Mixed synapses reconcile violations of the size principle in zebrafish spinal cord
Figures
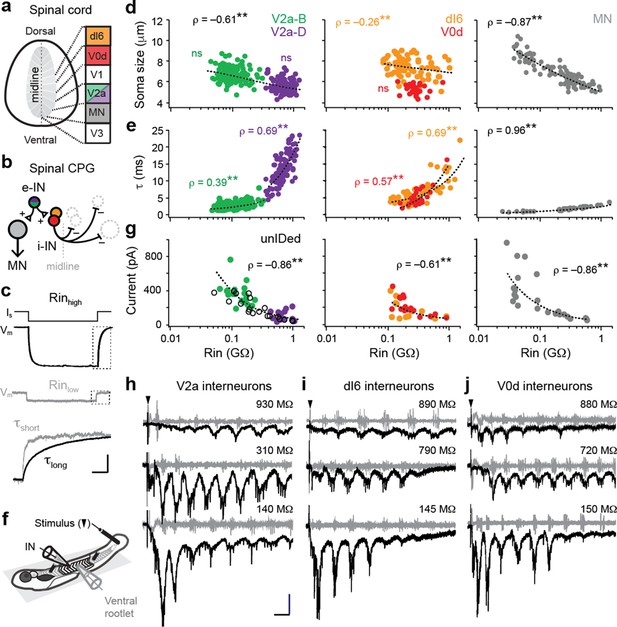
Size-independent scaling of time constants and excitation with interneuron input resistance.
(a) Cross-section of spinal cord denoting canonical classes of molecularly defined locomotor-related interneurons. (b) Wiring diagram of swimming circuitry comprised of excitatory interneurons (e-IN) that provide local excitation (+) of motor neurons (MNs) and inhibitory (i-IN) interneurons, which cross the midline and silence neurons on the opposite side (–). Corresponding interneuron classes color coded as in Figure 1a. (c) Whole-cell current clamp recordings illustrate current step (Is) and membrane potential deflections (Vm) in high and low input resistance (R) dI6 interneurons. Expanded traces below from boxed regions are normalized to illustrate differences in time constant (τ) related to resistance. Scale bar, 20 pA, 10 mV, 100 ms (top), and 10 ms (expanded). (d) Quantification of soma size versus input resistance for motor neurons and interneurons. Significant correlations are fit with logarithmic trendlines for illustrative purposes. ns, not significant. **, significant correlation following non-parametric Spearman Rank test. V2a-D=V2a neurons with descending axons; V2a-B=V2a neurons with bifurcating axons. V2a-B, ρ(143)=–0.08, p=0.326, n=145; V2a-D, ρ(103)=–0.14, p=0.219, n=105; dI6, ρ(70)=–0.26, p<0.05, n=72; V0d, ρ(26)=–0.09, p=0.641, n=28; MN, ρ(104)=–0.87, p<0.001, n=106. Source data are reported in Figure 1—source data 1. (e) Quantification of membrane time constant versus input resistance. V2a-B, ρ(152)=0.39, p<0.001, n=154; V2a-D, ρ(103)=0.69, p<0.001, n=105; dI6, ρ(68)=0.69, p<0.001, n=70; V0d, ρ(26)=0.57, p<0.001, n=28; MN, ρ(39)=0.96, p<0.001, n=41. Source data are reported in Figure 1—source data 1. (f) Schematic of the recording set up for ‘fictive’ swimming, evoked by a brief electrical stimulus to the tail skin (black triangle), with simultaneous recordings of interneuron (IN) activity and motor output from the ventral rootlet in chemically-immobilized larvae (see Materials and methods for details). (g) Quantification of peak inward excitatory currents versus input resistance during ‘fictive’ swimming.; V2a-B, ρ(22)=–0.58, p<0.01, n=24; V2a-D, ρ(12)=–0.11, p=0.702, n=14; V2a-unIDed, ρ(17)=–0.92, p<0.001, n=19; V2a-pooled, ρ(55)=–0.86, p<0.001, n=57; dI6, ρ(11)=–0.44, p=0.133, n=13; V0d, ρ(15)=–0.74, p<0.001, n=17; dI6-V0d-pooled, ρ(28)=–0.61, p<0.001, n=30. Figure 1g has been adapted from Figure 2i from Kishore et al., 2014, distributed under the terms of a Creative Commons Attribution-Noncommercial-Share Alike 3.0 Unported License CC BY-NC-SA 3.0 (https://creativecommons.org/licenses/by-nc-sa/3.0/). It is not covered by the CC-BY 4.0 license and further reproduction of this panel would need to follow the terms of the CC BY-NC-SA 3.0 license. Source data are reported in Figure 1—source data 1. (h) Whole-cell voltage-clamp recordings of V2a interneurons at calculated chloride ion reversal potential (–65 mV) with simultaneous ventral rootlet recordings (gray) reveal rhythmic inward excitatory currents (black) driving ‘fictive’ swimming after a brief electrical stimulus to the skin (at black arrow). Input resistance values accompany respective traces. Scale bar, 50 pA, 25 ms. (i) As in (h) but for dI6 interneurons. (j) As in (h) but for V0d interneurons.
© 2014, Kishore et al. All Rights Reserved. Figure 2i from Kishore et al., 2014, distributed under the terms of a Creative Commons Attribution-Noncommercial-Share Alike 3.0 Unported License CC BY-NC-SA 3.0 (https://creativecommons.org/licenses/by-nc-sa/3.0/). It is not covered by the CC-BY 4.0 license and further reproduction of this panel would need to follow the terms of the CC BY-NC-SA 3.0 license.
-
Figure 1—source data 1
Source data from panels d, e and g in Figure 1.
- https://cdn.elifesciences.org/articles/64063/elife-64063-fig1-data1-v1.xlsx
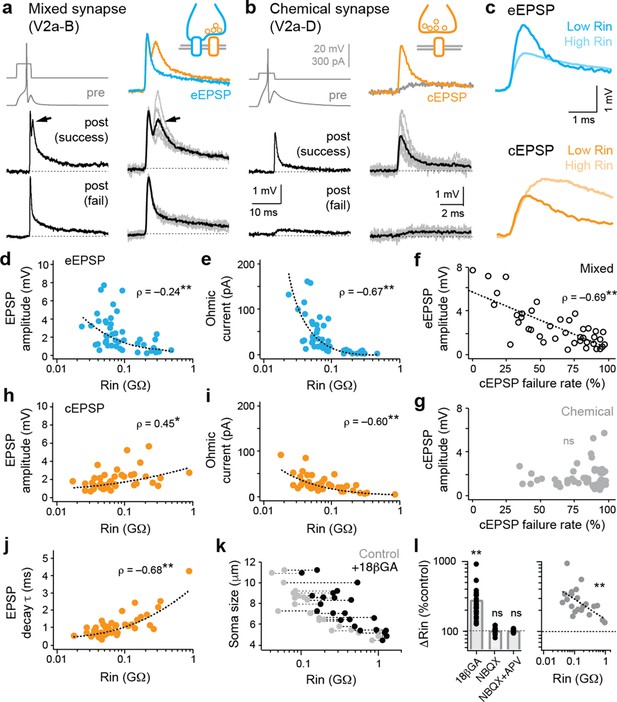
Electrical and chemical postsynaptic potentials are differentially scaled to input resistance.
(a) Example of a mixed synaptic connection from a V2a-B neuron on slower (left) and faster (right) time scales. A brief current pulse (pre, gray traces at top left) evoked a mixed synaptic response in a postsynaptic neuron (post, black traces at bottom left). Mixed synapses are characterized by an earlier electrical component and a later, less reliable chemical component prone to failures (at arrows; fail). Averages of two distinct components of mixed synapses (electrical, blue; chemical, orange) are illustrated top right. Averages in black illustrated bottom right are superimposed on individual sweeps in gray. (b) As in panel (a), but an example of a chemical connection arising from a V2a-D neuron. Note a slow excitatory postsynaptic potential that arises via indirect electrical coupling can be resolved when the chemical connection fails. Here, the chemical connection (orange) is superimposed on the slower postsynaptic potential (gray) observed during failures. (c) Superimposed data illustrate differences in the amplitude and time course of electrical (blue, eEPSP) and chemical (orange, cEPSP) excitatory postsynaptic potentials related to input resistance (Rin). (d) Quantification of electrical EPSP amplitude versus input resistance. **, significant correlation following non-parametric Spearman rank test (ρ(82)=–0.24, p<0.05, n=84). Source data are reported in Figure 2—source data 1. (e) Quantification of ‘ohmic current’ calculated from eEPSP amplitude and Rin (ρ(82)=–0.67, p<0.001, n=84). Source data are reported in Figure 2—source data 1. (f) Quantification of the failure rate of the chemical component versus the amplitude of the electrical component of mixed eEPSPs (black open circles). **, significant following non-parametric Spearman rank test (ρ(38)=–0.69, p<0.001, n=40). Source data are reported in Figure 2—source data 1. (g) As in (f), but for purely chemical synapse amplitude (ns; ρ(45)=0.08, p=0.599, n=47). Source data are reported in Figure 2—source data 1. (h) Quantification of chemical EPSP amplitude versus input resistance. **, significant correlation following non-parametric Spearman rank test (ρ(41)=0.45, p<0.01, n=43). Source data are reported in Figure 2—source data 1. (i), Quantification of ‘ohmic current’ calculated from cEPSP amplitude and Rin (ρ(41)=–0.60, p<0.001, n=43). Source data are reported in Figure 2—source data 1. (j) Quantification of chemical EPSP decay times. **, significant correlation following non-parametric Spearman rank test (ρ(41)=0.68, p<0.001, n=43). Source data are reported in Figure 2—source data 1. (k) Quantification of soma size versus Rin before (gray) and after (black) 18βGA application, illustrating the increase in resistance values regardless of size. Dotted lines link the same neurons (n=11 motor neurons, 12 interneurons). Source data are reported in Figure 2—source data 1. (l) Left, quantification of input resistance change expressed as a percent of controls in the presence of the gap junction blocker 18βGA versus glutamatergic blockers NBQX and/or APV. **, significant difference following non-parametric Mann-Whitney U-test (18βGA; U(22)=0, p<0.001, n=23). ns, not significant (NBQX; U(13)=84, p=0.520, n=14; NBQX+APV; U(4)=10, p=0.602, n=5). Right, quantification of the percentage increase in input resistance in the presence of 18βGA as a function of initial input resistance. **, significant correlation (ρ(21)=–0.61, p<0.01, n=23). Source data are reported in Figure 2—source data 1.
-
Figure 2—source data 1
Source data for panels d-l in Figure 2.
- https://cdn.elifesciences.org/articles/64063/elife-64063-fig2-data1-v1.xlsx
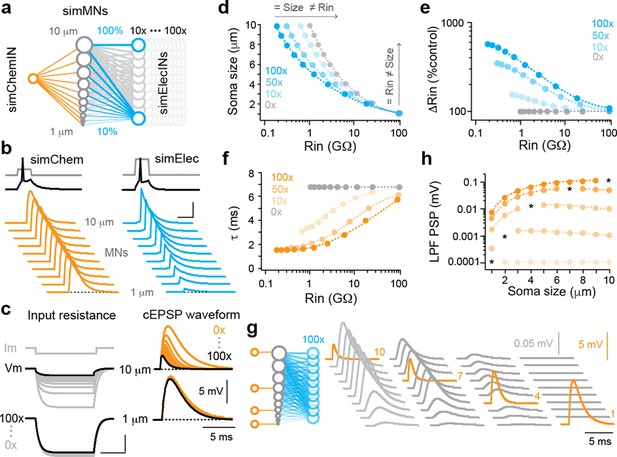
Simulations of convergent electrical and chemical synapses recapitulate experimental results.
(a) Schematic of the simulated network. A simulated interneuron forming chemical synapses (simChemIN, orange) with 10 simulated motor neuron (sim MNs, gray) somata ranging from 1 to 10 µm in diameter. Simulated interneurons forming electrical synapses (simElecINs, blue) can vary in number from 10 to 100, with a convergence ratio ranging from 10% to 100% based on size (i.e., the largest MNs receive 100% of inputs, while the smallest receive 10%). (b) Simulated chemical and electrical PSPs evoked by a single spike in the respective interneurons illustrate similarities (chemical) or differences (electrical) in amplitude related to size and excitability. Note increased current pulse amplitude in the simulated electrical interneuron due to a larger size and more electrical coupling lowering resistance. Scale bar, 20 pA, 45 mV (spikes), 5 mV (PSPs), and 5 ms. (c) Left, simulated current injection demonstrates the impact of increasing electrical synapse convergence (from 0x to 100x) on input resistance. Scale bars, 10 pA, 2.5 mV (top), 250 mV (bottom), and 20 ms. Right, simulated chemical PSPs illustrate the impact of increased convergence on amplitude and time course. (d) Quantification of relationship between soma size and input resistance with increasing electrical synapse convergence (0x, 10x, 50x, and 100x). Arrows indicate axes illustrating how the same sized neurons can have different resistances or the neurons with the same resistances can be different sizes. Source data is reported in are reported in Figure 3—source data 1. (e) Quantification of the relationship between changes in Rin relative to control values with increasing electrical synapse convergence. Source data are reported in Figure 3—source data 1. (f) Quantification of the relationship between membrane time constant (t) and input resistance with increasing electrical synapse convergence. Source data are reported in Figure 3—source data 1. (g) Schematic on left illustrates that individual motor neurons receive input from only one simulated chemical interneuron, while electrical synapse convergence is at maximum value (100x). Simulated chemical PSPs and the resulting coupling potentials are illustrated to the right. (h) Quantification of the amplitude of the low-pass filtered (LFP) coupling postsynaptic potential versus input neuron (marked by asterisks), as in (g). Source data are reported in Figure 3—source data 1.
-
Figure 3—source data 1
Source data for panels d, e, f, and h in Figure 3.
- https://cdn.elifesciences.org/articles/64063/elife-64063-fig3-data1-v1.xlsx