycan to visualize cross-walls in WT, ΔsepH (SV56), and ΔsepH/sepH+ (MB747) hyphae. Spores of each strain were germinated and grown in the presence of 0.25 mM HADA for 5 hr in a microfluidic device before imaging. Scale bar: 20 μm.
-
Figure 2—source data 1
Time-lapse fluorescence image series of selected and straightened hyphae (SS12) used to generate kymograph shown in Figure 2C.
- https://cdn.elifesciences.org/articles/63387/elife-63387-fig2-data1-v1.mp4
-
Figure 2—source data 2
Time-lapse fluorescence image series of selected and straightened hyphae (MB750) used to generate kymograph shown in Figure 2D.
- https://cdn.elifesciences.org/articles/63387/elife-63387-fig2-data2-v1.mp4
-
Figure 2—source data 3
Custom Python and R-scripts and extracted fluorescence intensities of Z-rings used to generate Figure 2E and F.
- https://cdn.elifesciences.org/articles/63387/elife-63387-fig2-data3-v1.zip
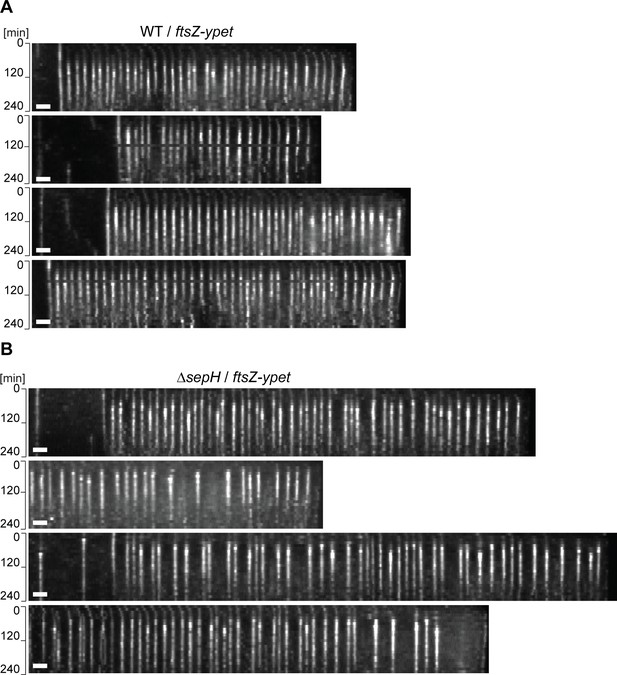
Additional examples of kymographs showing the localization of FtsZ-YPet over time in (A) wild-type (SS12) or (B) ΔsepH hyphae (MB750).
Scale bar: 2 μm.
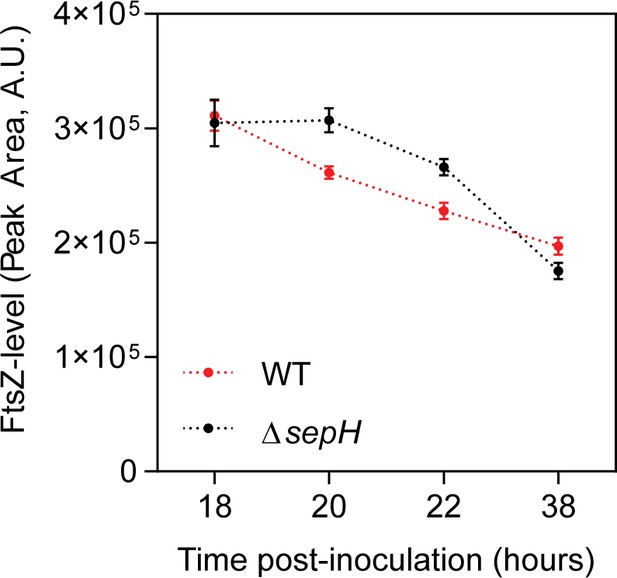
FtsZ levels in wild-type and ΔsepH cells during sporulation.
FtsZ levels were determined by automated Western blot analysis using an anti-FtsZ polyclonal antibody (1:200). Lysates were analyzed in triplicate for each strain and FtsZ levels were quantified at the indicated time-points.
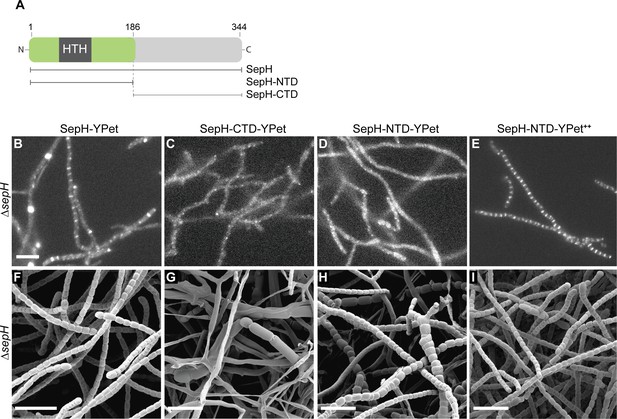
The DUF3071 domain is crucial for SepH function in vivo.
(A) Schematic showing the SepH domain architecture and constructed truncations. Numbers indicate the relevant amino acid positions. (B–E) Fluorescence micrographs showing the localization of the full-length and truncated SepH-YPet variants in the ΔsepH mutant expressed from the native promoter (B–D, strains MB918, MB827, MB828) or from the constitutive PermE* promotor (E, strain MB852). Scale bar: 5 µm. (F–I) Cryo-SEM images of the same strains presented in (B–E) showing the ability of (F) full-length SepH-YPet or (G–I) truncated versions of SepH fused to YPet to complement the sporulation defect of the ΔsepH mutant when produced from the native promoter (F–H, strains MB918, MB827, MB828) or a constitutive promoter (I, strain MB852). Note, expression of sepH-CTD (G) does not rescue the ΔsepH mutant. Scale bars: 5 µm.
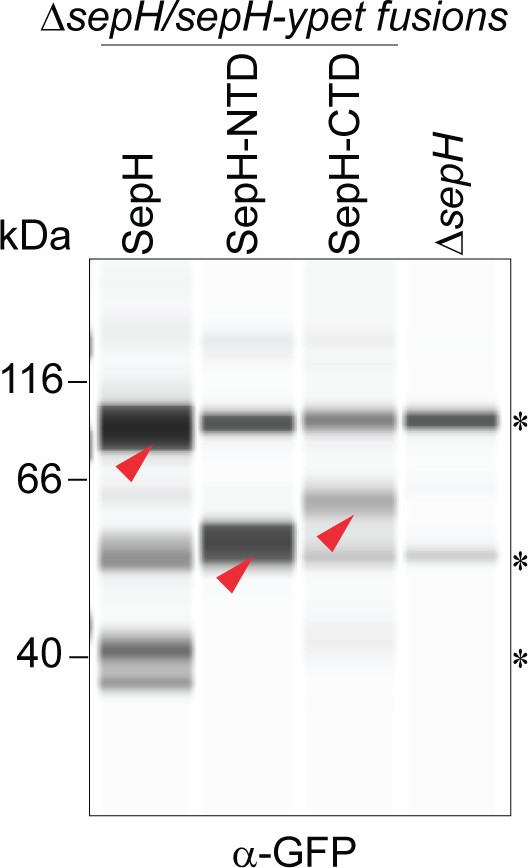
Automated Western blot analysis of the different SepH-YPet constructs shown in Figure 3B–D.
Strains (MB918, MB827, MB828, SV56) were grown to mid-exponential phase and SepH-YPet fusions were detected using an anti-GFP antibody (1:200). Red arrow heads indicate expected size for each construct. Asterisks denote non-specific signals that are also present in the negative control (ΔsepH) or likely degradation products. Shown are representative results of duplicate experiments.
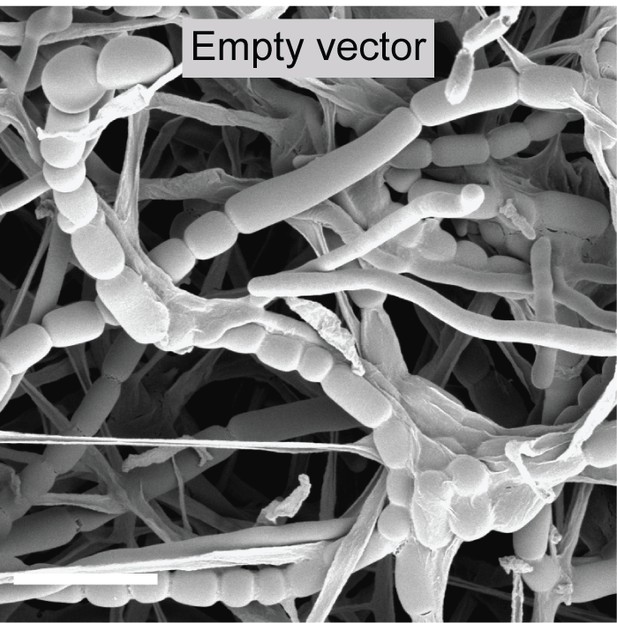
Control image showing the ΔsepH phenotype.
Cryo-SEM image showing sporulating hyphae of ΔsepH carrying an empty plasmid (MB749). Scale bar: 5 μm.
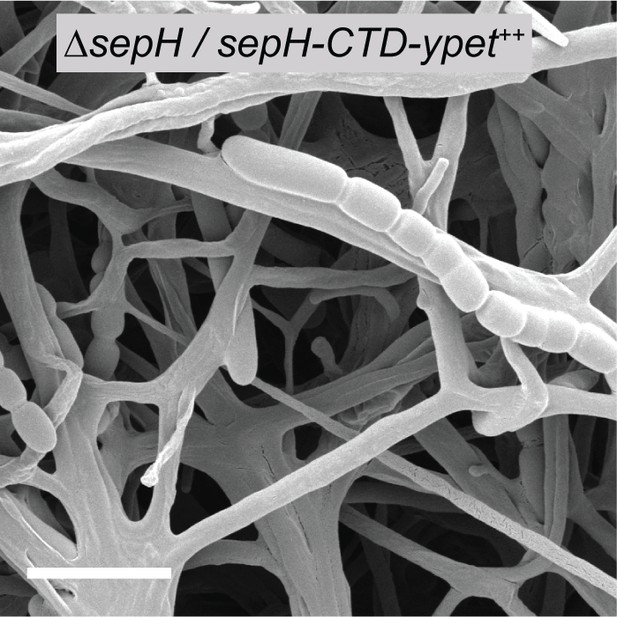
Constitutive expression of sepH-CTD-ypet does not rescue the ΔsepH phenotype.
Cryo-SEM image showing sporulating hyphae of ΔsepH producing SepH-CTD fused to YPet from the constitutive ermE* promoter (MB851, sepH-CTD-ypet++). Scale bar: 5 μm.
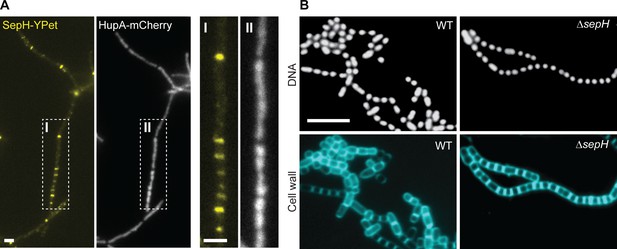
SepH is not associated with the nucleoid or required for chromosome segregation.
(A) Fluorescence micrographs showing the accumulation of SepH-YPet and the concomitant distribution of chromosomal DNA visualized using the nucleoid-associated protein HupA fused to mCherry (MB807). Boxes I and II indicate an enlarged hyphal segment shown in the left panels. Scale bars: 2 µm. (B) Fluorescence images of wild-type (WT) and ΔsepH (SV56) spore chains incubated with the fluorescent dyes 7-AAD and WGA Alexa Fluor 488 to visualize DNA and cell wall material, respectively. Scale bar: 5 µm.
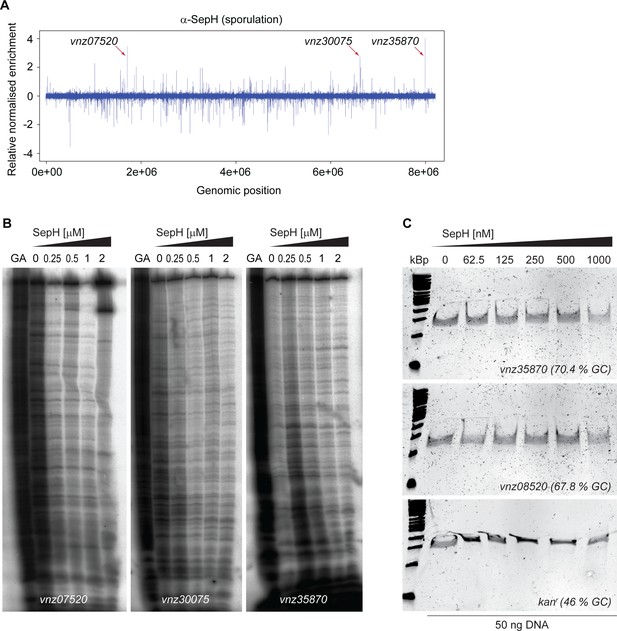
SepH does not bind DNA.
(A) Relative genome-wide distribution of putative SepH binding sites identified by ChIP-seq analysis using an anti-SepH polyclonal antibody during sporulation in wild-type versus ΔsepH (SV56) cells. Arrows point to the three most enriched putative binding sites of SepH upstream of vnz_07520, vnz_30075, and vnz_35870, which were further analyzed in (B) and (C). (B) DNase I footprinting analysis of SepH bound to radiolabeled probes derived from the sequence upstream of vnz_07520, vnz_30075, and vnz_35870. 5’-end-labeled probes were incubated with increasing concentrations of SepH and subjected to DNase I treatment. The footprints are flanked by Maxam and Gilbert sequence ladders (GA). No binding of SepH to the probes could be detected. (C) EMSA analysis to test for non-specific binding of SepH to the promoter region of vnz_35870, a sequence internal to vnz_08520 (ftsZ) and a low-GC sequence from the kanmycin resistance gene (kanr). No binding activity of SepH to any of the tested DNA probes could be detected. Numbers in brackets indicate GC-content of the DNA probe.
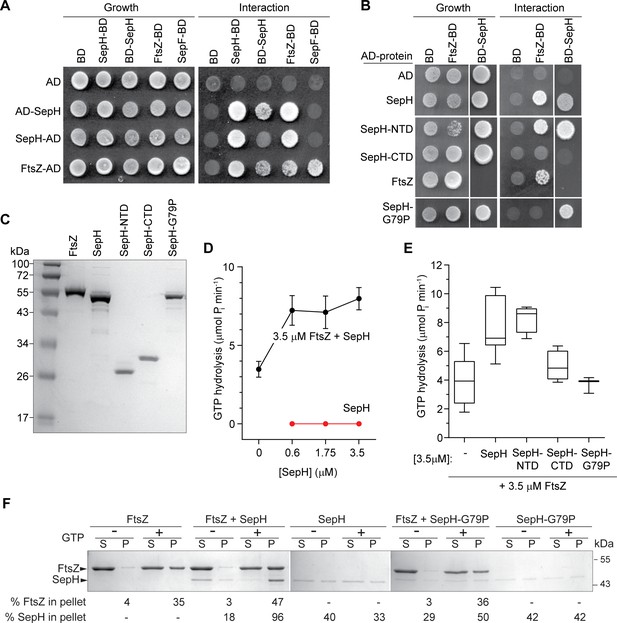
SepH helix-turn-helix (HTH) motif is crucial for the interaction with FtsZ.
(A) Yeast two-hybrid analysis. The indicated proteins were fused to the GAL4 activation domain (AD) and the GAL4 DNA-binding domain (BD). The viability of the yeast strains expressing the respective fusion proteins was confirmed by spotting the individual strains on minimal medium containing leucine and tryptophan (left panel). Interaction between the protein fusion allows growth on minimal medium lacking leucine, tryptophan, histidine, and alanine (right panel). The full set of tested interactions can be found in Figure 5—figure supplement 1. Each interaction was tested in triplicate. (B) Yeast two-hybrid assay showing the interaction between FtsZ and different SepH variants, including full-length SepH (SepH), the N-terminal domain of SepH (SepH-NTD), the C-terminal SepH domain (SepH-CTD), and the mutated SepH HTH domain (SepH-G79P). Experiments were performed as described above. (C) Coomassie-stained SDS gel with purified S. venezuelae FtsZ, SepH, SepH-NTD (residues 1–186), SepH-CTD (residues 187–344), and SepH-G79P. (D) Mean GTP hydrolysis rate of 3.5 µM FtsZ alone or in the presence of increasing concentrations of SepH. SepH did not show GTPase activity (red graph). Error bars represent SEM (n ≥ 3). (E) Mean GTP hydrolysis rate of FtsZ (3.5 µM) in the presence of wild-type SepH and SepH variants at a molar ratio of 1:1. Error bars represent min/max values (n ≥ 3). (F) Co-sedimentation of SepH with polymerized FtsZ in vitro. 3.5 µM FtsZ was incubated for 15 min in the presence or absence of 2 mM GTP, and 0.6 µM SepH or SepH-G79P as indicated. Polymerized FtsZ was collected by high-speed ultracentrifugation. The presence of proteins in the supernatant (S) and pellet (P) was analyzed by SDS-PAGE and Coomassie staining. Representative images of two independent experiments are shown. The percentage of total FtsZ or SepH/SepHG79P in the pellet fraction is indicated below. Note that due to number of samples, protein fractions were loaded on separate protein gels which resulted in slightly different staining intensity.
-
Figure 5—source data 1
High-speed co-sedimentation data used in Figure 5F.
- https://cdn.elifesciences.org/articles/63387/elife-63387-fig5-data1-v1.pptx
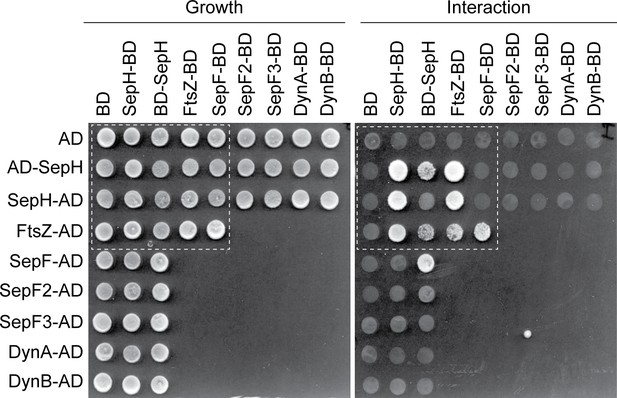
Yeast-two hybrid analysis showing the complete set of tested interactions between SepH and different cell division proteins.
Growth and putative interaction between the different fusion proteins was verified by spotting the individual strains onto minimal media lacking either leucine and tryptophan (growth) or leucine, tryptophan, histidine, and alanine (interaction). The white dashed box indicates the subset of interactions shown in Figure 5A. Each interaction was tested in triplicate and a representative overview of the results is shown.
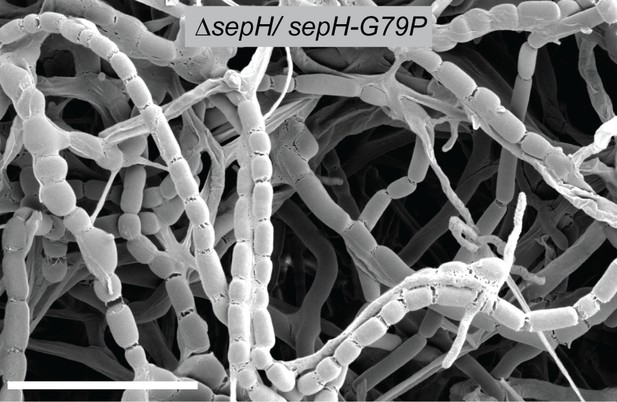
Cryo-SEM micrograph of sporulating ΔsepH hyphae expressing sepH-G79P ectopically from the native promoter (MB938).
Scale bar: 10 µm.
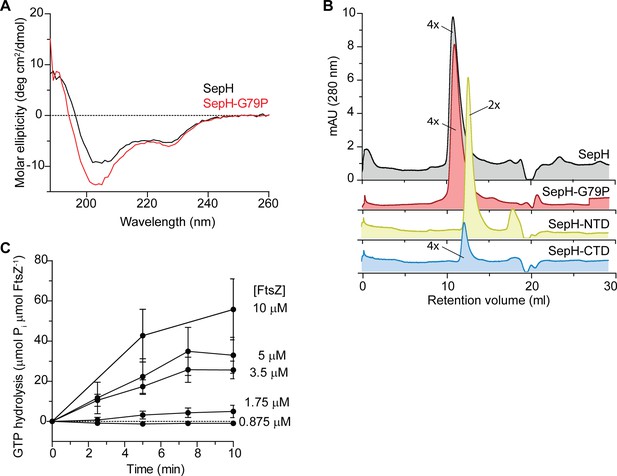
Biochemical characterization of SepH, SepH variants, and FtsZ.
(A) CD spectroscopy analysis of wild-type SepH (black) and SepH-G79P (red). Both proteins show a similar spectral pattern indicating that they are not significantly different in their secondary structure. (B) Size exclusion chromatograms of purified SepH (gray), SepH-G79P (red), SepH-NTD (yellow), and SepH-CTD (blue). Predicted multimerization states of the purified proteins based on the migration of MW standards is indicated (4×, tetramer; 2×, dimer). Shown are representative results of duplicate experiments. (C) Mean GTP hydrolysis rate of increasing concentrations of FtsZ over time. Error bars represent SEM (n ≥ 3).
-
Figure 5—figure supplement 3—source data 1
Analytical gel filtration data.
- https://cdn.elifesciences.org/articles/63387/elife-63387-fig5-figsupp3-data1-v1.xlsx
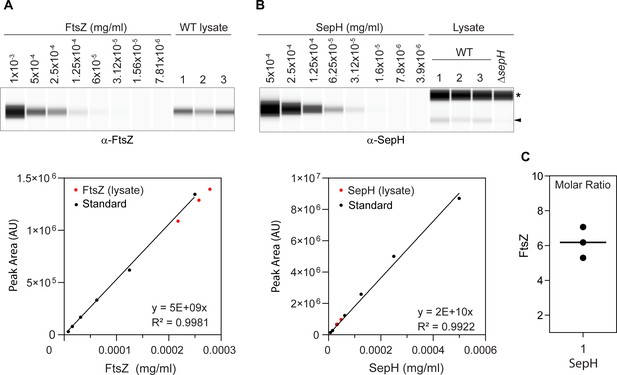
Quantification of SepH and FtsZ abundance by quantitative (automated) Western blotting.
(A) and (B). Top, virtual Western blot image showing a dilution series of purified FtsZ (left) and SepH (right) used to generate a standard curve and endogenous FtsZ and SepH amounts in wild-type cell lysates. Proteins were detected using a polyclonal antibody against FtsZ and SepH. Asterisk denotes an unspecific cross-reaction by the α-SepH antibody. Arrow head points at SepH detected in the cell lysate. Below, the bands for the FtsZ and SepH standards were quantified and fit by a linear function (black circles). The amount of FtsZ and SepH (red circles) was calculated from the calibration. Quantification of SepH and FtsZ in cell lysates was performed in biological triplicate experiments, and automated WES analysis was performed in technical replicates. (C) Calculation of the molar ratio of SepH and FtsZ in S. venezuelae hyphae shows that SepH abundance is approximately sixfold lower compared to FtsZ. Circles present the ratio calculated for each replicate, and the line is the mean.
-
Figure 5—figure supplement 4—source data 1
High-speed co-sedimentation data with GMPCCP.
- https://cdn.elifesciences.org/articles/63387/elife-63387-fig5-figsupp4-data1-v1.pptx
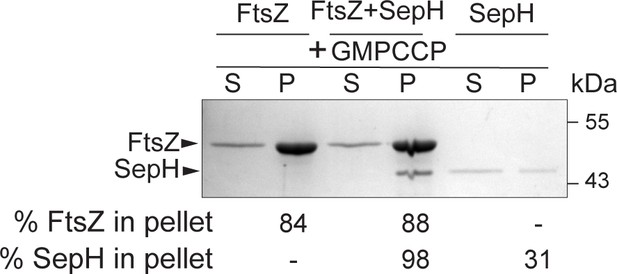
High-speed co-sedimentation with GMPCCP.
3.5 µM FtsZ was incubated with 1 mM GMPCCP and in the presence or absence of 0.6 µM SepH as indicated. Reactions were incubated for 15 min followed by high-speed ultracentrifugation. The presence of proteins in the supernatant (S) and pellet (P) was analyzed by SDS-PAGE and Coomassie staining. A representative image of two independent experiments is shown. The percentage of total FtsZ or SepH in the pellet fraction is indicated below.
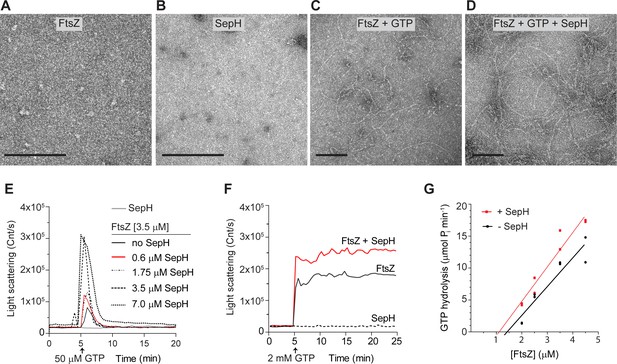
SepH stimulates the polymerization of dynamic FtsZ protofilaments.
(A–D) Visualization of purified FtsZ and/or SepH using negative staining transmission electron microscopy (TEM). No structures were detected for 3.5 μM FtsZ in the absence of GTP (A), or 0.6 μM SepH in the presence of GTP (B). Filaments were observed for FtsZ (3.5 μM) when 2 mM GTP was added (C), and increased FtsZ polymerization was observed when SepH (0.6 μM) was added to the reaction (D). Scale bar: 100 nm. (E) Light scatter traces showing the reversible assembly of 3.5 μM FtsZ filaments in the presence of 50 μM GTP and increasing amounts of SepH. Red line denotes DLS trace generated with a molar ratio of FtsZ to SepH at 6:1. (F) Light scatter traces showing the polymerization of 3.5 μM FtsZ with 2 mM GTP in the presence (red line) or absence of 0.6 μM SepH (black line). SepH alone did not generate light scattering when incubated with 2 mM GTP (dashed line). Light scatter graphs display representative traces of at least three independent experiments. (G) Critical concentration (Cc) of FtsZ from S. venezuelae in the presence and absence of 0.6 μM SepH. Cc was determined by extrapolating the linear regression line of the FtsZ GTPase rate backwards to where it intercepts the X-axis. GTPase hydrolysis rates are the result of two independent experiments.
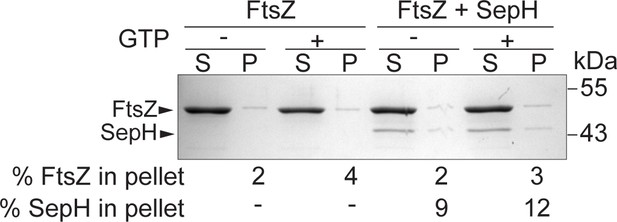
Low-speed co-sedimentation with GTP.
Polymerized FtsZ (3.5 µM) was sedimented in the presence or absence of 0.6 µM SepH and 2 mM GTP following a 15 min incubation period. The presence of proteins in the supernatant (S) and pellet (P) was analyzed by SDS-PAGE and Coomassie staining. A representative image of two independent experiments is shown. The percentage of total FtsZ or SepH in the pellet fraction is indicated below.
-
Figure 6—figure supplement 1—source data 1
Low-speed co-sedimentation data with GTP.
- https://cdn.elifesciences.org/articles/63387/elife-63387-fig6-figsupp1-data1-v1.pptx
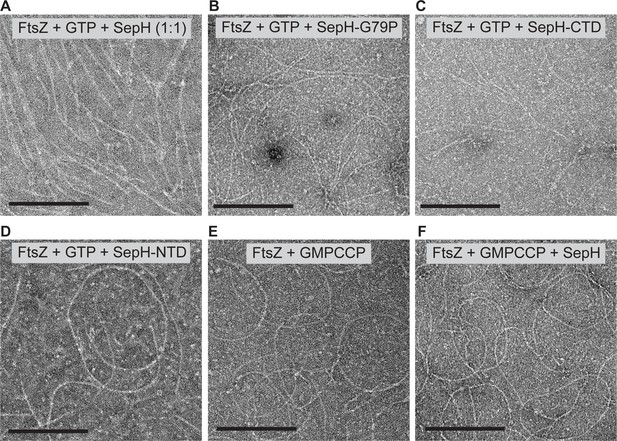
FtsZ filament morphology in the presence of excess SepH, SepH variants, and with GMPCCP.
(A–D) FtsZ (3.5 μM) filaments formed in the presence of 2 mM GTP and with either an equimolar ratio (1:1) of wild-type SepH (A) or at a 6:1 molar ratio with the different SepH variants SepH-G79P (B), SepH-CTD (C), or SepH-NTD (D). (E and F) FtsZ (3.5 μM) filament morphology with and without 0.6 μM SepH when assembled with the slow-hydrolysable GTP analogue GMPCCP (2 mM). Polymerization reactions were incubated for 15 min and FtsZ filaments were visualized by protein negative stain TEM. Scale bars: 200 nm.
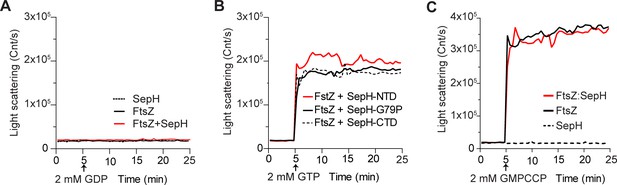
Additional FtsZ polymerization results as measured by DLS.
Light scattering traces of 3.5 μM FtsZ assembly kinetics resulting from incubation with (A) SepH (0.6 μM) and 2 mM GDP, (B) with 2 mM GTP and 0.6. μM of the different SepH variants, or (C) in the presence of the slow-hydrolysable GTP analogue GMPCCP (2 mM) with or without 0.6 μM SepH. Light scatter curves display representative traces of three independent experiments.
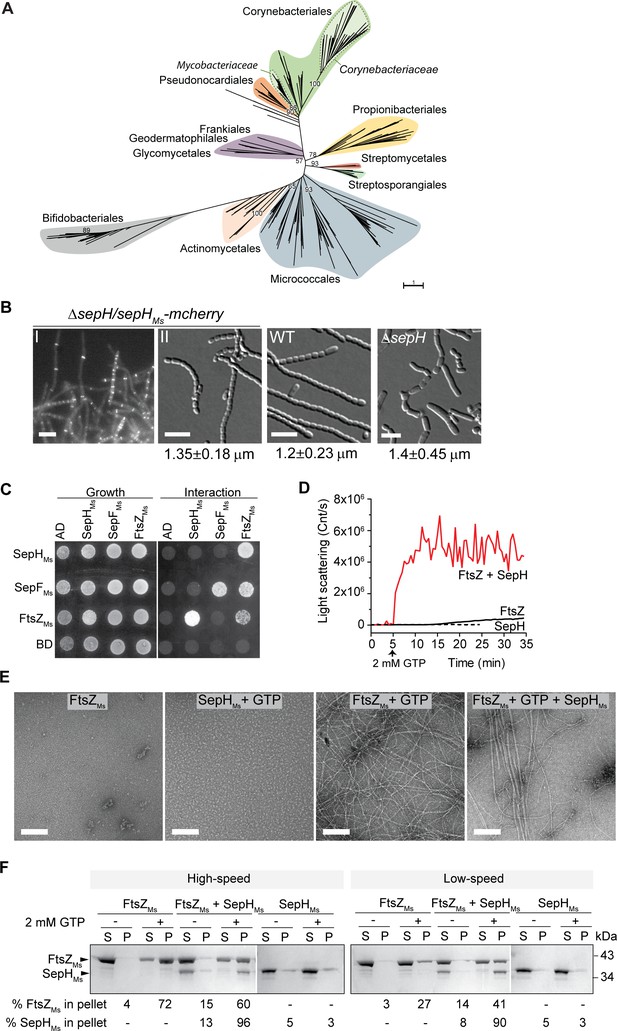
SepHMs stimulates FtsZ polymerization and bundling in vitro.
(A) Phylogenetic tree showing the distribution of SepH within different actinobacterial orders. Major orders with more than two representative leaves are shown in different colors. Numbers denote bootstrap values. The scale bar represents the average substitutions per site. (B) Representative fluorescence and DIC images showing the subcellular localization (I) and wild-type (WT)-like sporulation (II) of the S. venezuelae ΔsepH mutant producing SepHMs-mCherry (SS380). For comparison spore chains of the WT and the ΔsepH mutant (SV56) are shown and the mean ± SD spore length for each strain is denoted below. 350 spores per biological replicate (n = 3) and strain were measured. Scale bars: 5 μm. (C) Yeast two-hybrid analysis to test the interaction between SepHMs and FtsZMs from M. smegmatis. Viability of the yeast strains carrying the respective fusion proteins was confirmed by spotting the individual strains on minimal medium lacking leucine and tryptophan (left panel). An interaction between the protein fusions allows growth on minimal medium lacking leucine, tryptophan, histidine, and alanine (right panel). Shown is a representative image. Experiments were performed in triplicate. (D) Assembly dynamics of FtsZMs from M. smegmatis using dynamic light scattering. Light scatter traces for 6 µM FtsZ (black) and 6 µM FtsZMs in the presence of 3 µM SepHMs (red) are shown. 2 mM GTP was added to induce FtsZ polymerization. Light scatter graphs display representative traces of at least three independent experiments. (E) FtsZMs filament morphology visualized by negative stain TEM of 6 µM FtsZMs alone, with 2 mM GTP and with 3 µM SepHMs. SepHMs (3 μM) does not form visible structures when incubated with GTP. Scale bars: 200 nm. (F) High = and low-speed co-sedimentation assay of polymerized FtsZMs (6 μM) with and without SepHMs (3 μM) in the presence of 2 mM GTP. Presence of FtsZMs and SepHMs in the supernatant (S) or pellet (P) was analyzed by SDS-PAGE and Coomassie staining. The average percentage of total FtsZMs or SepHMs in the pellet fraction based on results from two independent experiments is indicated below. Note that due to the number of samples, protein fractions were run on several protein gels which resulted in different staining intensity.
-
Figure 7—source data 1
Alignment of SepH proteins used to construct phylogenetic tree and phylogenetic tree file with bootstrap values used to generate Figure 7A.
- https://cdn.elifesciences.org/articles/63387/elife-63387-fig7-data1-v1.zip
-
Figure 7—source data 2
Spore measurement data used in Figure 7B.
- https://cdn.elifesciences.org/articles/63387/elife-63387-fig7-data2-v1.xlsx
-
Figure 7—source data 3
Co-sedimentation data used in Figure 7F.
- https://cdn.elifesciences.org/articles/63387/elife-63387-fig7-data3-v1.xlsx
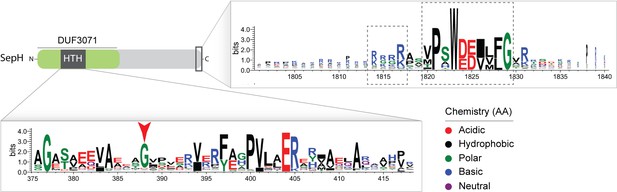
SepH sequence logo.
Logo generated from an alignment of 360 representative actinobacterial SepH sequences. Amino acids are colored according to their chemical properties. The SepH N-terminal region contains a highly conserved helix-turn-helix (HTH) motif. The red arrow head denotes the glycine residue that was substituted in the S. venezuelae SepH-G79P variant. The C-terminal domain contains two additional conserved sequence motifs of unknown function (dashed boxes).
-
Figure 7—figure supplement 1—source data 1
Alignment used to generate SepH sequence logo.
- https://cdn.elifesciences.org/articles/63387/elife-63387-fig7-figsupp1-data1-v1.zip
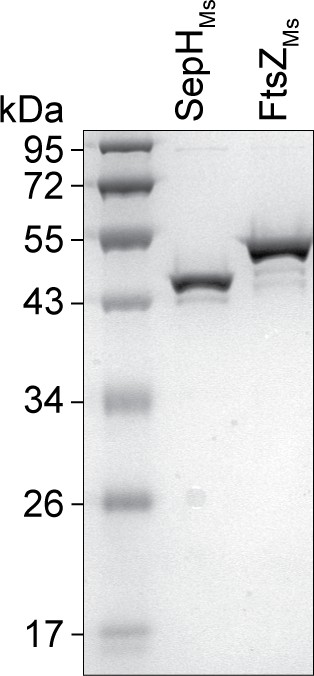
SDS gel showing purified SepHMs and FtsZMs.
Coomassie-stained SDS gel with SepH-6xHis (SepHMs) and untagged FtsZ (FtsZMs) from M. smegmatis.
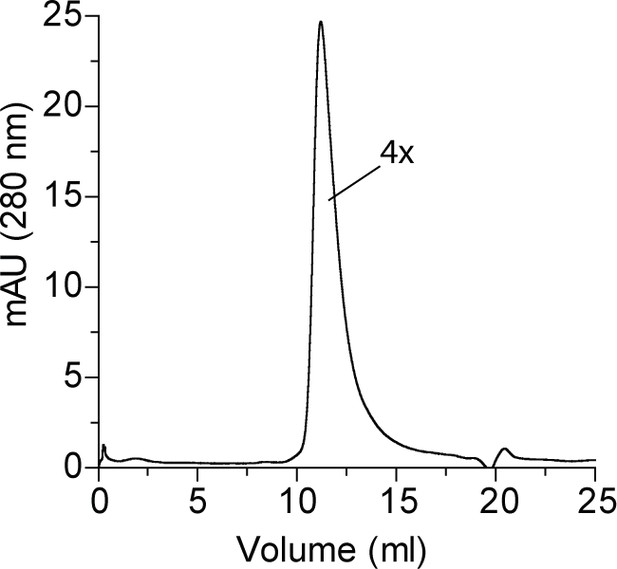
Size exclusion chromatogram of purified SepHMs.
Based on the migration of MW standards, purified SepHMs is predicted to form a tetramer (4×). Experiment was performed in duplicate.
-
Figure 7—figure supplement 3—source data 1
Size exclusion chromatogram data.
- https://cdn.elifesciences.org/articles/63387/elife-63387-fig7-figsupp3-data1-v1.xlsx
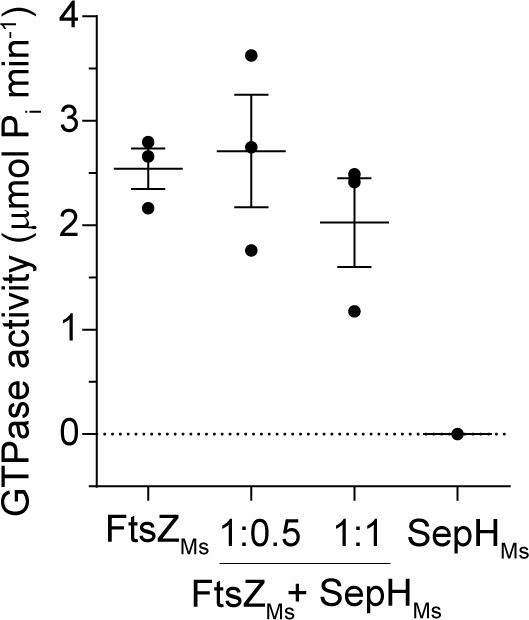
GTP hydrolysis rate of FtsZMs with and without SepHMs.
Mean GTP hydrolysis rates of 6 µM FtsZMs, 3 µM SepHMs, and 6 µM FtsZMs in the presence of 3 µM SepHMs (1:0.5) or 6 μM SepHMs (1:1). Error bars represent SEM (n = 3).
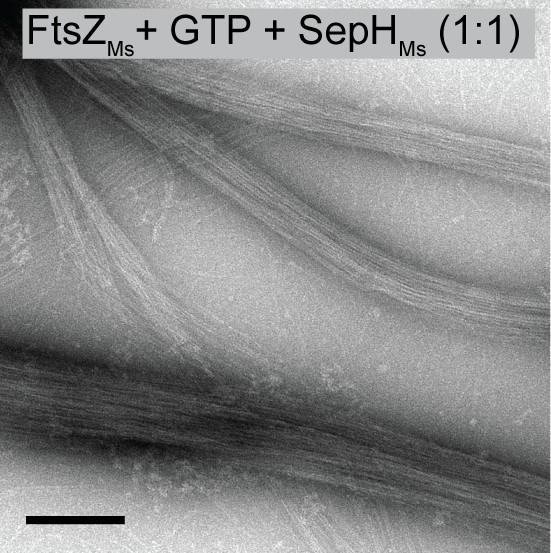
FtsZMs (6 μM) filament bundles formed in the presence of 6 μM SepHMs and 2 mM GTP.
Filaments were visualized by negative stain TEM. Scale bar: 200 nm.
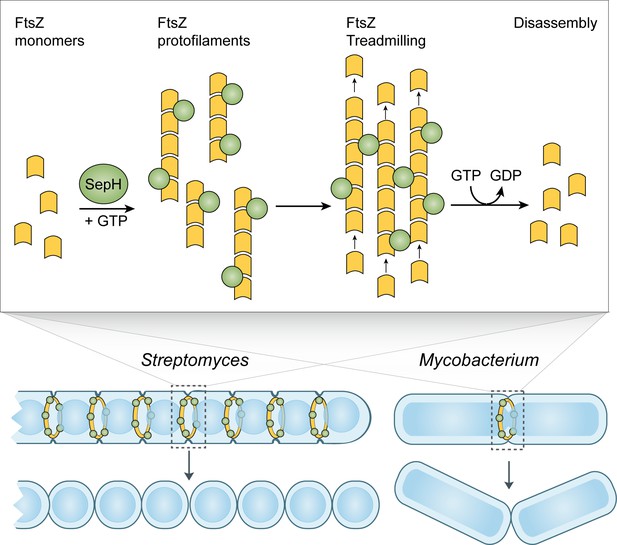
Model of SepH-mediated FtsZ remodeling in Streptomyces and Mycobacterium.
SepH (green) directly binds FtsZ (yellow) and stimulates the robust assembly of FtsZ protofilaments. Filament-associated SepH from M. smegmatis can further mediate lateral interactions between FtsZ filaments while SepH from S. venezuelae is likely to only transiently stabilize FtsZ protofilaments. The GTP hydrolysis rate of FtsZ is likely not directly affected by SepH but will eventually lead to the disassembly of FtsZ filaments. Importantly, SepH functions by increasing the local concentration of FtsZ which promotes the condensation of filaments into a Z-ring and aids FtsZ treadmilling during the early stages of cell division. This process is linked to septal peptidoglycan synthesis and the formation of division septa.
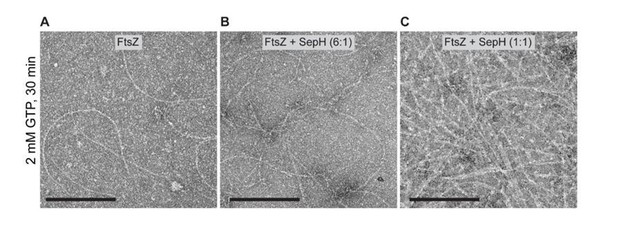
Representative TEM images of negative stained FtsZ polymers after 30 min of incubation with 2 mM GTP and in the absence or presence of either 0.
6 μM SepH (6:1) or 3.5 μM SepH (1:1). Scale bar: 200 nm.
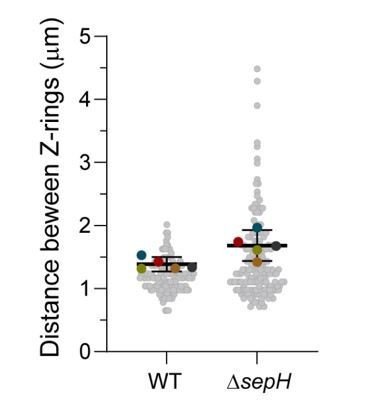
Average distance between Z-rings in wild-type and ∆sepH mutant hyphae.
Results are based on data extracted from 5 independent time-lapse series of sporulating wild-type and sepH-deficient hyphae, respectively, which were also used to generate kymographs shown in Figure 2 and the corresponding figure supplements (main manuscript). Grey dots present entire data set, colored dots are the mean distance for each experimental replicate, line and bars show total mean distance with 95% CI.
Videos
Time-lapse fluorescence and DIC microscopy movie showing the localization of FtsZ-YPet in vegetative and sporulating hyphae of wild-type S. venezuelae (SS12).
Scale bar: 10 μm.
Time-lapse fluorescence and DIC microscopy movie showing growth and localization of FtsZ-YPet in vegetative and sporulating hyphae of the S. venezuelae ΔsepH mutant (MB750).
Scale bar: 10 μm.
Tables
Reagent type (species) or resource | Designation | Source or reference | Identifiers | Additional information |
---|---|---|---|---|
Gene (Streptomyces venezuelae) | sepH | StrepDB | vnz_27360 | http://strepdb.streptomyces.org.uk/ |
Gene (S. venezuelae) | ftsZ | StrepDB | vnz_08520 | http://strepdb.streptomyces.org.uk/ |
Gene (Mycobacterium smegmatis mc2 155) | sepHMs | Mycobrowser | msmeg_5685 | https://mycobrowser.epfl.ch/ |
Gene (M. smegmatis mc2 155) | ftsZMs | Mycobrowser | msmeg_4222 | https://mycobrowser.epfl.ch/ |
Strain, strain background (S. venezuelae) | WT | NZ_CP018074.1 | NRRL B-65442 | Wild type |
Genetic reagent (S. venezuelae) | ΔsepH::apr | This paper | SV56 | Chromosomal sepH locus was replaced by apr-oriT cassette amplified with primers mb118/mb119 and then transduced into WT using ΦSV1 |
Antibody | Anti-SepH (Rabbit polyclonal) | This paper | Cambridge Research Biochemicals | Automated Western blot (1:200) |
Antibody | Anti-FtsZ (Rabbit polyclonal) | This paper | Cambridge Research Biochemicals | Automated Western blot (1:200) |
Antibody | Anti-GFP (Rabbit polyclonal) | Sigma Aldrich | SAB4301138-100UL | Automated Western blot (1:200) |
Recombinant DNA reagent | pTB146 (plasmid) | doi:10.1038/emboj.2008.264 | Plasmid for heterologous protein production | |
Recombinant DNA reagent | pET-21b (plasmid) | Novagen | 69741 | Plasmid for heterologous protein production |
Recombinant DNA reagent | SepH (pFRL39, plasmid) | This paper | sepH in pTB146 | Purification of SepH |
Recombinant DNA reagent | FtsZ, (pSS287, plasmid) | This paper | ftsZ in pTB146 | Purification of FtsZ |
Recombinant DNA reagent | FtsZMs(pSS560, plasmid) | This paper | ftsZMs in pTB146 | Purification of FtsZMs |
Recombinant DNA reagent | SepHMs(pSS561, plasmid) | This paper | sepHMs in pET21b | Purification of SepHMs |
Sequence-based reagent | mb118 | This paper | Redirect PCR primer | CACGTGACGTCGGCAGGCACCACCCGGGAGGTCCCCATGATTCCGGGGATCCGTCGACC |
Sequence-based reagent | mb119 | This paper | Redirect PCR primer | AGCCGCGGAACCGGCGGACCGCCACGGCTCCTGCCGTCATGTAGGCTGGAGCTGCTTC |
Commercial assay or kit | 12–230 KDa Wes separation module | Bio-Techne | SM-W004 | Plate and capillaries for Automated Western blot |
Commercial assay or kit | WES anti-rabbit detection module | Bio-Techne | DM-001 | Secondary antibody, luminol and reagents for Automated Western blot |
Commercial assay or kit | Frozen-EZ Yeast Transformation II Kit | Cambridge Bioscience | T2001 | Yeast two-hybrid analysis |
Commercial assay or kit | Pi ColorLock Kit | Expedeon | 303–0030 | |
Commercial assay or kit | CellASIC ONIX B04A-03 Microfluidic Bacteria Plate | Millipore | B04A-03-5PK | Time-lapse microscopy |
Chemical compound, drug | GTP | Jena Bioscience | NU-1012 | GTPase assay, DLS, TEM, Co- sedimentation |
Chemical compound, drug | GDP | Sigma Aldrich | G7127-10MG | DLS, TEM, Co- sedimentation |
Chemical compound, drug | GMPCPP (GpCpp) | Jena Bioscience | NU-405S | DLS, TEM, Co- sedimentation |
Chemical compound, drug | WGA (Wheat Germ Agglutinin), Alexa Fluor 488 Conjugate | Molecular Probes | W11261 | Cell wall staining |
Chemical compound, drug | 7-AAD (7-Aminoactinomycin D) | Molecular Probes | A1310 | DNA staining |
Chemical compound, drug | HADA (3-[[(7-Hydroxy-2-oxo-2H-1-benzopyran-3-yl) carbonyl]amino]-D-alanine hydrochloride) | Other | Cell wall staining; Gift from M. Thanbichler: synthesized after doi:10.1038/nprot.2014.197 | |
Software, algorithm | Fiji | Open-source software package | Image analysis | |
Software, algorithm | ZenBlue 2012 | Zeiss | Version 1.120 | Image analysis |
Software, algorithm | Compass for SW | Bio-Techne | Version 4.0 | WES |
Software, algorithm | Prism | GraphPad | Version 9.0 | Data analysis |
Software, algorithm | CLUSTALX | http://www.clustal.org/clustal2/ | Phylogenetic analysis | |
Software, algorithm | MAFFT | https://mafft.cbrc.jp/alignment/software/ | Phylogenetic analysis | |
Software, algorithm | MUSCLE | https://www.ebi.ac.uk/Tools/msa/muscle/ | Phylogenetic analysis | |
Software, algorithm | CD-HIT | http://weizhongli-lab.org/cd-hit/ | Phylogenetic analysis | |
Software, algorithm | TRIM-AL | http://trimal.cgenomics.org/ | Phylogenetic analysis | |
Software, algorithm | PHYML | http://www.atgc-montpellier.fr/phyml/ | Phylogenetic analysis | |
Software, algorithm | iTOL | https://itol.embl.de/ | Phylogenetic analysis | |
Software, algorithm | WebLogo3 | http://weblogo.threeplusone.com/ | Sequence logo |
Additional files
-
Supplementary file 1
Tables listing bacterial strains, plasmids, and oligonucleotides used in this study.
- https://cdn.elifesciences.org/articles/63387/elife-63387-supp1-v1.docx
-
Transparent reporting form
- https://cdn.elifesciences.org/articles/63387/elife-63387-transrepform-v1.docx