Perturbation of amygdala-cortical projections reduces ensemble coherence of palatability coding in gustatory cortex
Figures
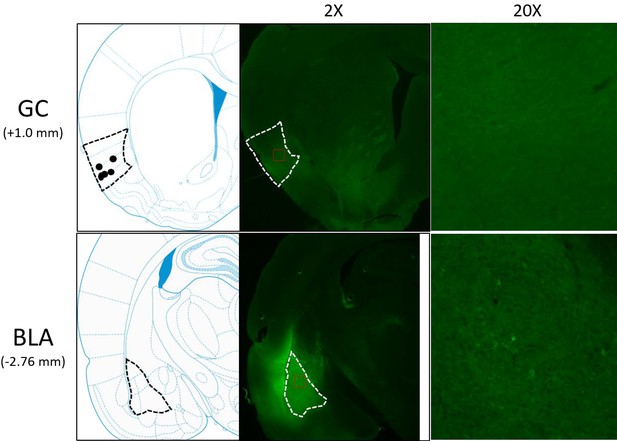
Schematics and sample histology showing ArchT virus infection, visualized by the GFP tag, in GC (top panel) and BLA (bottom panel).
Brain slices were taken from 1.0 mm anterior to bregma for GC and 2.76 mm posterior to Bregma for BLA. Black and white dashed lines outline GC and BLA in each panel, and ×20 images were the magnification of areas within red dashed lines in ×2 images. Solid circles in the schematics are final locations of the tips of opto-trodes (Schematics were modified from Paxinos and Watson, 2005 with permission will be requested from Elsevier upon the publication).
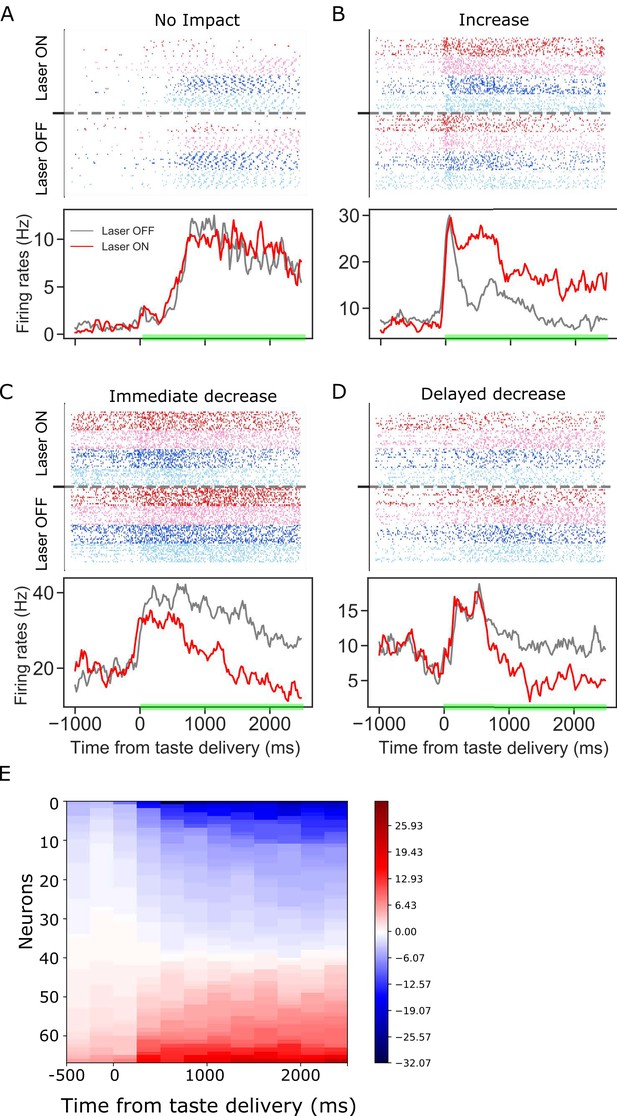
Panels A-D: GC taste responses with raster plots (top) and peri-stimulus histogram (bottom; averaged across all taste trials) during Laser-Off and Laser-On trials.
Each panel shows a representative GC neuron whose activity: (A) was not modulated, (B) increased, (C) immediately decreased, or (D) had a delayed decrease due to BLA→GCx. (E). Mean firing rate differences between control and perturbed trials across 2.5 s (x-axis, divided into ten 250 ms-bins) post-taste delivery. Each row in the y-axis is an individual neuron for which activity was significantly altered by BLA→GCx. Blue and red colors indicate the degree to which responses were decreased or increased by the perturbation, respectively. In each case, the perturbation either deceased or increased GC activity, but not both.
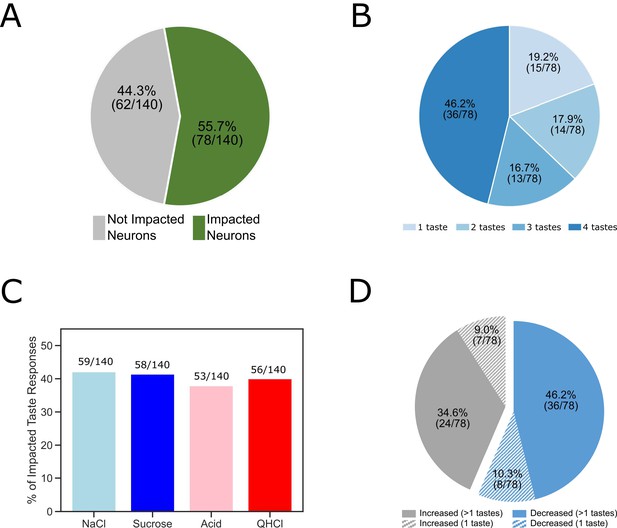
The overall impact of perturbation of BLA→GC axons on the entire GC population.
(A) Pie chart showing 55.7% (78/140) of recorded GC neurons were affected by laser stimulation. (B) The impacted GC neurons with various taste responses being influenced by laser stimulation. For 46.2% of the impacted neurons, responses to all four tastes were changed; 16.7% of the neurons showed changes to three (out of 4) tastes; for 17.9% of the GC neurons changed their responses to two tastes. Finally, for the rest of the neurons there was only one taste response altered by perturbation. (C) Bar graph showing the number of each individual taste response being altered by BLA→GCx. As revealed, the impact was comparable across tastes:~40% of each taste response was altered by stimulation. (D) Among the impacted neurons, 56.4% (44/78; blue) of them decreased their response rates in reaction to taste delivery while 43.6% (34/48; gray) increased response rates. Noted that in cases in which BLA→GCx impacted >1 taste response (60 [36 + 24] solid color), the perturbation either consistently decreased (60%; 36/60) or increased (40%; 24/60) firing rates.
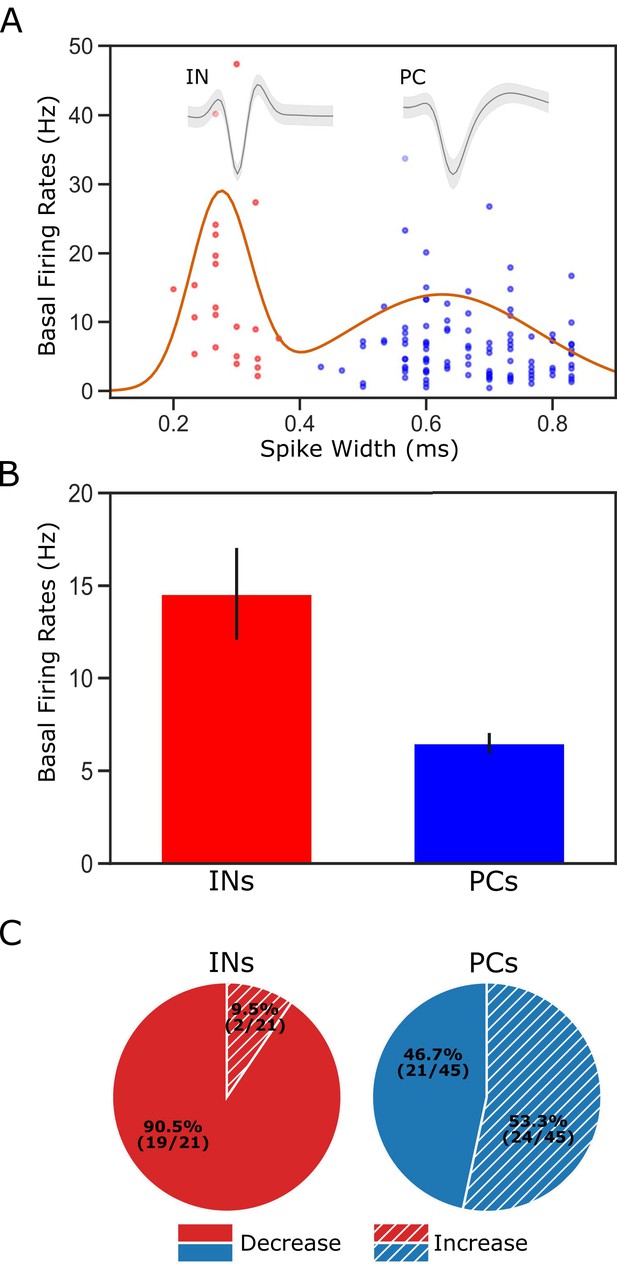
Classification of putative interneurons (INs) and pyramidal cells (PCs).
(A) Given the radical difference in waveforms between INs and PCs (representative examples of each type shown on the top of the figure), neuronal type assignment was based on the half-spike width (calculated from valley to the post-valley peak); units with width <0.35 ms are marked as INs (red dots) while those with width >0.35 ms are classified as PCs (blue dots). A Gaussian fit to the firing rates of the recorded neurons is overlaid with the data points. (B) GC single units classified as INs have higher basal firing rates than those classified as PCs. (C) The impact of perturbation of BLA→GC axons was different among the two types of GC neurons. While most of the GC INs (90.5%) were suppressed, only 46.7% of PCs showed decreased firing rates following laser stimulation.
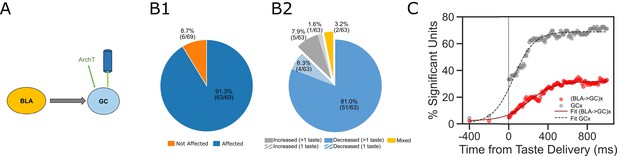
Comparisons between laser perturbation on GC somas and BLA→GC axons.
(A) GC recording was done ~4 weeks after ArchT AAV virus was infused into GC. (B) Pie charts demonstrate that laser stimulation caused firing changes in 91.3% of the recorded GC neurons (B1), among which, 87.3% showed suppressed impact while others were either enhanced or showed mixed impact by the perturbation (B2). Noted that both the percentage of affected neurons and the percentage of decreased units following direct perturbation of GC somas are significantly greater than those with perturbation on BLA→GC axons (Figure 3). (C) Percentages of GC neurons affected by perturbation of GC somas (grey dots) or BLA→GC axons (red dots). As indicated by fitted lines (of sigmoid function); the percentages of impacted GC neurons not only started higher immediately following laser stimulation but also rose at a faster rate than that was found with BLA→GCx.
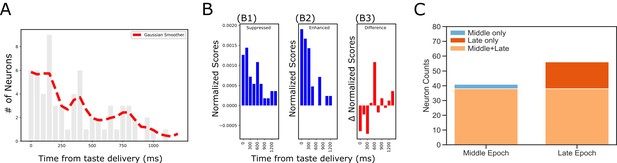
Dynamics revealed in analysis of impact latency and duration.
(A) Histogram of impact latencies occurring post-taste delivery. Instead of an exponential decay function, the distribution is best fitted with a mixture Gaussian function (red dash line) which found two peaks at ~300 ms and ~750 ms, respectively. (B) Distribution of impact latency grouped by decreased impact (B1) and increased impact (B2). B3 reveals the difference between decreased and increased impact, demonstrating a clear sign shift in the time bin started at 600 ms; after the shift, decreased impact became more dominant than increased ones. (C) Number of laser-impacted GC neurons grouped by whether they showed significant laser impact during the Middle ‘Identity’ epoch (100–600 ms) or the Late ‘Palatability’ epoch (700–1200 ms). As revealed, BLA→GCx rarely only impacted Middle epoch firing (n = 3). Instead, most of the affected neurons showed significant impact over the late, palatability epoch with the impact that either started when the Late epoch began (n = 15) or started earlier and remained impacted over the Late epoch (n = 38).
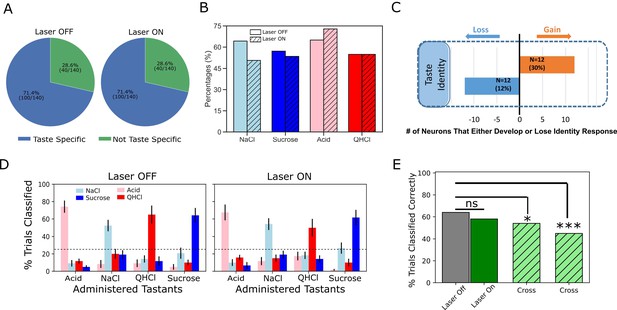
Laser perturbation of BLA→GC axons has little impact on GC taste specificity.
(A) The numbers of taste-specific neurons (71.4%) in Laser Off and Laser On trials are essentially identical. (B) The proportions of neurons responding to each taste (NaCl, Sucrose, Acid, and QHCl) are similar (and statistically non-distinct) for Laser-Off and Laser-On trials. (C) A within-neuron analysis reveals that similar numbers of neurons lost taste-specific activity and developed taste-specific activity anew with laser stimulation. (D) A classification analysis performed on the entire GC dataset shows that discriminability of taste spiking responses was similar in Laser-Off (left panel) and Laser-On (right panel) trials, with the classifier reliably picking out the administered taste at well above chance levels (dashed lines indicate 25% chance performance). (E) The overall percentage of trials in which tastes were correctly identified is similar for Laser-Off and Laser-On trials. When classification was evaluated across laser conditions (i.e. when the classifier was trained on control trials and tested on laser trials or vice versa), the percentage of trials in which the taste was correctly identified dropped significantly (‘Full dataset’; left hatched green bar); the rate of correct identification dropped still further when the analysis was restricted to neurons significantly impacted by laser (right hatched green bar). *<0.05, ***<0.001 in Chi-squared analyses.
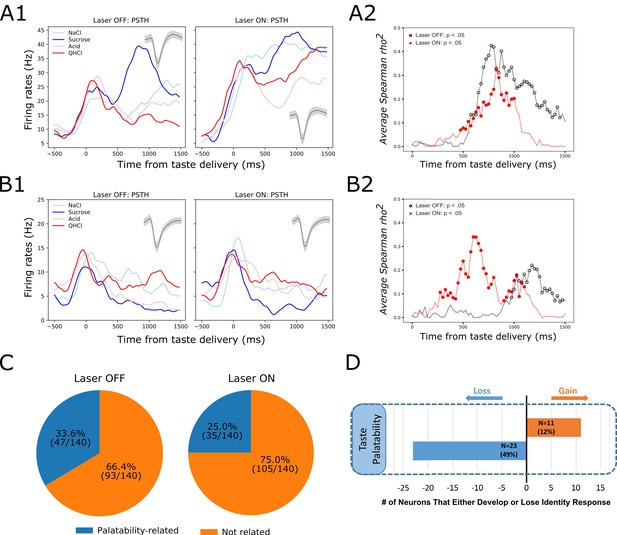
GC palatability information lost following perturbation on BLA→GC axons.
(A) Representative GC neuron showing decreased palatability-related activity by stimulation. PSTHs of this neuron (Panel A1) were plotted over Laser-Off trials (left panel) and Laser-On trials (right panel). Panel A2 is the moving window analysis of Spearman correlation between firing rates and taste palatability across post-stimulus time. (B) Representative GC neuron showing increased (unmasked) palatability-related activity following laser stimulation; PSTHs of the neuron are plotted in Panel B1 and palatability correlation is shown in Panel B2. (C) Overall, the number of GC neurons showing palatability activity was significantly decreased by laser stimulation from 33.6% to 25.0%. (D) Within-neuron analysis revealed that whereas ~50% of the GC neurons (N = 23) that initially showed palatability activity lost the response during perturbation, only 12% of the neurons (N = 11) gained palatability responsiveness following stimulation.
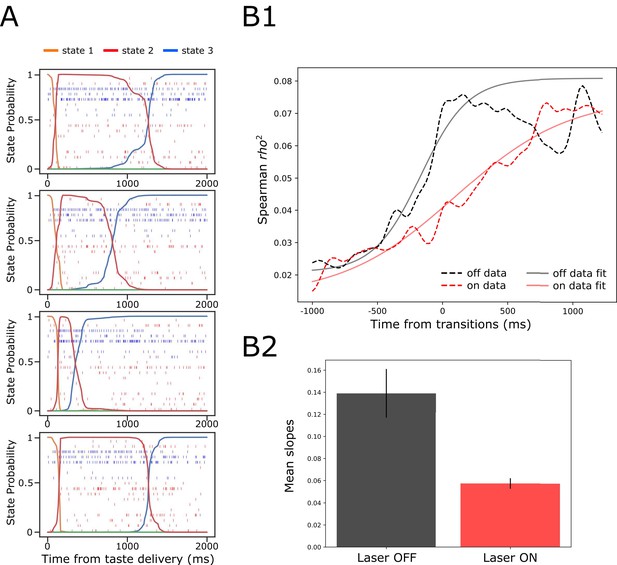
GC ensemble palatability activity was greatly impaired by perturbation on BLA→GC axons.
(A) Example ensemble responses evoked by NaCl administration characterized using HMM: The colored lines overlain on the ensemble of spike trains (each row representing a single neuron, y-axis) indicate the calculated probability that the ensemble is in that particular state. (B1) Solid lines are moving window analysis of palatability correlations between firing rates (calculated from spike trains ranged from 1000 ms before to 1500 ms after the transition time) and taste palatability; dashed lines are sigmoid fits for the raw data. (B2) The slopes of the sigmoid fits in B1 (error bars denote 95% Bayesian credible intervals); the development of correlations is significantly shallower on the perturbed (Laser-On) trials than on control (Laser-Off) trials.
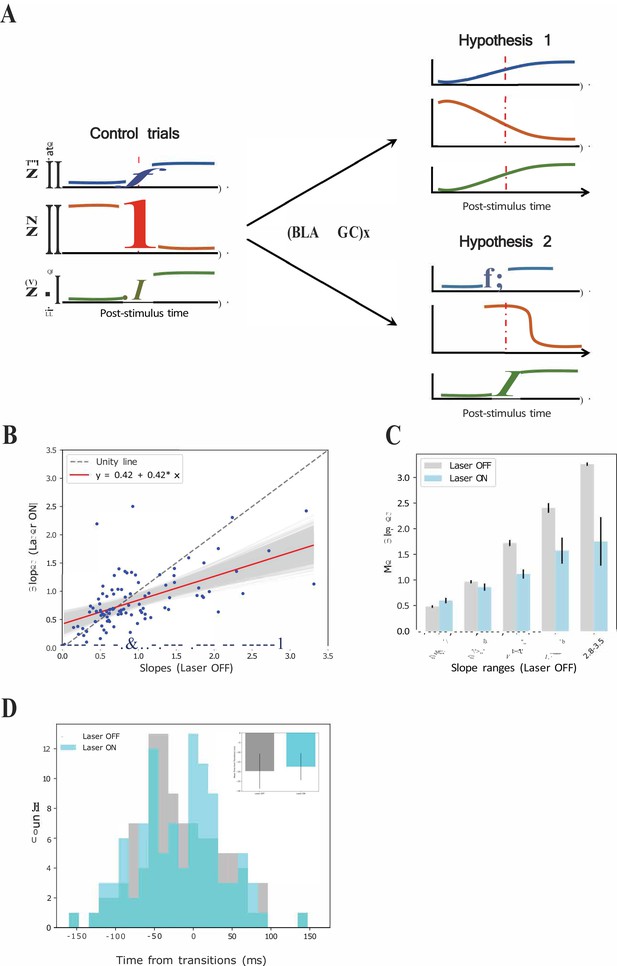
GCx reduces the sharpness of firing rate changes around state transitions into the palatability epoch.
(A) Schematics demonstrating two potential mechanisms by which BLA→GCx can decelerate the rise of palatability correlations. Hypothesis 1: laser stimulation causes a general reduction in the sharpness of firing-rate changes for individual neurons; Hypothesis 2: laser stimulation ‘decoupled’ the inter-neuron timing of those changes without altering the firing rate dynamics of each individual neuron. Red dashed line in each subpanel indicates the transition time during taste responses. (B) Scatter plot shows the slope changes for each GC neuron (Laser-Off [x-axis] against Laser-On [y-axis]). The red line is the regression fit of the data, and its slope was significantly shallower than the unity line (gray-dashed line with slope as 1, that is no impact of laser). (C) Mean slopes (± SEM) of GC neurons that were assigned into five groups based on the slopes in the control, Laser-Off, trials. As revealed, BLA→GCx (light blue bars) significantly reduced the changes in firing rates (slopes) around the state transition time. (D) Histogram of the latencies of when the sharpest slope occurred relative to the transition time across Laser-Off and Laser-On conditions. The mean latency (± SEM) are depicted in the inset, which reveals no significant difference across laser conditions. Accordingly, the reduction in the magnitude of firing changes (i.e. slopes) around the transition time likely accounts for the slowness in palatability correlation ramping up during perturbation on BLA→GC axons.
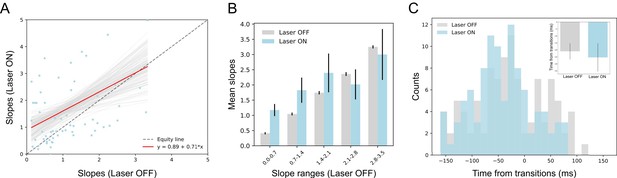
GCx has little impact on state transitions into the identity epoch.
(A) Scatter plot shows the slope changes during the transition times into the identity state (a dominant during 100 ~ 600 ms post-taste delivery) for each GC neuron (Laser-Off [x-axis] against Laser-On [y-axis]). The red line is the regression fit of the data, whose slope is not significantly from the unity line (gray-dashed line with slope as 1, i.e. no impact of laser). (B) Mean slopes (± SEM) of GC neurons that were assigned into five subgroups based on the slopes in the control, Laser-Off, trials. Consistent with Panel A, the changes in firing rates (slopes) around the state transition times were comparable between Laser-Off and Laser-On conditions. The seemingly increase in the Laser-On condition for the first two slope ranges (0.0–1.4) is a numerical difference and not supported by statistical significance (see text for more details). (C) Histogram of the latencies of when the sharpest slope occurred relative to the transition time across Laser-Off and Laser-On conditions. The mean latencies (± SEM) from each ensemble (the inset) are not significantly different across laser conditions. Thus, BLA→GCx has little impact on the state transitions into the identity epoch.
Tables
Reagent type (species) or resource | Designation | Source or reference | Identifiers | Additional information |
---|---|---|---|---|
Strain, strain background (virus) | AAV9-CAG-ArchT-GFP | UNC Vector Core | Lot #: AV6221E | |
Antibody | anti-GFP-rabbit IgG (rabbit Polyclonal) | Life Technologies | Cat#: A11122 RRID:AB_221569 | (1:500) |
Antibody | Alexa Flour 488 donkey anti-rabbit IgG (Donkey Polyclonal) | Life Technologies | Cat#: A21206 RRID:AB_2535792 | (1:200) |
Strain, strain background (female Long Evans Rat) | Charles River Laboratories | Strain code: 006 | ||
Chemical compound, drug | Sodium chloride | Fisher Scientific | Cat#: S271-500 | 0.1M (0.29 g/50 ml) |
Chemical compound, drug | Sucrose | Fisher Scientific | Cat#: S5-500 | 0.3M (5.14 g/50 ml) |
Chemical compound, drug | Citric acid | Fisher Scientific | Cat#: A104-500 | 0.1M (1.05 g/50 ml) |
Chemical compound, drug | Quinine hydrochloride dihydrate | Sigma-Aldrich | Cat#: Q1125-5G | 1 mM (0.0198 g/50 ml) |
Software, algorithm | Conda | Conda | RRID:SCR_018317 | |
Other | DAPI stain | Vector Laboratories, Inc | Cat#: H-1200–10 |