Dorsal periaqueductal gray ensembles represent approach and avoidance states
Figures
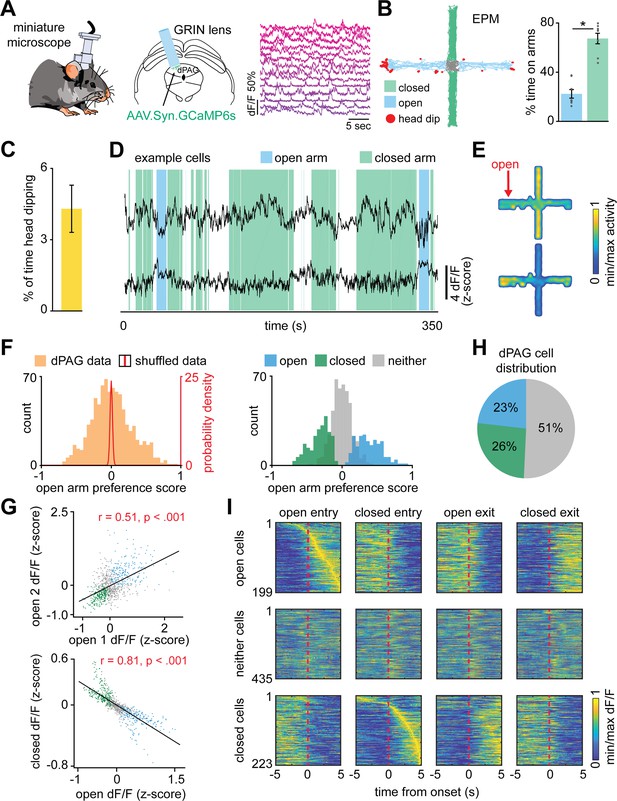
Dorsal periaqueductal gray (dPAG) neuronal ensembles encode arm type in the elevated plus maze (EPM).
(A) Gradient refractive index (GRIN) lens implantation, virus expression strategy, and example Ca2+ signals of neurons in the dorsal periaqueductal gray (dPAG). (B) Example mouse exploration path recorded in the EPM. Mice spent significantly more time in the closed arms compared to the open arms (data are represented as mean ± SEM; W = 0, p=0.012, Wilcoxon signed rank test, n = 8 mice). (C) Mean percentage of time in which mice engaged in head dips during the whole EPM session (n = 8 mice). (D) dPAG dF/F traces from the same mouse that display preferential activity in the closed (upper trace) and open (lower trace) arms of the EPM (open and closed arm-preferring cells). Epochs corresponding to exploration of the closed and open arms are shown respectively as green and blue shaded areas. (E) Activity heatmaps for corresponding example neurons shown in (D). (F) The open arm preference score was calculated for each neuron (orange bars; see Materials and methods), as was the distribution of open arm preference score for shuffled data (red line). Bars show the distribution of open arm preference score for open, closed, and neither cells (n = 857 cells). (G) Scatterplots showing correlations between neural activity across the two open arms (top) and between open and closed arms of the EPM (bottom). Each point represents one cell (n = 857 cells, r = Pearson’s correlation coefficient). (H) Pie chart shows the percent of all recorded neurons that were classified as open, closed, or neither cells (n = 857 cells). (I) For each subplot, each row depicts the mean normalized activity of an open, closed, or neither arm-preferring cell during behavior-aligned arm transitions (n = 857 cells).
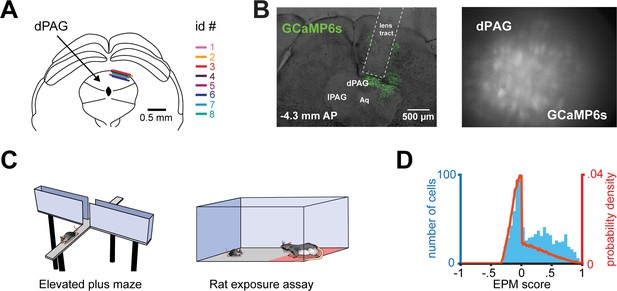
Deep brain imaging of dorsal periaqueductal gray (dPAG) neurons and distribution of elevated plus maze (EPM) scores.
(A) Illustration and sequence of EPM and rat exposure assays. (B) (Left) Coronal section of the periaqueductal gray showing GCaMP6s expression and representative gradient refractive index (GRIN) lens placement in the dPAG. Position of the section relative to bregma is indicated in the lower left corner. Aq: aqueduct (Sylvius). Scale bar: 500 μm. (Right) Maximum projection of the dPAG field of view in an example mouse. (Top right) Anatomical scheme of GRIN lens front location of animals expressing GCaMP6s under the control of the Syn promoter in large populations of dPAG neurons (n = 8 mice). (C) Behavioral tests. (D) The EPM score (blue) differs significantly from distribution expected by chance (red line) (n = 857 cells; U = 15.62, p<0.001, Wilcoxon rank sum test). The EPM score is near +1 for cells that fire similarly in arms of the same type. For example, a cell that had high activity in both open arms and very low rate on both closed arms would have a score near 1. A cell with high activity in both closed arms and low activity in both open arms also would have a score near 1. Thus, both open and closed arm-preferring cells would have positive scores. A cell active uniformly in the environment would have a score near 0. A cell that fired differently in arms of the same type would have negative score, such as a cell that has high activity in one open arm and lower than mean activity in the other open arm. Note that this is a different metric from the open arm preference score metric as shown in Figure 1F.
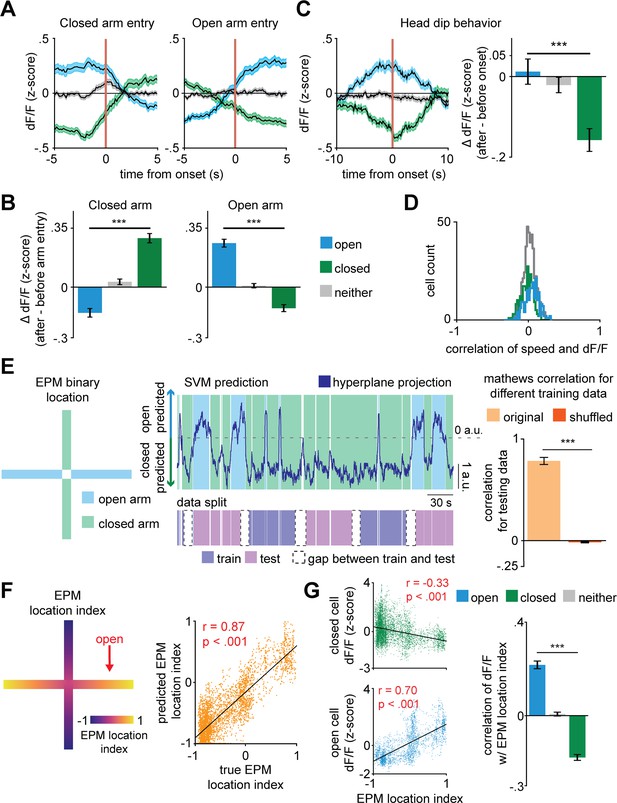
Dorsal periaqueductal gray (dPAG) population activity predicts elevated plus maze (EPM) exploration.
(A) Traces show the mean z-scored activity (±1 SEM) of all open, closed, and neither cells, behavior-aligned to arm transitions (respectively the blue, green, and gray traces). Cell categorization and calculated results were performed on non-overlapping training and testing data (n = 180 open cells, n = 513 neither cells, n = 164 closed cells). (B) Bars depict the change in z-scored dF/F for entries to closed left) and open (right) arms, separately for open, closed, and neither cells (data are represented as mean ± SEM; both closed and open arms; n of cells same as (A); closed arm U = 10.6, ***p<0.001, open arm U = 10.8, p<0.001, Wilcoxon rank sum test, n = 8 mice). (C) Average activity traces for open, closed, and neither cells relative to onset of head dips in the EPM and quantification of changes in activity for all cell types (0–2.5 s after minus 2.5–5.0 s before head dip onset) (data are represented as mean ± SEM; n of cells same as (A); U = 3.9, ***p<0.001, Wilcoxon rank sum test). (D) Histograms depict the distribution of the Pearson’s correlation of dF/F with speed for each cell type in the EPM (n of cells same as Figure 1I. (E) Prediction of arm-type mouse position in the EPM from neural data using a linear support vector machine (SVM). The blue and green areas represent the actual arm-type occupancy label (open and closed arm, respectively), and the black trace represents the prediction of arm location by the SVM hyperplane projection. If the trace was above 0 a.u., then that period was classified as open arm exploration, otherwise, it was classified as closed arm occupancy. The pink and purple represent the data split (training and testing data, respectively). The Matthews correlation coefficient for real and permuted shuffled training data are shown to the right (mean ±1 SEM; n = 8 mice, U = 4.9, p<0.001, Wilcoxon rank sum test). (F) (Left) Example of EPM location index where 1 and −1 correspond, respectively, to the extreme end of the open and closed arms and (right) prediction of labeled EPM location index from dPAG neural data for an example mouse (scatterplot displays testing data that was not used for training, r = Pearson’s correlation coefficient). (G) (Left) Correlation of example closed and open cell activity and (right) mean correlation of dF/F with EPM location index (data are represented as mean ± SEM; n of cells same as (A); U = 13.7, ***p<0.001, Wilcoxon rank sum test, r = Pearson’s correlation coefficient).
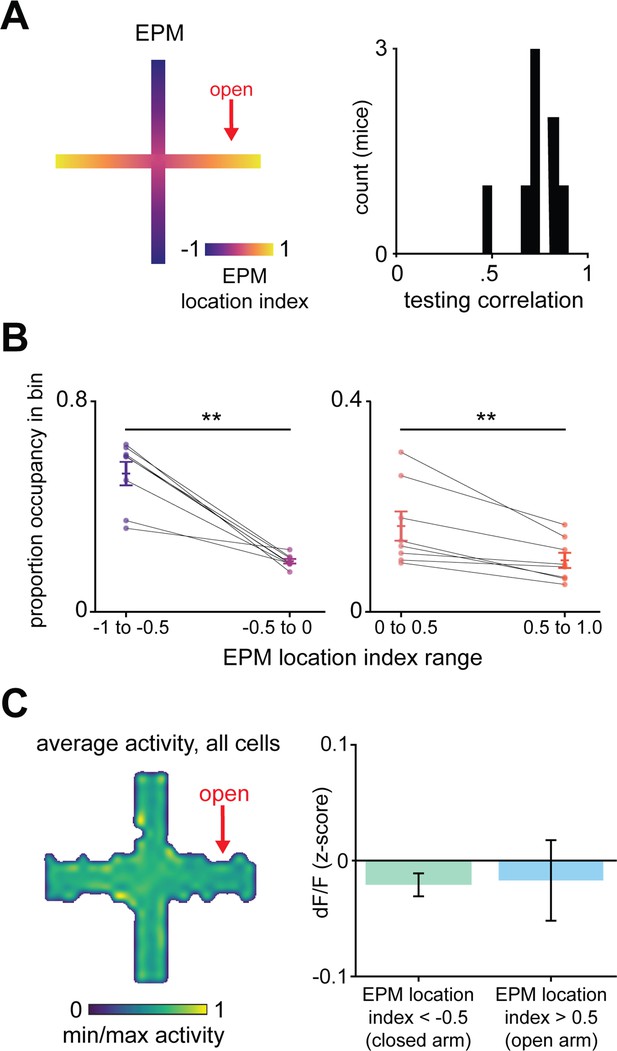
Validation of linear regression to predict elevated plus maze (EPM) location index using dorsal periaqueductal gray activity.
(A) Example of EPM location index where 1 and −1 correspond, respectively, to the extreme end of the open and closed arms (left). Histogram of testing correlations between labeled and predicted EPM location index for individual mice is significantly greater than 0 (right, t = 17.7, p<0.001, 7 d.o.f., one-sample t-test, n = 8 mice). (B) Mice spend more time in the extremes of closed arms than in less extreme locations of closed arms (left, W = 0, p=0.008, n = 8 mice) and less time in the extremes of open arms than in less extreme locations of open arms (right, W = 0, p=0.008, n = 8 mice). (C) Heatmap depicts the mean z-scored dF/F at each position of the EPM (left, n = 857 cells). Mean dF/F in extremes of EPM location index are not significantly different from each other (n = 8 mice, U = −0.11, p=0.92).
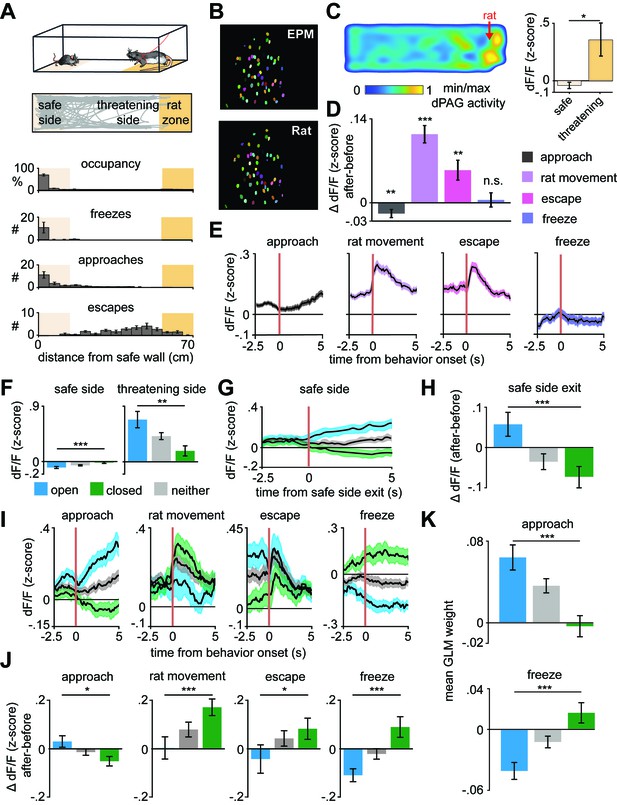
Arm-specific ensembles maintain functions across threatening situations.
(A) Illustration of the rat exposure assay (top) and example track (bottom), with labels depicting the area to which the rat is confined (rat zone) as well as areas near to and far from the rat (safe side). In all figures depicting this assay the rat area will be shown to the right. (B) Example imaging field of view with dorsal periaqueductal gray (dPAG) cells co-registered between elevated plus maze (EPM) and rat exposure sessions. (C) Heatmap depicts the mean z-scored dF/F at each position of the rat exposure assay (left, n = 713 cells). Mean dF/F is significantly greater in the threatening zone than in the safe zone (n = 713 cells, n = 7 mice, U = 2.24, p=0.03, Wilcoxon rank sum test). (D) Change in dF/F (0–2.5 s after minus 0–2.5 s before) activity for all dPAG cells for behaviors in the rat exposure assay (data are represented as mean ± SEM; n of cells for approach, rat movement, escape = 714, n of cells for freeze = 640; approach t = −2.65, **p=0.008, rat movement t = 7.87, ***p<0.001, escape t = 3.28, **p=0.001, freeze t = 0.39, p=0.69, one-sample t-test). (E) Traces show the mean z-scored activity of all cells (±1 SEM), aligned to onset of various behaviors (onset is indicated by the red vertical line) in the rat exposure assay (n of cells same as D). (F) Bars depict the mean z-scored dF/F of cells on the safe side and threatening side of the enclosure (data are represented as mean ± SEM; n = 64 open cells, n = 166 neither cells, n = 87 closed cells; n = 7 mice, safe U = −3.82, ***p=0.0001, threatening U = 3.05, **p=0.002, Wilcoxon rank sum test). (G) Traces show the mean z-scored activity of open, closed, and neither cells (±1 SEM), aligned to exit of the safe side of the enclosure (far from the rat). (H) Bars show the mean change in z-scored dF/F (0–2.5 s after minus 0–2.5 s before) aligned to safe side exit for open, closed, and neither cells (data are represented as mean ± SEM; n = 64 open cells, n = 166 neither cells, n = 87 closed cells; U = 3.29, ***p=0.001, Wilcoxon rank sum test). (I) Traces show the mean z-scored activity of open, closed, and neither cells (±1 SEM), aligned to behaviors in the rat exposure assay (approach, rat movement, escape: n = 64 open cells, n = 87 closed cells, n = 166 neither cells; freeze: n = 50 open cells, n = 67 closed cells, n = 132 neither cells). Onset of behaviors is indicated by a red vertical line. (J) Bars depict the change in z-scored dF/F (0–2.5 s after minus 0–2.5 s before) for behaviors in the rat exposure assay, separately for open, closed, and neither cells (data are represented as mean ± SEM; n of cells same as (I); approach U = 2.45, *p=0.014, rat movement U = −3.70, ***p=0.0002, escape U = −2.12, *p=0.034, freeze U = −3.62, ***p=0.0003, Wilcoxon rank sum test). (K) A generalized linear model (GLM) to predict single-cell activity was constructed using approach, escape, and freeze behaviors as variables. Bar plots show average GLM weights for approach and freeze for open, closed, and neither cells (data are represented as mean ± SEM; n = 62 open cells, n = 155 neither cells, n = 83 closed cells; approach U = 4.17, ***p<0.001, p=0.11, freeze U = 3.02, ***p<0.0002, Wilcoxon rank sum test).
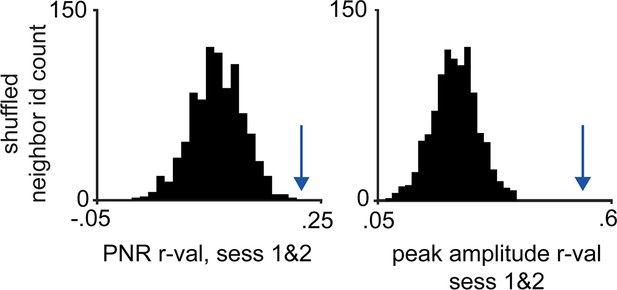
Validation of co-registration procedure.
The peak-to-noise ratio (PNR) and mean peak amplitude correlation values were calculated for co-registered cells between rat and elevated plus maze (EPM) assays. Cell identities were then shuffled within the 10 nearest neighbors 1000 times, and the same correlation measures were calculated for each iteration. The resulting distribution was compared to the actual PNR and mean peak amplitude values, indicated with arrow (for both left and right, n = 462; p<0.001, n = 7 mice).
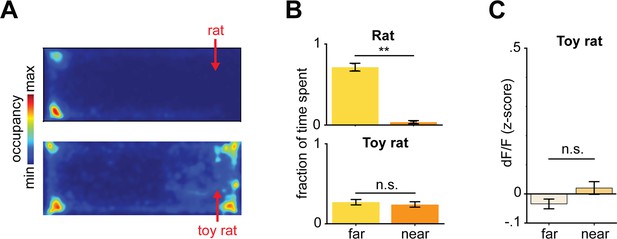
Behavioral and dorsal periaqueductal gray (dPAG) activity differences between rat and toy rat exposure.
(A) Heatmaps depicts the maximum and minimum spatial occupancy of mice during rat (top) and toy rat exposure (bottom). (B) Fraction of time spent near and far from rat (top, U = 3.13, p=0.0017, Wilcoxon rank sum test, n = 7 mice) and toy rat (bottom, U = 0.70, p>0.05, Wilcoxon rank sum test, n = 7 mice). (C) Average z-scored dPAG activity far and near to a toy rat (U = 1.85, p>0.05, Wilcoxon rank sum test, n = 7 mice).
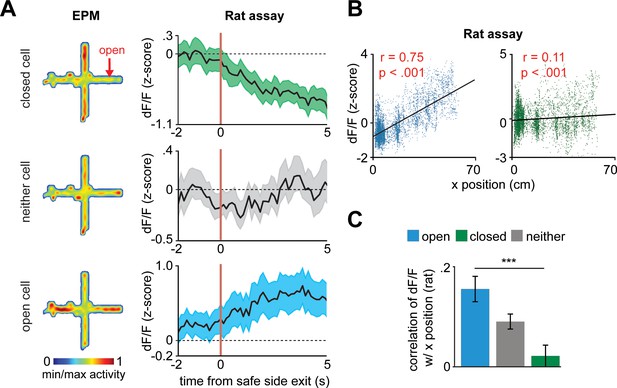
Correlation of dorsal periaqueductal gray cell ensembles with distance to a rat.
(A) Heatmaps show the normalized activity of example open, closed, and neither cells during the elevated plus maze (EPM) session (left). Traces show the normalized activity of the same cells when exiting the safe side of the rat assay and moving toward the rat (right). (B) Correlation of example open and closed cell activity with distance from the safe wall (rat exposure). Higher x position corresponds to locations more near the rat. (C) Mean correlation of dF/F with x position in the rat assay (data are represented as mean ± SEM; n = 64 open cells, n = 166 neither cells, n = 87 closed cells; U = 3.89, ***p<0.001, Wilcoxon rank sum test, n = 7 mice, r = Pearson’s correlation coefficient).
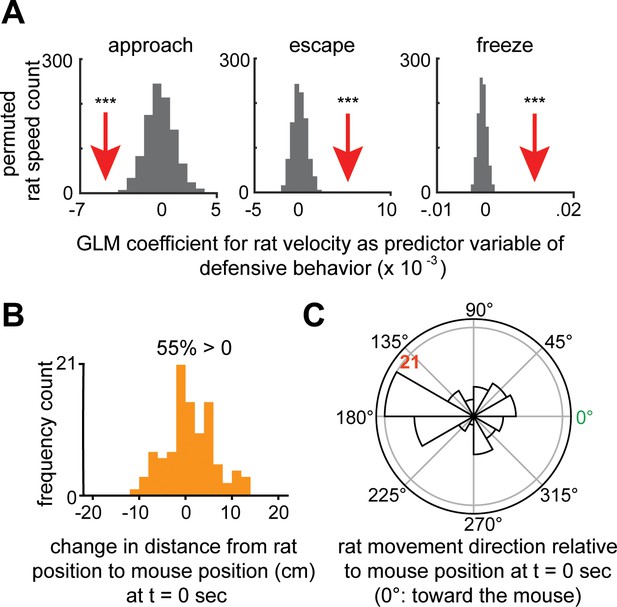
Increased rat velocity predicts lower approach to rat and higher threat avoidance-related behaviors such as escape and freeze.
(A) Separate generalized linear models (GLMs) were fit with rat velocity as the predictor variable and one of the binarized mouse behaviors: either approach (left), escape (middle), or freeze (right), as the response variable. The red arrow depicts the actual GLM coefficient for rat velocity, given each mouse behavior, while the histogram depicts the permuted distribution of rat velocity coefficient values for shuffled timepoints. Compared to this distribution, rat velocity shows a significantly negative coefficient for approach and significantly positive coefficients for escape and freeze. These data show that higher rat velocity predicts decreased occurrence of approach and increased frequency of escape and freezing (***p<0.001, n = 7 mice). (B) On average, rat movements do not decrease distance to the mouse’s position at the start of rat movement (measured 2 s after movement start). Negative values indicate movement toward the mouse (n = 100 occurrences). Fifty-five percent of rat movements increase distance from the mouse, indicating that rat movements are not consistently directed at the mouse which would be expected to decrease rat-mouse distance. (C) Rat movements are not consistently directed toward mice. Polar plot showing the distribution rat movement direction relative to mouse position at the start of rat movement initiation. Zero degrees represents movement toward the mouse, while 180 degrees corresponds to movements away from the mouse. Radius values = number of events.
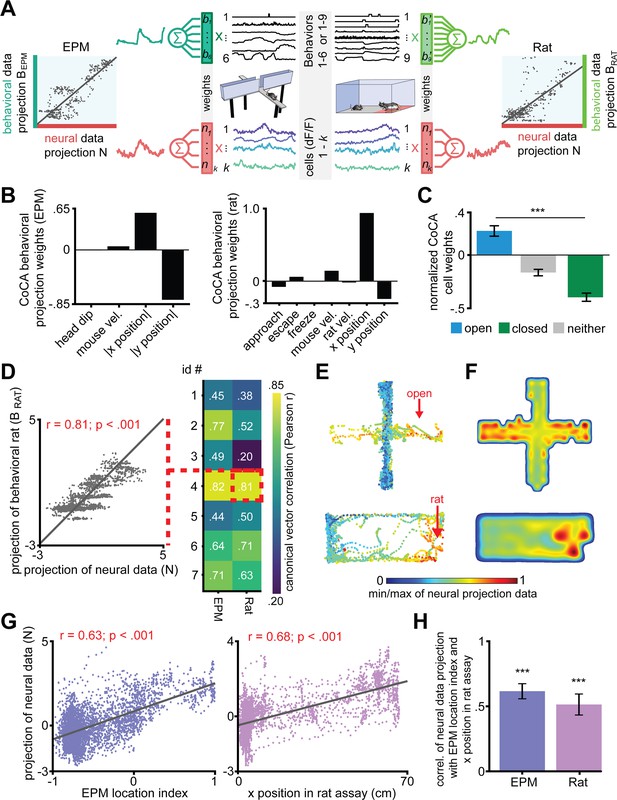
Dorsal periaqueductal gray (dPAG) displays a shared neural representation of risk imminence across the elevated plus maze (EPM) and rat exposure assays.
(A) Constrained correlation analysis (CoCA) reveals correlated encoding of behaviors and neural activity consistent across EPM and rat exposure assays. Linear projections of behaviors (top) correlate with projections of neural activity (bottom). Weights were optimized to maximize the correlation between neural and behavioral projections. Weight selection was constrained in the following way: weights for behavioral variable weights for each assay had to be conserved across mice, whereas neural projector weights had to be fixed across the EPM and rat assays for each cell. The behavioral variables used are listed in the x-axis of (B). Colors indicate consistent cells and projector weights. (B) Weights of CoCA behavioral projector variables for the EPM (top) and rat exposure (bottom) assays showing the relative importance of each variable in each assay. All variables were normalized to unit variance before training and testing, with the exception of |x| and |y|, which were scaled to the range [0, 1]. (C) CoCA neural projection weights normalized to the range [−1, 1], mean ± 1 SEM (data are represented as mean ± SEM; n = 64 open cells, n = 166 neither cells, n = 87 closed cells; U = 8.02, ***p<0.001, Wilcoxon rank sum test, n = 7 mice). (D) (Left) Example correlation of CoCA projection of behavioral data with projection of neural data for testing data (mouse 4, rat exposure). Each point is one timepoint of data. (Right) Correlation values of CoCA projection of behavioral data with projection of neural data for testing data for each mouse in each assay (p<0.05 all trials vs. random weights, see Materials and methods). (E) CoCA projection of neural data in the EPM (top) and rat exposure (bottom) assays for the same mouse. (F) Similar to (E), but as a heatmap using testing data from all mice for EPM (top) and rat exposure (bottom). (G) Projection of testing neural data is correlated with EPM location index (EPM, left) and x position (rat, right) for an example mouse (r = Pearson’s correlation coefficient). Larger EPM location index corresponds to locations more near the extreme end of the open arms. Larger x position values correspond to locations more near the rat. (H) Average correlation of projection of neural data with EPM location index and x position (rat assay) differs significantly from 0 (data are represented as mean ± SEM; n = 7 mice; EPM t = 10.80; rat t = 6.49, ***p<0.001, one-sample t-test).
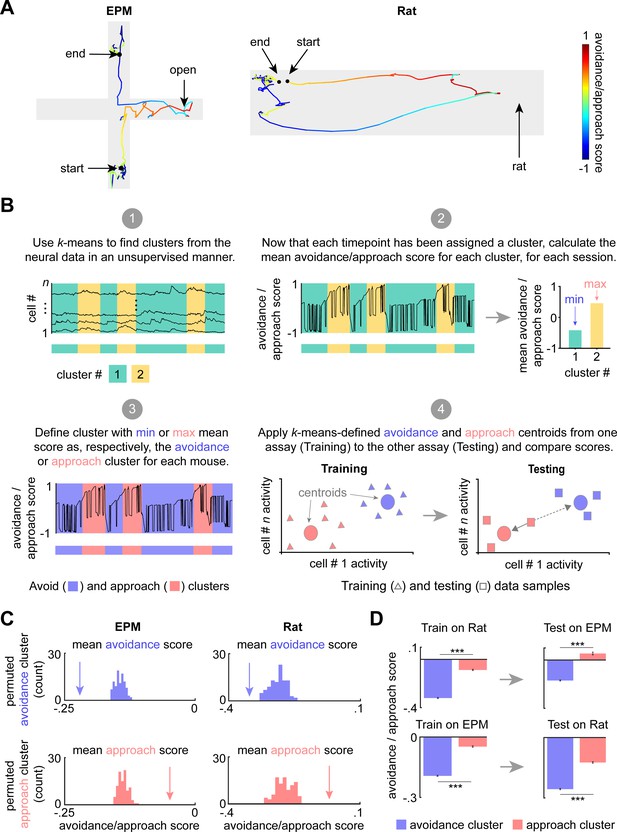
Dorsal periaqueductal gray (dPAG) displays a shared neural representation of avoidance and approach states across the elevated plus maze (EPM) and rat exposure assays.
(A) Example tracks in the EPM (left) and rat exposure assay (right), color-coded by avoidance/approach score (see Materials and methods). The approach score increased during movements toward the threat, reaching +1 when the animal reaches the end of the open arms or the rat. The score decreases during movement away from threat and reaches its minimum value of −1 when the mouse reaches the end of closed arms or the furthest point from the rat. This score was developed as a measure of avoidance/approach states. (B) Explanatory diagram depicting steps of the clustering analysis (see Materials and methods). (1) k-Means was used to find clusters in the neural data in an unsupervised manner. (2) The mean avoidance/approach score was calculated for each cluster defined in step 1. (3) The ‘avoidance’ and ‘approach’ clusters were identified as those with, respectively, the minimum or maximum mean avoidance/approach score calculated in step 2. (4) The approach and avoidance centroids defined in one assay were used to classify neural data from the other assay, based on the minimum Euclidean distance for each sample (as depicted by solid arrow). (C) Arrow depicts the experimentally observed mean avoidance/approach score for avoidance and approach clusters (EPM k = 2; rat k = 3, see Figure 4—figure supplement 1) across concatenated sessions (n = 7 mice). This mean was compared to a distribution of avoidance (top) or approach (bottom) cluster means, calculated by permuting the neural data 100 times (EPM cells n = 734, rat assay cells n = 713; for all, p<0.01). (D) (Left) Bars depict the mean rat and EPM avoidance/approach scores (±1 SEM) for approach and avoidance clusters across mice. (Right) As described in Materials and methods and Figure 4B, these cluster centroid locations, trained on one assay, were then used to define approach and avoidance timepoints in the other assay. Bars depict the corresponding mean avoidance/approach score (±1 SEM) for this testing data (train on EPM: avoidance cluster n = 31,938, approach cluster n = 22,630; test on rat: avoidance cluster n = 30,245, approach cluster n = 24,412; train on rat: avoidance cluster n = 14,658, approach cluster n = 10,514; test on EPM: avoidance cluster n = 15,319, approach cluster n = 2510 (n represents the number of timepoints, not cells); co-registered cells n = 317; Wilcoxon rank sum test, ***p<0.001).
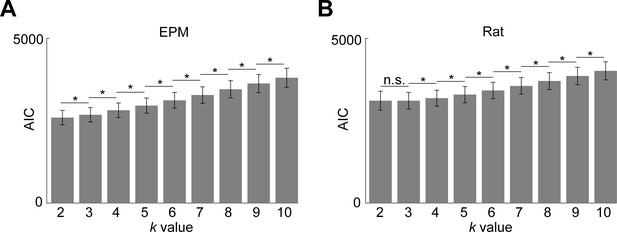
Determination of optimal number of clusters for elevated plus maze (EPM) and rat assay according to Akaike information criterion (AIC).
(A-B) Bars depict the AIC, a metric used to evaluate the degree to which a model fits the data. Lower AIC values indicate a better fit (comparisons between neighboring k values made by Wilcoxon signed-rank test; n = 7 mice). (A) For the EPM assay, a k-means model with k = 2 results in the lowest AIC value. (B) For the rat assay, a k-means model with either k = 2 or 3 results in the lowest AIC value. *p<0.05.
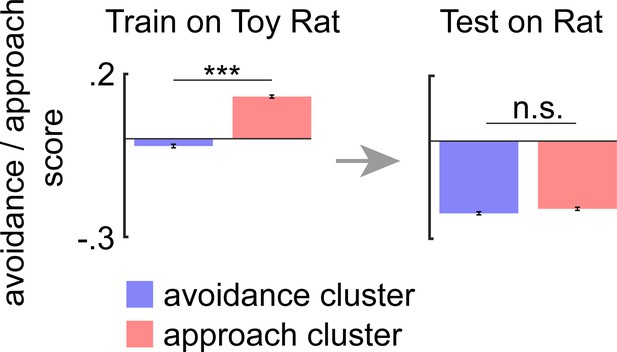
Dorsal periaqueductal gray (dPAG) ensembles do not encode avoidance and approach to a control toy rat.
(Left) Bars depict the mean rat exposure assay avoidance/approach scores (±1 SEM) for approach and avoidance clusters across mice. (Right) As described in Materials and methods and Figure 4B, these cluster centroid locations, identified using rat exposure assay data, were then used to define approach and avoidance timepoints in the toy rat assay. Bars depict the corresponding mean avoidance/approach score (±1 SEM) for this testing data (toy rat: avoidance cluster n = 12,844, approach cluster n = 15,888; rat exposure assay: avoidance cluster n = 14,662, approach cluster n = 13,570; co-registered cells n = 309; Wilcoxon rank sum test, ***p<0.001, n = 6 mice).
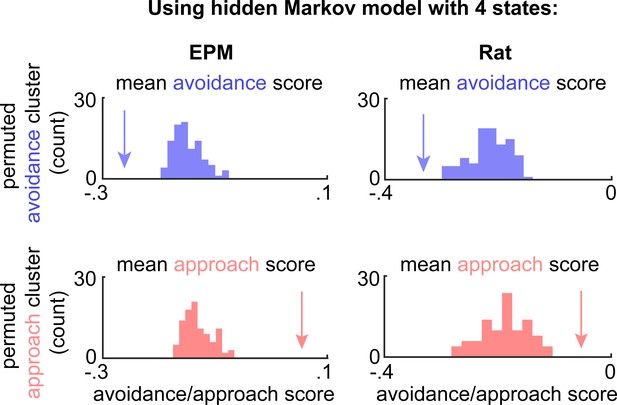
Hidden Markov models also differentiate avoidance and approach states.
Arrow depicts the experimentally observed mean avoidance/approach score for avoidance and approach states using a Hidden markov model (four states per assay; see Materials and methods) across concatenated sessions (n = 7 mice). This mean was compared to a distribution of avoidance (top) or approach (bottom) state means, calculated by permuting the neural data 100 times (elevated plus maze [EPM] cells n = 734, rat assay cells n = 713; for all, p<0.01).
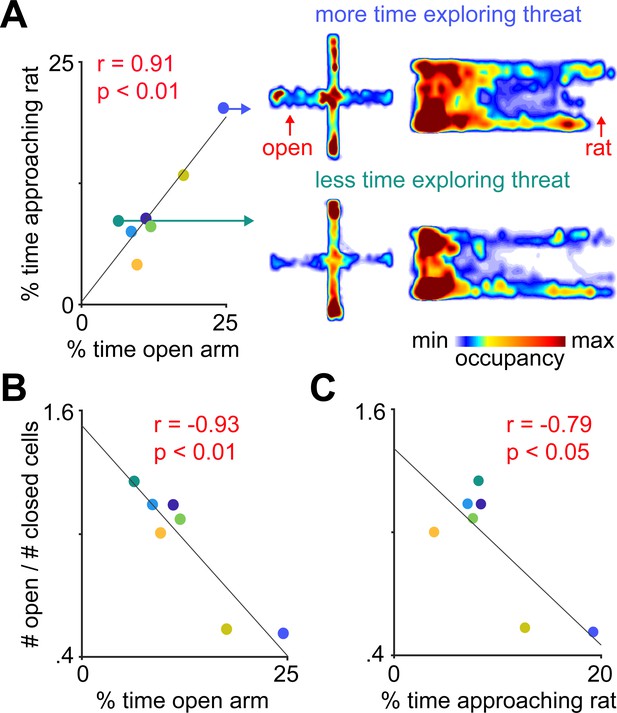
Fraction of open arm cells was negatively correlated with approach to threat across mice.
(A) Exploration of the open arms in the elevated plus maze (EPM) and approach to rat are correlated. Each point represents one mouse (n = 7 mice). Heatmaps show exploration in the EPM and the rat assay for example mice showing high (top traces) and low (bottom traces) levels of approach to the open arms and the area near the rat (both high threat areas are shown in red). (B-C) Mice with a higher fraction of open arm cells show lower exploration of the open arms in the EPM (B) and less time approaching the rat. (C) r = Pearson’s correlation coefficient.
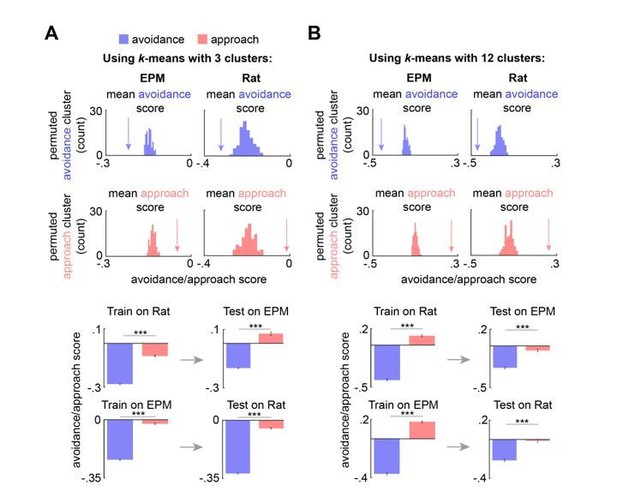
Approach and avoidance identified by k-means with a range of k values and Hidden Markov Model.
(A) (top) Arrow depicts the mean avoidance/approach score for avoidance and approach clusters (3 clusters), identified by k-means, across concatenated sessions (n = 7 mice). This mean was compared to a permuted distribution of avoidance/approach means, calculated by shuffling the neural data 100 times (n cells same as Figure 4C; p < 0.01). (bottom left) Bars depict the mean Rat and EPM avoidance/approach scores (+/- 1 s.e.m.) for approach and avoidance clusters across mice. (bottom right) As described in Methods and Figure 4B, these cluster centroid locations, trained on one assay, were then used to define approach and avoidance timepoints in the other assay. Bars depict the corresponding mean avoidance/approach score (+/- 1 s.e.m.) for this testing data. (Train on EPM: avoidance cluster n = 17753, approach cluster n= 13096; Test on Rat: avoidance cluster n = 15727, approach cluster n = 7724; Train on Rat: avoidance cluster n = 21092, approach cluster n = 10672; Test on EPM: avoidance cluster n = 9867, approach cluster n = 3028; n cells same as Figure 4D; Wilcoxon ranked-sum test) (B) Same as (A), but with 12 clusters. (Train on EPM: avoidance cluster n = 2614, approach cluster n= 3671; Test on Rat: avoidance cluster n = 1749, approach cluster n = 1488; Train on Rat: avoidance cluster n = 3693, approach cluster n = 2337; Test on EPM: avoidance cluster n = 2163, approach cluster n = 1323; n cells same as Figure 4D; Wilcoxon ranked-sum test) *** p < 0.001.

Rat movements are not consistently directed towards mice.
(A) Polar plot showing the distribution of direction of rat movement relative to mouse position at time = 0 seconds. (B) Distribution of direction of average rat movement across 2 seconds after rat movement initiation relative to mouse position at the start of rat movement initiation. Zero degrees represents movement toward initial mouse position, while 180 degrees corresponds to movements away from the mouse.
Tables
Reagent type (species) or resource | Designation | Source or reference | Identifiers | Additional information |
---|---|---|---|---|
Strain, strain background (Mus musculus) | C57BL/6J | Jackson Labs | RRID:IMSR_JAX:000664 | |
Strain, strain background (Rattus norvegicus) | Long-Evans | Charles River Labs | RRID:RGD-631593 | |
Recombinant DNA reagent | AAV9.Syn.GCaMP6s.WPRE.SV40 | Addgene | 100843-AAV9 | |
Software, algorithm | MATLAB | Mathworks | RRID:SCR_001622 |