Characterization and functional analysis of cathelicidin-MH, a novel frog-derived peptide with anti-septicemic properties
Figures
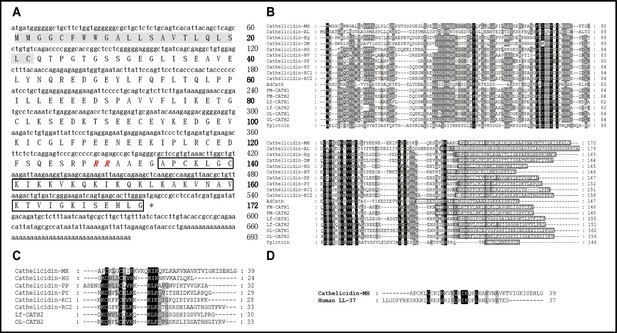
Sequence characterization of cath-MH.
(A) The cDNA and deduced amino acid sequence of cath-MH. The signal peptide is emphasized in gray, and the RR residues in red italic indicate the end of an acidic spacer domain. The sequence of mature peptide is boxed, and the stop codon is denoted by an asterisk (*). Nucleotide and amino acid numbers are displayed after the sequences. Multisequence alignment of cathelicidins from (B) Amphibians or (C) frogs. In order to maximize structural similarity, residue deletions denoted by the hyphens (-) have been introduced in some sequences and the identical residues are indicated in black. The highly conserved residues are shaded. The domains of putative mature peptides are boxed. (D) Sequence alignment of cath-MH and LL-37.
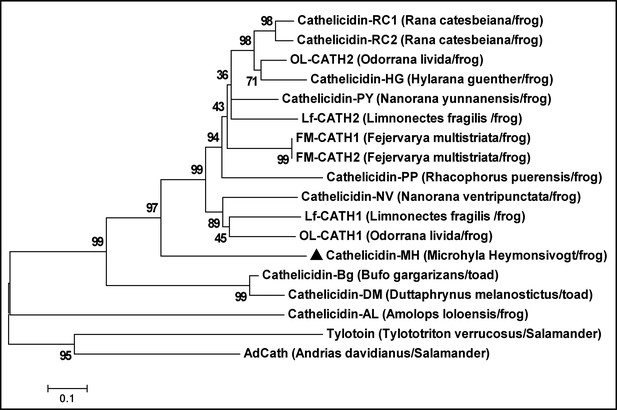
Evolutionary relationships of cath-MH and other amphibian cathelicidins.
The tree was constructed using the neighbor-joining method according to the amino acid sequences of cathelicidin identified from amphibians. The numbers on the branches indicate the percent bootstrap support. Cath-MH is represented by a black triangle.
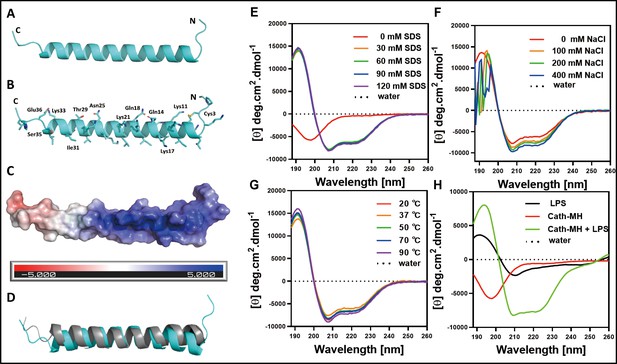
The secondary structure of cath-MH.
(A) Cartoon representation of the cath-MH structure with labeled N and C termini. (B) Cartoon visualization of residues participating in the stabilization of the helix. Amino acid side chains are shown as sticks. (C) Electrostatic potential of the monomeric structure of cath-MH mapped on the molecular surface. The negative surface potential is colored red while the positive one is colored blue. (D) Cartoon representation of superposition of the cath-MH (shown in cyan) and LL-37 (PDB ID: 5NMN; shown in gray) structures. CD spectra of 0.213 mg/ml cath-MH dissolved in the indicated concentrations of SDS solutions (E) or in 60 mM SDS plus the indicated concentrations of NaCl (F) or in 60 mM SDS at 20, 50, 70, and 90°C for 1 hr (G) or, in 5 mM HEPES plus 50 μM LPS (H) (n = 3 per group).
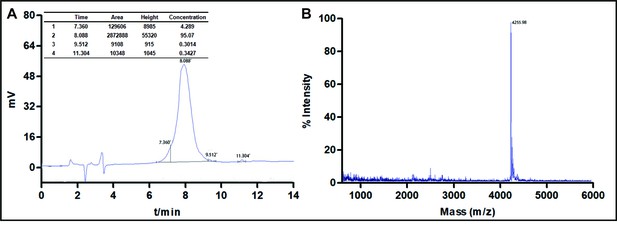
The synthesis and characterization of cath-MH.
(A) RP-HPLC. (B) MALDI-TOF-MS.
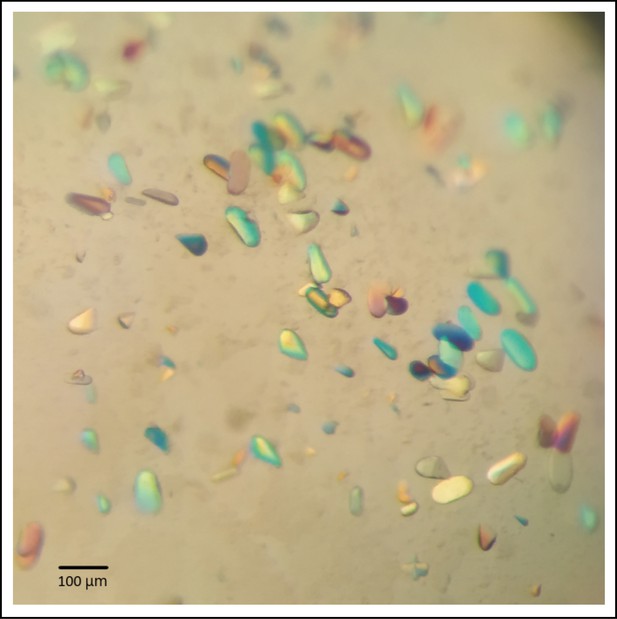
The cath-MH crystals grown from 2 M ammonium sulfate used for diffraction analysis.
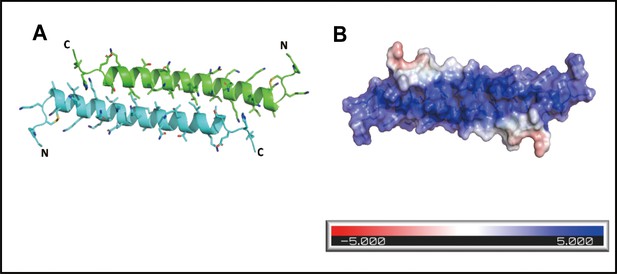
Visualization of the cath-MH dimeric structure.
(A) Cartoon representation of the dimeric peptide structure with side chains shown as sticks. (B) Electrostatic potential of the dimeric structure of cath-MH mapped on the molecular surface: negative surface potential is colored red, positive surface potential is shown in blue.
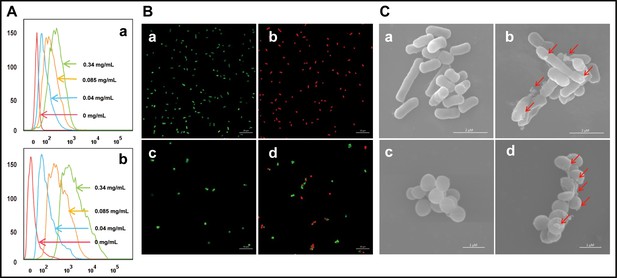
Effect of cath-MH on bacterial cell membranes.
(A) Flow cytometry analysis of interaction between FITC-labeled cath-MH and E. coli ATCC 25922 (a) or S. aureus ATCC 25923 (b). (B) Confocal laser scanning microscopy of control E. coli ATCC 25922 (a), S. aureus ATCC 25923 (c), and cath-MH-treated E. coli ATCC 25922 (b), S. aureus ATCC 25923 (d). Cath-MH is at concentration of 1× MIC. Live and dead cells stained by SYTO9 and PI are presented as green and red fluorescence, respectively. Scale bars: 10 μm. (C) Scanning electron microscopy of control E. coli ATCC 25922 (a), S. aureus ATCC 25923 (c), and cath-MH-treated E. coli ATCC 25922 (b), S. aureus ATCC 25923 (d). Red arrows indicate membrane defects. Scale bars: 2 μm in E. coli ATCC 25922; 1 μm in S. aureus ATCC 25923 (n = 3 per group).
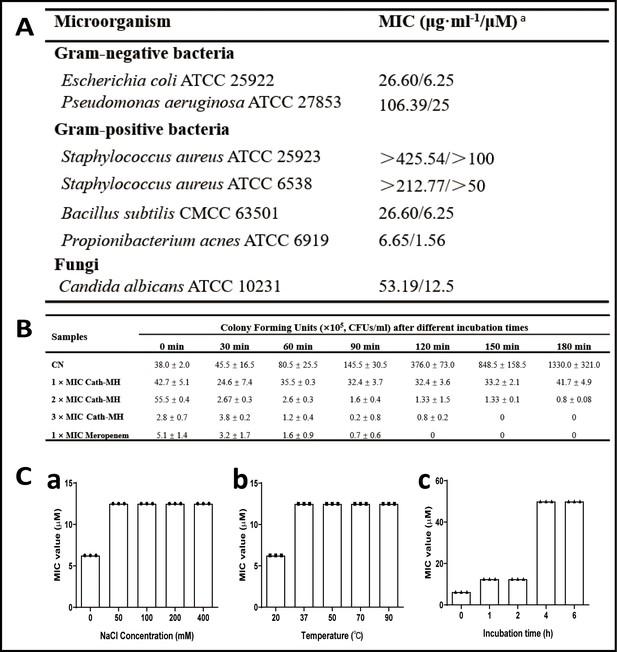
Antimicrobial activity of cath-MH.
(A) MICs of cath-MH against microbes. a MIC: minimal inhibitory concentration. These concentrations represent mean values of three independent experiments performed in duplicates. (B) Bacteria killing kinetics of cath-MH against E. coli ATCC 25922 (n = 3 per group). E. coli ATCC 25922 was mixed with cath-MH at the indicated concentrations for 0, 30, 60, 90, 120, 150, and 180 min, respectively. The MICs of cath-MH and meropenem against E. coli ATCC 25922 are 26.6 and 11.5 μg/ml. Data represent mean ± SEM values from three independent experiments. (C) Stability analysis of cath-MH under different conditions. The MICs of cath-MH incubated in different concentrations of NaCl at room temperature for 1 hr (panel a), at different temperatures for 1 hr (panel b), or with human serum at a ratio of 1:4 (v/v) at 37°C for 0 - 6 hr (panel c) (n = 3 per group).
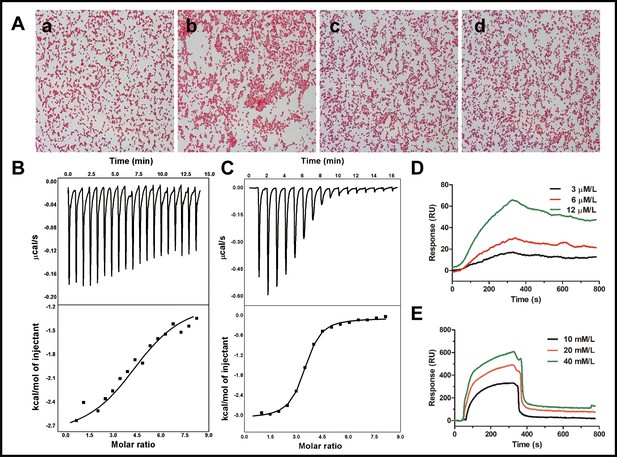
Binding reaction of cath-MH with LPS and d-(+)-galacturonic acid.
(A) Agglutination of E. coli ATCC 25922 treated with cath-MH. E. coli ATCC 25922 at a density of 1 × 109 CFU/ml were treated with BSA (a) and 1× MIC of cath-MH (b); 1× MIC of cath-MH plus an equal volume of 1 mg/ml LPS (c); or 4 mM d-(+)-galacturonic acid (d) for 30 min at room temperature before being Gram stained (migration: 1000×). ITC analysis of cath-MH binding to (B) LPS and (C) d-(+)-galacturonic acid. The top panels show thermo changes of each injection at different time points, while the bottom panels show changes in enthalpy as a function of ligand/target molar ratio. Data were fitted using a single-site binding model using MicroCal Origin software. SPRi analysis of (D) LPS and (E) d-(+)-galacturonic acid binding to cath-MH immobilized on a gold chip (n = 2 per group).
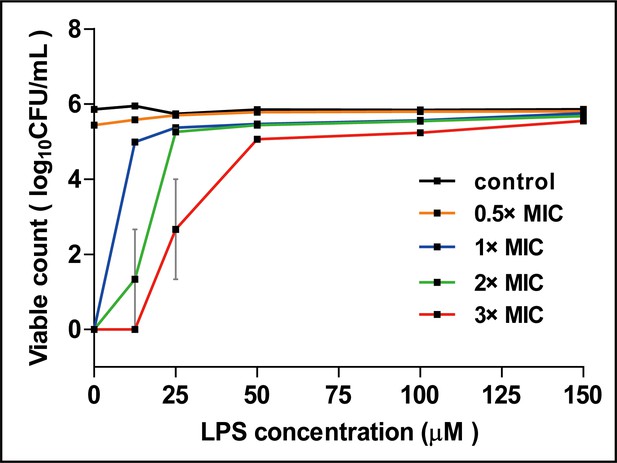
The effect of LPS on the antimicrobial activity of cath-MH against B. subtilis CMCC 63501.
0.5 ×, 1 ×, 2 ×, and 3 × MICs of cath-MH were pre-mixed with the indicated concentrations of LPS (from Escherichia coli O55:B5) and incubated for 30 min before the mixtures were separately added to exponential phase 106 CFU/ml B. subtilis CMCC 63501 in LB broth. After incubation at 37°C for 60 min, colony forming units were counted. Data shown are mean ± SEM (n = 3 per group).
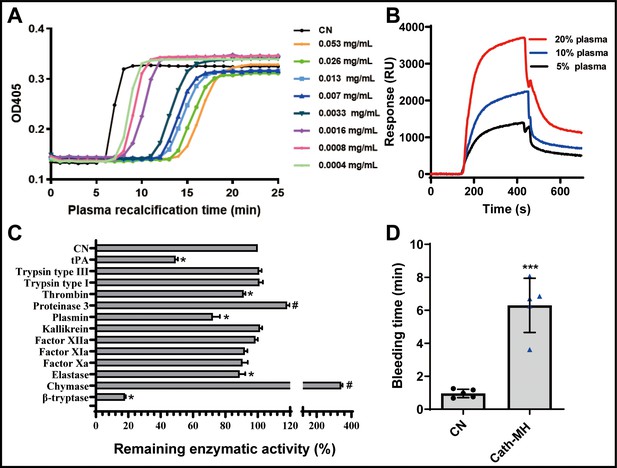
Effects of cath-MH on plasma recalcification time (PRT) and enzymatic activity.
(A) Effect of cath-MH on normal human PRT. The indicated concentrations of cath-MH in 50 μl of 0.15 M NaCl were incubated with 50 μl of normal human platelet-free plasma and 50 μl of 25 mM CaCl2. Data are reported as mean ± SEM (n = 3 per group). (B) Representative SPRi sensograms of human plasma binding to cath-MH immobilized on a gold chip. (C) Inhibitory activity cath-MH against specific proteases. 0.004 mg/ml cath-MH was examined against 13 different proteases in triplicate. Enzyme concentrations are described in Supplementary file 5. Bars indicate the mean remaining enzymatic activity in the presence of cath-MH. #p<0.05 and *p<0.05, significantly different compared to control group without cath-MH. (D) Effect of cath-MH on bleeding time in vivo (2 mm segment of the tail). CN: control. Data are reported as mean ± SEM (n = 3 per group). ***p<0.001, significantly different compared to control group without cath-MH.
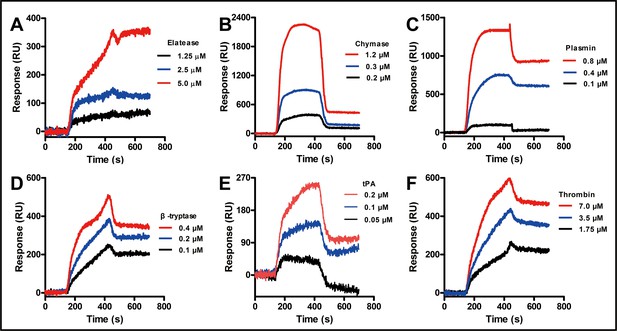
Representative SPRi sensograms of cath-MH immobilized in a gold chip binding to the indicated different proteases.
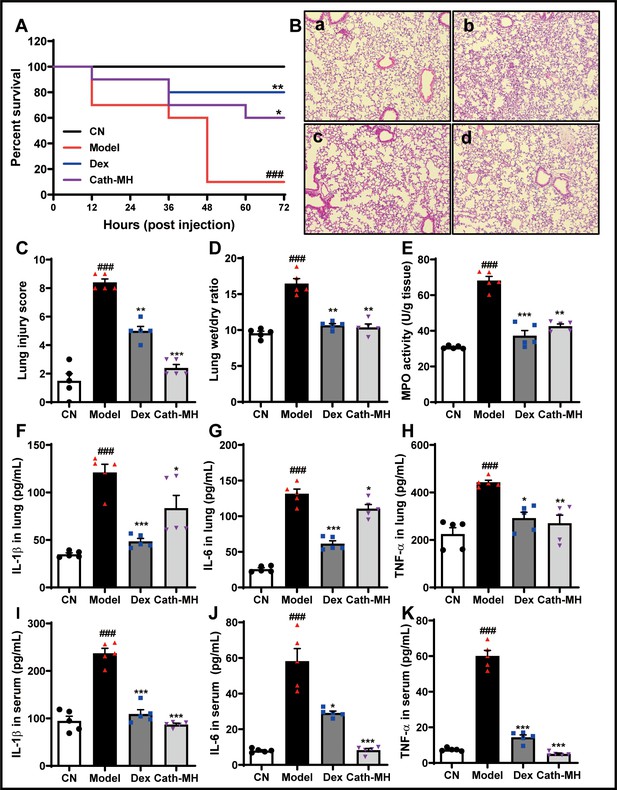
Effects of cath-MH on LPS-induced septic mice.
Mice except for those in the control group were i.p. challenged with 25 mg/kg LPS 1 hr before injection of saline, cath-MH (5 mg/kg), or dexamethasone (10 mg/kg) (n = 10 mice per group). Survival was observed at the indicated time point after LPS challenge and is expressed as Kaplan–Meier survival curves. Tissues were stained with hematoxylin and eosin, and pro-inflammatory cytokines were measured by ELISA 12 hr after LPS challenge. (A) Effects of cath-MH on the survival rates of LPS-induced mice. (B) Lung histology after 12 hr of LPS challenge in control (a), model (b), dexamethasone-treated (c), and cath-MH-treated (d) mice (magnification ×100). (C) Lung injury scores. (D) Wet/dry ratio of lung tissues. (E) MPO activity of lung tissues. (F–H) Effects of cath-MH on pro-inflammatory cytokine protein expression in lung tissues of mice. (I–K) Effects of cath-MH on pro-inflammatory cytokine protein expression in serum. Data presented are mean ± SEM (n = 5 per group). CN: control, Dex: dexamethasone. ###p<0.001 significantly different compared to control group treated with saline only; *p<0.05, **p<0.01, ***p<0.001 compared to the model group.
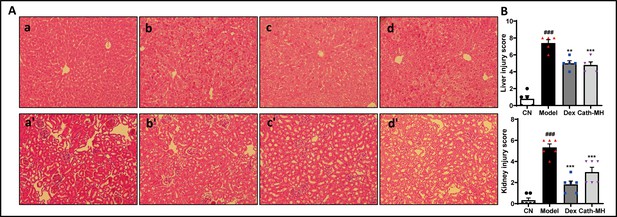
Effect of cath-MH on liver and kidney in LPS-induced septic mice.
(A) Histological images of liver and kidney after 12 hr of LPS challenge in control group (panel a, a’), model group (panel b, b’), dexamethasone-treated group (panel c, c’), and cath-MH-treated group (panel d, d’). Liver tissues: a – d ; kidney tissues: a’- d’. (B) The injury scores of liver and kidney tissues. Data presented are mean ± SEM (n = 3 per group). ### p < 0.001 significantly different compared to control group treated with saline only; **p < 0.01, *** p < 0.001 significantly different compared to model group.
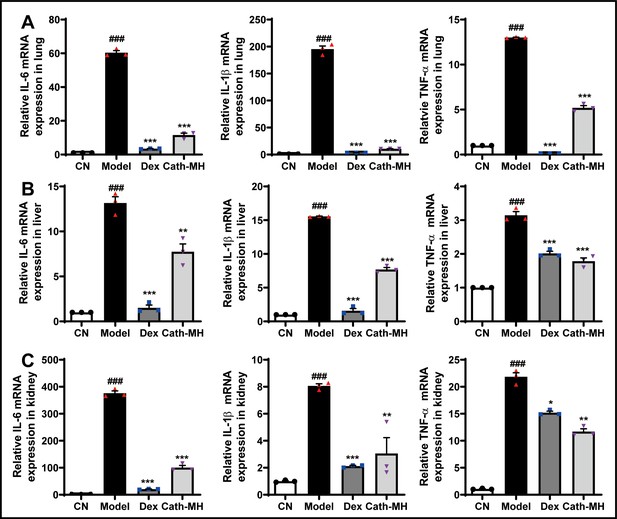
Effects of cath-MH on pro-inflammatory cytokine mRNA expression in different organs of LPS-induced septic mice.
Data presented are mean ± SEM (n = 3 per group). ###p < 0.001 compared to the control group; *p < 0.05, **p < 0.01, ***p < 0.001 compared to the model group.
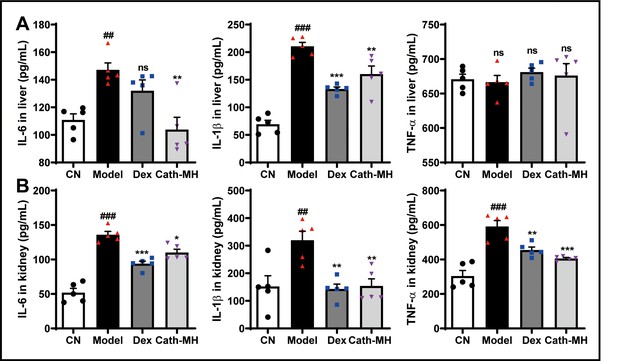
Effects of cath-MH on pro-inflammatory cytokine protein expression in livers and kidneys of LPS-induced septic mice.
Data presented are mean ± SEM (n = 3 per group). ##p < 0.01 and ###p < 0.001 compared to the control group; *p < 0.05, **p < 0.01, ***p < 0.001 compared to the model group.
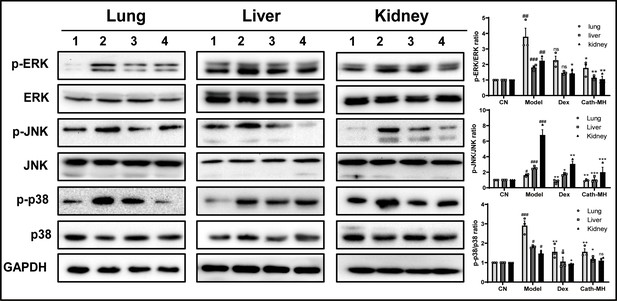
Effects of cath-MH on MAPK signaling in lung, liver, and kidney tissues.
Mice were euthanized 12 hr after LPS administration, and the tissue samples were prepared for western blotting (n = 3 per group). (A) Western blotting images of p38, ERK, and JNK. (B) Ratio of phosphorylated ERK, JNK, and p38 to total protein. Western blot images shown are representative of three independent experiments with similar results. Images were quantified using ImageJ software. The tissues in Lanes 1–4 are from the control, model, dexamethasone (10 mg/kg), and cath-MH group (5 mg/kg), respectively. Data presented are mean ± SEM (n = 3 per group). #p<0.05, ##p<0.01, ###p<0.001 significantly different compared to the control group; *p<0.05, **p<0.01, ***p<0.001 significantly different compared to the model group.
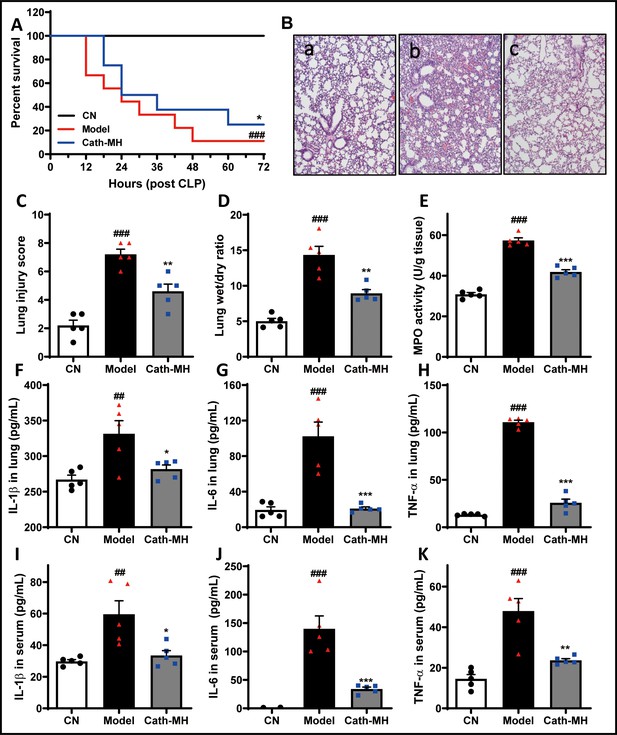
Effects of cath-MH in CLP-induced mice.
Survival at the indicated time points, pathological alterations, MPO activity, and cytokine expression at 24 hr after CLP were examined. (A) Effects of cath-MH on the survival rates of CLP-induced mice. (B) Representative histological images of lungs 24 hr after operation in control (a), model (b), and cath-MH-treated (c) mice (magnification ×100). (C) Lung injury scores of mice. (D) Wet/dry ratio of lung tissues. (E) MPO activity in lung tissues. (F–H) Effects of cath-MH on pro-inflammatory cytokine protein expression in lung tissues. (I–K) Effects of cath-MH on serum pro-inflammatory cytokine protein expression. Data presented are mean ± SEM (n = 5 per group). ##p<0.01, ###p<0.001 significantly different compared to sham control group; *p<0.05, **p<0.01, ***p<0.001 compared to the model group.
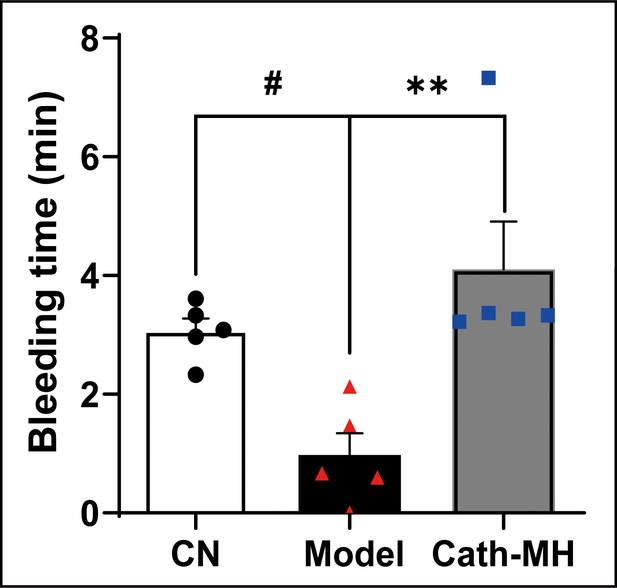
Effect of cath-MH on bleeding time in CLP-induced septic mice.
(5 mm segment of the tail) (n = 5 per group). #P< 0.05 significantly different compared to sham control group; **p < 0.01 compared to the model group.
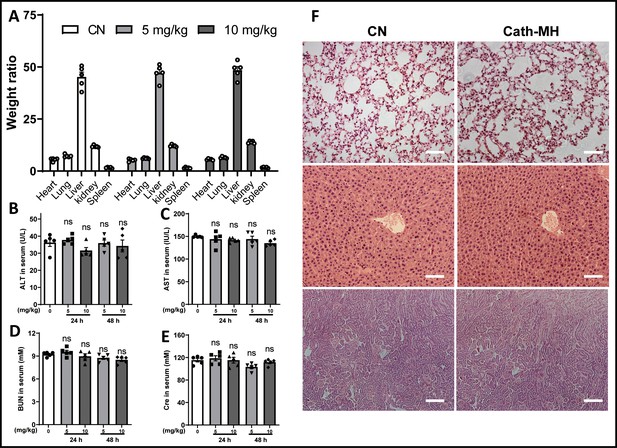
Toxicity of cath-MH in vivo.
Normal mice were injected i.p with cath-MH (5 mg/kg or 10 mg/kg) or saline, and sacrificed for biochemical index detection at 24 hr and 48 hr post injection. (A) Organ body weight ratio after 48 hr treatment; (B-E) The level of ALT, AST, BUN and Cr in serum after 24 hr and 48 hr treatment; (F) Histopathological observation of mice tissues at 48 hr. Tissues of lung (top), liver (middle) and kidney (bottom) were stained with hematoxylin-eosin, and observed and recorded under light microscope (200×) (n = 5 per group).
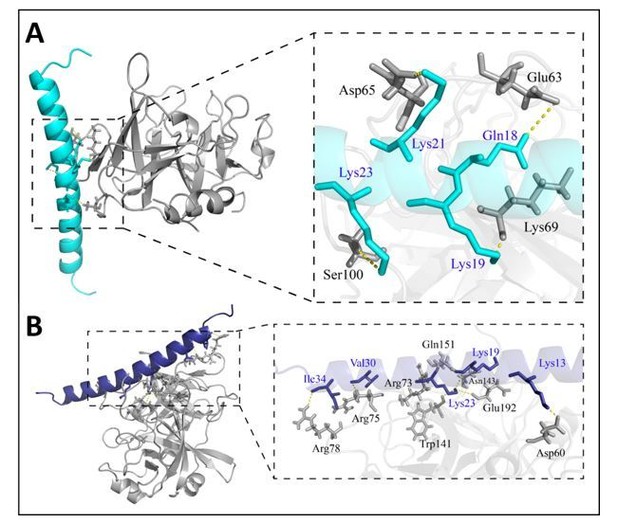
Model comparison of Heymonin (cath-MH) bound to chymase (A) and thrombin (B).
Molecular dynamics simulations were performed to examine the binding interactions of Heymonin (cath-MH) using models generated from the existing X-ray crystal structures of chymase (PDB ID 4AG1) and thrombin (PDB ID 4UD9). The model of Heymonin (cath-MH) (cyans or blue) bound to chymase (A. gray) or thrombin (B. gray) is shown on the left, with the enzyme shown in ribbon representation and Heymonin (cath-MH) shown in stick model (CPK coloring, cyans or blue ) on the right.
Tables
The structure and function of different cathelicidins.
Peptide | Origin | Amino acid sequence | #AA | Function | Ref. |
---|---|---|---|---|---|
Heymonin | Frog Microhyla heymonsivogt | APCKLGCKIKKVKQKIKQKLKAKVNAVKTVIGKISEHLG | 39 | antimicrobial activity; LPS neutralization; Sepsis therapeutic efficacy; Serine protease inhibitory activity | None |
cathelicidin-PY | frog Paa yunnanensis | RKCNFLCKLKEKLRTVITSHIDKVLRPQG | 29 | antimicrobial activity; LPS neutralization; anti-inflammatory | (Wei et al., 2013) |
human LL-37 | human | LLGDFFRKSKEKIGKEFKRIVQRIKDFLRNLVPRTES | 37 | antimicrobial activity; LPS neutralization; anti- inflammatory; wound healing and angiogenesis; Sepsis therapeutic efficacy; platelet activation induction | (Durr et al., 2006) |
Hc-CATH | sea snake Hydrophis cyanocinctus | KFFKRLLKSVRRAVKKFRKKPRLIGLSTLL | 30 | antimicrobial activity; LPS neutralization; anti-inflammatory | (Wei et al., 2005) |
chicken CATH-2 | Chicken | RFGRFLRKIRRFRPKVTITIQGSARG | 26 | antimicrobial activity; immunomodulatory | (Kraaij et al., 2020) |
cathelicidin-PP | tree frog Polypedates puerensis | ASENGKCNLLCLVKKKLRAVGNVIKTVVGKIA | 32 | antimicrobial activity; LPS neutralization; anti-inflammatory | (Mu L et al., 2017) |
cathelicidin-RC1 | Bullfrog Rana Catesbeiana | KKCKFFCKVKKKIKSIGFQIPIVSIPFK | 28 | antimicrobial activity | (Ling et al., 2014) |
Cathelicidin-RC2 | Bullfrog Rana Catesbeiana | KKCGFFCKLKNKLKSTGSRSNIAAGTHGGTFRV | 33 | weak antimicrobial activity | (Ling et al., 2014) |
Additional files
-
Supplementary file 1
Secondary structural components of cath-MH in different environments.
- https://cdn.elifesciences.org/articles/64411/elife-64411-supp1-v1.docx
-
Supplementary file 2
Conditions for growing cath-MH crystals used for X-ray diffraction.
- https://cdn.elifesciences.org/articles/64411/elife-64411-supp2-v1.docx
-
Supplementary file 3
Data collection and refinement statistics of X-ray diffraction.
- https://cdn.elifesciences.org/articles/64411/elife-64411-supp3-v1.docx
-
Supplementary file 4
Different thermodynamic parameters from ITC.
- https://cdn.elifesciences.org/articles/64411/elife-64411-supp4-v1.docx
-
Supplementary file 5
The buffers and the enzyme concentrations used in protease inhibition assays.
- https://cdn.elifesciences.org/articles/64411/elife-64411-supp5-v1.docx
-
Supplementary file 6
Primers (mouse) used for qRT-PCR.
- https://cdn.elifesciences.org/articles/64411/elife-64411-supp6-v1.docx
-
Transparent reporting form
- https://cdn.elifesciences.org/articles/64411/elife-64411-transrepform-v1.pdf