Presynaptic NMDARs cooperate with local spikes toward GABA release from the reciprocal olfactory bulb granule cell spine
Figures
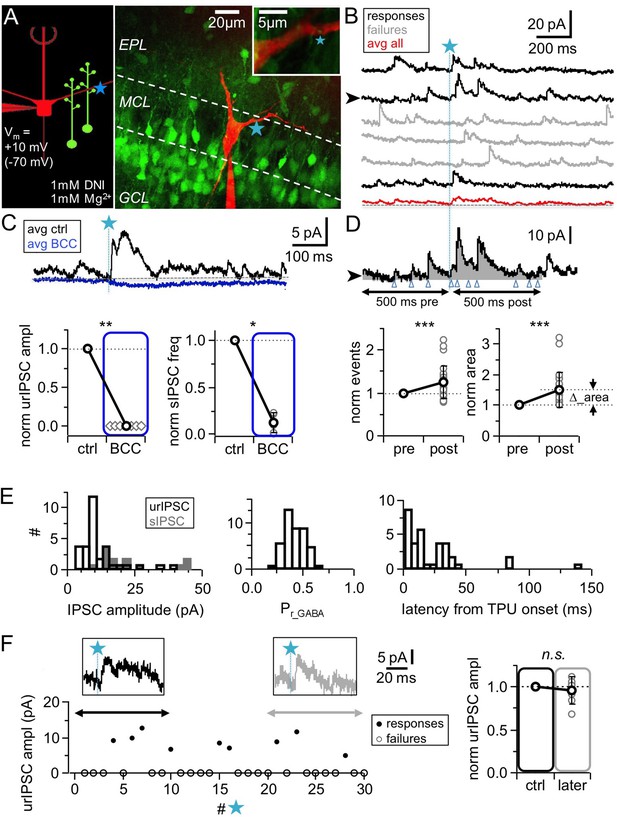
TPU-induced glutamatergic activation of GC spines triggers GABA release detected as uncaging-evoked reciprocal IPSCs (urIPSCs) in MCs.
(A) Experimental setting (scheme and image): Somatic whole-cell voltage-clamp recording from MC filled with Alexa 594 (red, 50 μM) and TPU of DNI-caged glutamate (DNI; blue star) at GC spines in VGAT-Venus rats (GCs green). (B) Example of consecutive uncaging traces showing urIPSC responses (black) and failures (gray); average of all recordings shown below (red). (C) Effect of GABAAR blockade (bicuculline, BCC, 50 µM, blue) on urIPSCs and spontaneous activity. Top: Example experiment, showing averaged traces. Bottom: Cumulative data on urIPSC amplitude (left panel, n = 7 MCs) and on frequency of spontaneous IPSCs (right panel, n = 6 MCs). (D) Analysis of asynchronous events. Top: Example trace from (B) with analysis intervals pre- and post-uncaging (gray areas, black arrows). Counted events marked by blue arrowheads. Bottom: Normalized IPSC event number and area (integrated activity, relative increase vs control ∆) in pre- vs post-uncaging intervals (n = 27 MCs, see Materials and methods). (E) Properties of first detected triggered urIPSCs (see Materials and methods). Left: Amplitudes (n = 32 MCs, Vm = +10 mV). Dark gray: Amplitude distribution of spontaneous IPSCs for comparison (n = 14 MCs, mean amplitudes). Middle: Release probabilities Pr_GABA (n = 44 MCs). Right: Latencies from TPU onset (n = 36 MCs). (F) Stability of urIPSC recordings. Left: Representative experiment showing individual urIPSC amplitude values over consecutive stimulations (1 TPU per min). Insets: Averaged urIPSC responses in the first (black, n = 3 responses) and last ten minutes (gray, n = 3 responses). Right: Comparison of averaged normalized urIPSC amplitudes separated by 10 min interval (n = 7 MCs).
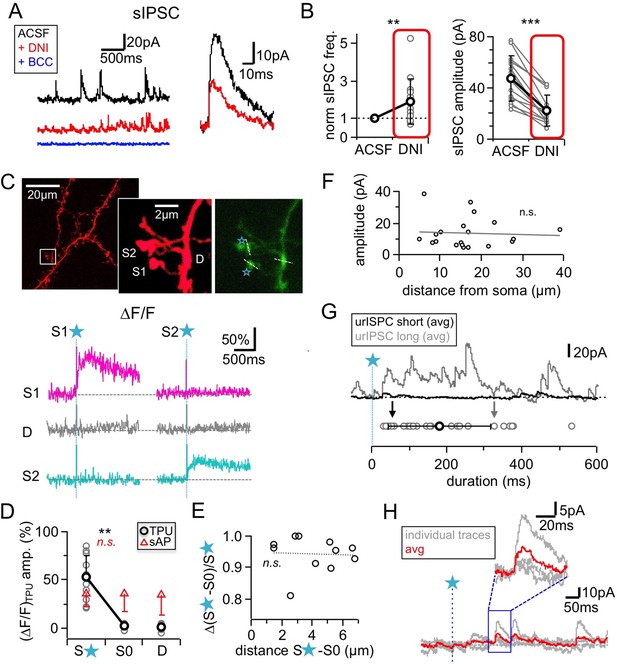
Effects of DNI (1 mM) on sIPSCs, spine-specificity of TPU, TPU sites, duration of responses.
(A) Left: Example recordings in ACSF, after DNI wash-in and with added bicuculline (BCC, 50 µM). Right: Representative single events before and after DNI wash-in. (B) Summary of effects of DNI (red) on spontaneous IPSCs. Left: Frequency. Right: Absolute amplitude. (n = 14 MCs each). (C) Effect of uncaging on non-stimulated adjacent GC spines (S1, S2). Representative experiment. Top: Z-projections of two-photon scan of GC dendrite and spine pair filled with Alexa594 and OGB-1 (right panel, see Materials and methods). Locations of TPU marked with blue stars, location of line scans marked with white dashed lines. Bottom: Averaged fluorescence transients recorded in both spine heads and dendritic shaft in between in response to TPU at S1 only (left) and TPU at S2 only (right). (D) Cumulative data from 11 spine pairs. gray circles: Individual ∆F/F amplitudes in stimulated spine, non-stimulated spine S0 and dendritic shaft. Black circles: mean values. Red triangles: Mean ∆F/F amplitudes in response to single somatically evoked action potential. No significant difference across all three compartments. (E) Cumulative data from the same 11 spine pairs. Linear regression between relative difference in ∆F/F in the stimulated vs the non-stimulated spine head (y-axis) and the distance between the spine head centers (x-axis). No correlation was found (Pearson’s correlation coefficient r = −0.17, p=0.31). (F) Distance of TPU site from soma vs urIPSC amplitudes. There was no detectable correlation (n = 20 MCs, Pearson’s correlation coefficient r = −0.07, p=0.39). (G) Top: Exemplary averaged responses from two experiments. Bottom: Cumulative durations of triggered events (black and gray arrow: durations of exemplary averaged responses). (H) Exemplary experiment with a long latency of first detectable response (see Materials and methods). Inset: Magnification of responses.
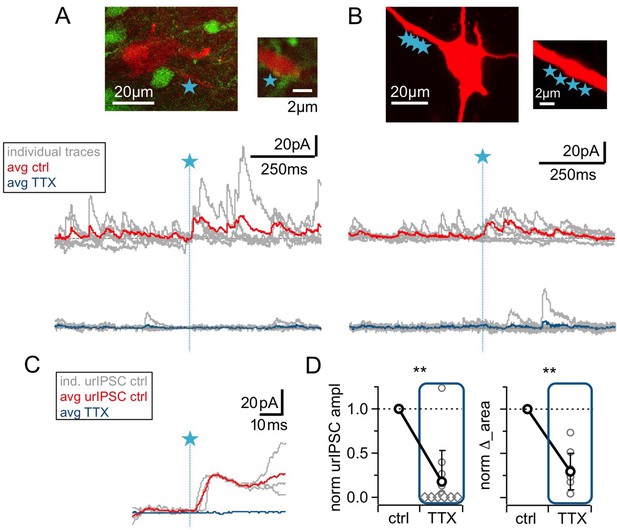
The urIPSC is reduced by Nav blockade (TTX, 500 nM).
(A, B) Representative experiments showing a patch-clamped mitral cell (Alexa594 50 μM, red), the uncaging site(s) along a lateral dendrite (blue star) and below the corresponding uncaging traces with individual traces shown in gray, average control traces in red and average TTX traces indicated in blue (A: VGAT-Venus rat, single-site TPU, B: Wistar rat, multi-site TPU). The average traces contain all recordings, both with and without detected responses. (C) Magnified illustration of traces in (A) (for control individual traces with urIPSC responses and their average, for TTX only average, color coding as above). (D) Left: Cumulative normalized data showing the strong reduction of urIPSC amplitude during Nav blockade with TTX (n = 12 MCs). Diamonds indicate the experiments with no detectable response in the presence of the drug. Right: Comparison of normalized IPSC ∆_area between control and in the presence of TTX (see Materials and methods, n = 10 MCs).
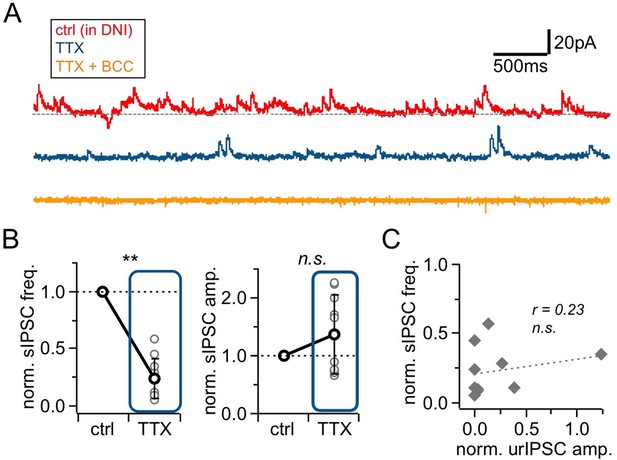
Effects of Nav blockade (TTX, 500 nM) on spontaneous activity.
(A) Representative experiment showing voltage-clamp recordings (+10 mV) from a mitral cell in the presence of DNI (control, top trace), upon addition of TTX (middle trace) and further wash-in of BCC (50 µM; bottom trace). (B) sIPSCs in control and in presence of TTX (n = 10 MCs). Left: Frequency. Right: Amplitude. sIPSCs were abolished in BCC (analysis not shown) (C) Linear regression between the TTX effect on sIPSC frequency vs urIPSC amplitude (n = 10 MCs). No significant correlation.
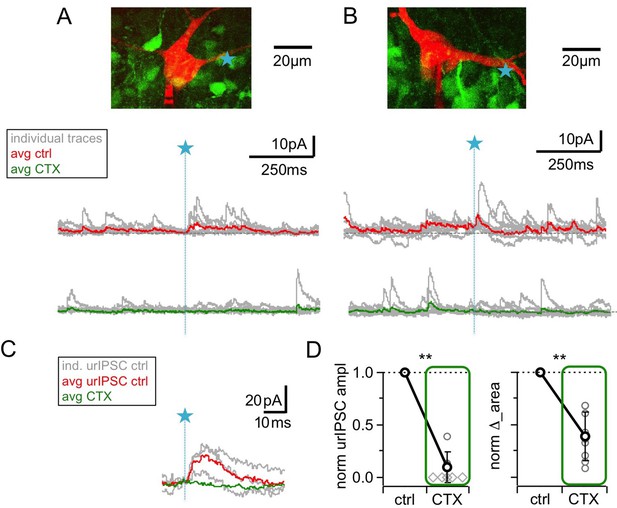
Blockade of high voltage activated Ca2+-channels by ω-conotoxin MVIIC (CTX, 1 μM) causes a prominent reduction of urIPSC amplitudes.
(A, B) Two representative experiments in brain slices from VGAT-Venus rat with the corresponding MC (red), the site of TPU (blue star) and the uncaging traces according to the condition (individual traces: gray, average control: red, average CTX: green). The average traces contain all recordings, both with and without detected responses. Left: same cell as in Figure 1B. (C) Magnified illustration of traces in B (for control individual traces with urIPSC responses and their average, for CTX only average, color coding as above). (D) Left: Summary of effects of CTX on average normalized urIPSC amplitude (n = 8 MCs). Diamonds indicate the experiments with no detectable response in the presence of the drug. Right: Comparison of delta urIPSC areas normalized to control vs in the presence of CTX (n = 9 MCs).
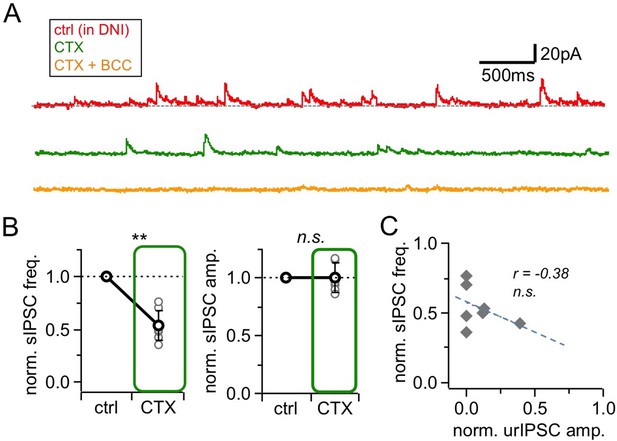
Effects of HVACC blockade by ω-conotoxin MVIIC (CTX, 1 μM) on spontaneous activity.
(A) Representative experiment showing voltage-clamp recordings (+10 mV) from a mitral cell in the presence of DNI (control, top trace), upon addition of CTX (middle trace) and further wash-in of BCC (50 µM; bottom trace). (B) sIPSCs in control and in presence of CTX (n = 7 MCs). Left: Frequency. Right: Amplitude. sIPSCs were abolished in BCC (analysis not shown) (C) Linear regression between the CTX effect on sIPSC frequency vs urIPSC amplitude (n = 7 MCs). No significant correlation.
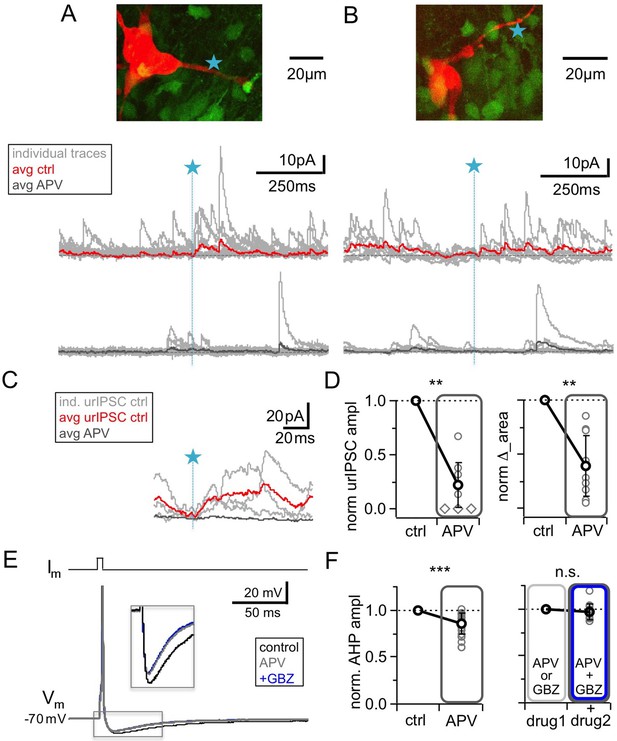
NMDAR blockade with D-APV (25 μM) results in a strong reduction of urIPSC amplitudes and also reduces AHPs following mitral cell APs.
(A, B) Two representative uncaging experiments with the corresponding MC (red), the site of TPU (blue star) and the uncaging traces according to the condition (individual traces: gray, average control: red, average APV: dark gray); both VGAT-Venus. The average traces contain all recordings, both with and without detected responses. (C) Magnified illustration of traces in A (for control individual traces with urIPSC responses (gray) and their average (red), for APV only average (dark gray)). (D) Left: Summary of effects of APV on average normalized urIPSC amplitude (n = 10 MCs). Diamonds indicate the experiments with no detectable response in the presence of the drug. Right: Comparison of delta urIPSC integrals between control vs in the presence of APV (n = 10 MCs). (E) Representative example of MC AP evoked by somatic current injection in control conditions (black trace) and in the presence of APV (gray trace) and added GBZ (20 µM; blue trace). Inset: Magnified afterhyperpolarizations (AHPs). (F) Left: Cumulative effects of APV on average normalized AHP amplitude (n = 18 MCs). Right: Effect of occlusion experiments on AHP amplitude (APV before GBZ or GBZ before APV, n = 12 MCs).
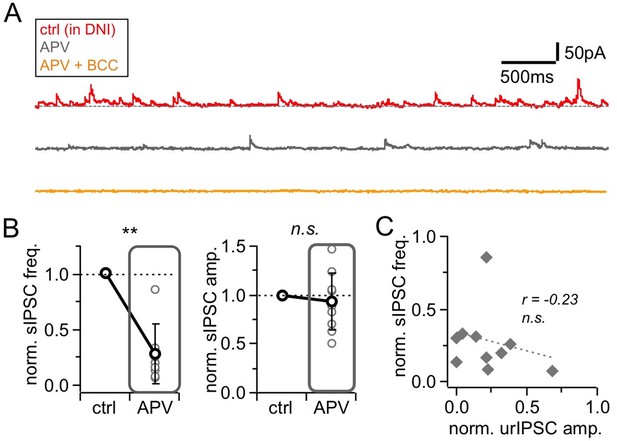
Effects of NMDAR blockade by D-APV (APV, 25 µM) on spontaneous activity.
(A) Representative experiment showing voltage-clamp recordings (+10 mV) from a mitral cell in the presence of DNI (control, top trace), upon addition of APV (middle trace) and further wash-in of BCC (50 µM; bottom trace). (B) sIPSCs in control and in presence of APV (n = 10 MCs). Left: Frequency. Right: Amplitude. sIPSCs were abolished in BCC (analysis not shown) (C) Linear regression between the APV effect on sIPSC frequency vs urIPSC amplitude (n = 10 MCs). No significant correlation.
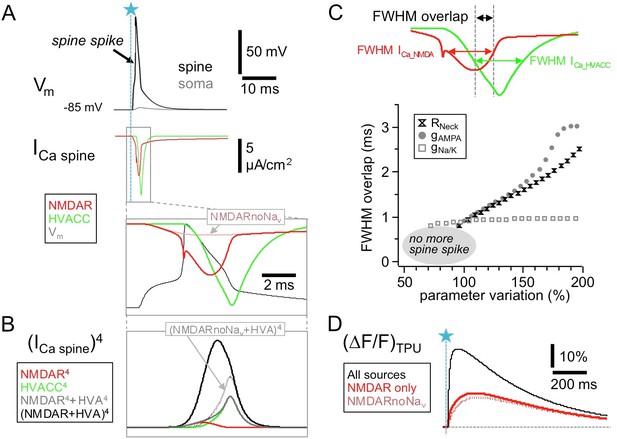
HVACC- and NMDAR-mediated Ca2+-currents overlap temporally in the wake of the spine spike.
Simulated spine Ca2+-currents and (ΔF/F)TPU. (A) Top panels: spine membrane potential Vm and Ca2+-currents (parameters exactly as in Aghvami et al., 2019, their Figure 2B, except for removed exogenous Ca2+ buffer). Bottom: Blow-up of NMDAR- and HVACC-mediated Ca2+-currents, overlaid with the membrane potential Vm. Note the early onset of the NMDAR Ca2+-current and its increase during the underlying local action potential, with a notch caused by the reduction of driving force during the AP upstroke. Red dotted line: NMDAR Ca2+-current in the absence of Navs. (B) The temporal coincidence creates an 'overlap bonus’ with respect to local Ca2+ concentration and triggering of release: Fourth power of single and added Ca2+-currents. Black dotted line: Addition of HVACC with NMDAR Ca2+-current in the absence of Navs (purely hypothetical: pretending that HVACC was not affected, only the NMDAR Ca2+-current). (C) Robustness test for extent of overlap between NMDAR- and HVACC-mediated Ca2+-current. Overlap is measured as the stretch of overlapping FWHMs of the current transients (Full Width Half Maxima, see Materials and methods). Parameter variation within 50–200% of the nominal value of the neck resistance Rneck, the AMPAR conductance gAMPA and the coupled Nav/Kv conductance gNa/K. Results are shown only for parameter runs with spine spike. (D) Simulated fluorescence transients ∆F/F (in the presence of 100 µM OGB-1) show that the contribution of the Nav-mediated boost of NMDAR Ca2+-currents to the total NMDAR-mediated Ca2+ signal is almost negligible (see Discussion).
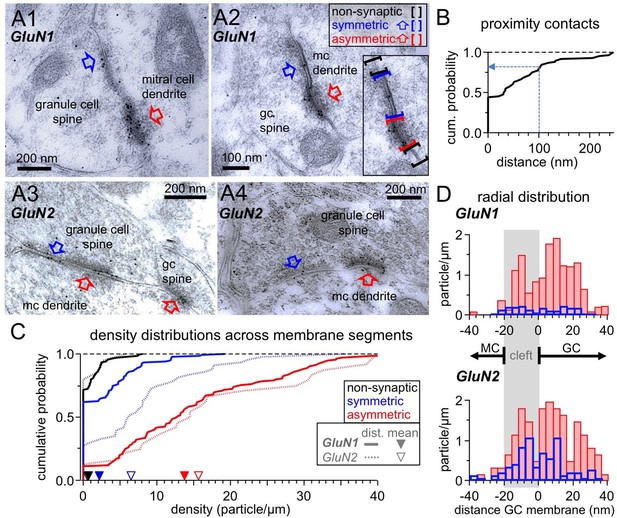
Ultrastructural analysis of the distribution of NMDARs shows their presence at both post- and pre-synaptic GC spine membranes in reciprocal dendro-dendritic synapses.
(A) Representative micrographs of reciprocal dendrodendritic synapses labeled for GluN1 (A1, A2) and GluN2A/B (A3, A4). Note that asymmetric MC-to-GC synapses (red arrows) are strongly labeled and that symmetric GC-to-MC junctions (blue arrows) are also immunopositive. Gold particles are usually not associated with non-synaptic plasma membrane domains of GC spines. The inset in panel A2 illustrates the selection of non-synaptic membrane segments (black brackets), symmetric synapses (blue) and asymmetric synapses (red). (B) Frequency distribution of distances between symmetric and asymmetric synaptic profiles on the same GC spine membrane (based on n = 60 micrographs with both types of profiles on the same spine). (C) Frequency distributions of labeling densities across synaptic (symmetric and asymmetric) and non-synaptic membrane segments for GluN1 (thick lines, n = 120, 111, 138 segments, respectively) and GluN2A/B (thin dotted lines, n = 46, 25, 24 segments, respectively). The arrows on the x-axis indicate the mean density (GluN1: solid, GluN2A/B: open). Color code as in (A). (D) Radial distribution of gold particles representing GluN1 (top panel) and GluN2A/B (bottom panel) at asymmetric (red) and symmetric (blue) synapses. Particle numbers were normalized to the total length of the respective synaptic segments. Absolute particle numbers see Results.
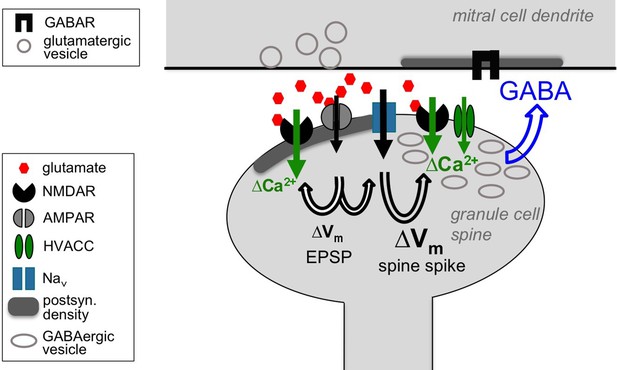
Cooperative release of GABA from the granule cell reciprocal spine.
Depolarizing currents are indicated by black solid arrows and Ca2+ entry by green solid arrows. Upon release of glutamate from the mitral cell dendrite, AMPARs get activated, leading to depolarization of the spine, then activation of both post- and presynaptic NMDARs and Navs. The latter in turn drive further depolarization, resulting in the spine spike, which both activates HVACCs and enhances Ca2+ entry via NMDARs by additional relief of their Mg2+ block. HVACC- and presynaptic NMDAR-mediated Ca2+-currents cooperate to promote recurrent release of GABA onto the mitral cell dendrite.