Defects in translation-dependent quality control pathways lead to convergent molecular and neurodevelopmental pathology
Figures
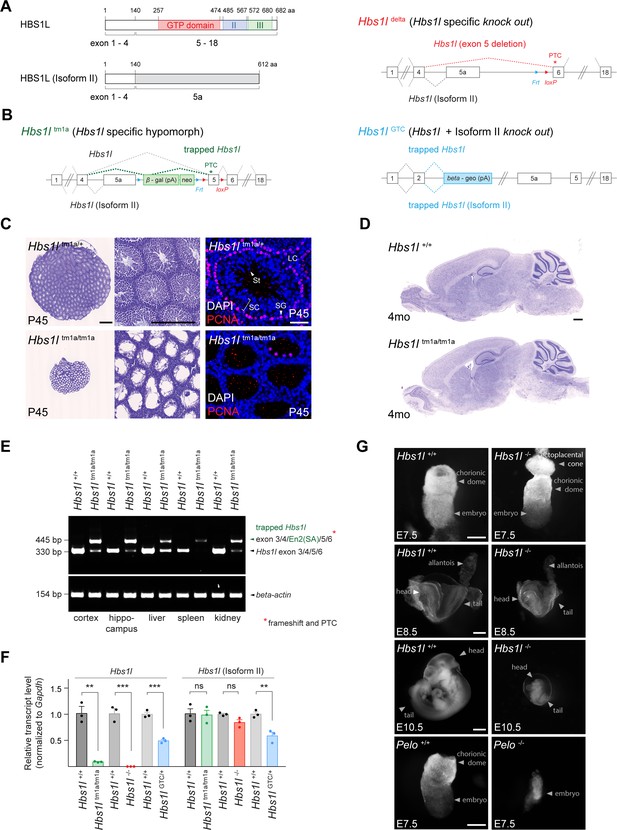
Hbs1l is required for embryogenesis.
(A) Domain structure of HBS1L and isoform II and the exons encoding the two splice variants. (B) Design of Hbs1l loss-of-function alleles that target Hbs1l and isoform II. Hbs1ltm1a allele, Hbs1l specific gene trap (hypomorph); Hbs1ldelta allele, Hbs1l specific deletion of exon 5 (Hbs1l-, knock out); and Hbs1lGTC allele, Hbs1l gene trap to target Hbs1l and isoform II (knock out). (C) Cresyl violet-stained cross-sections of testis from P45 control (Hbs1ltm1a/+) and Hbs1ltm1a/tm1a mice. Immunofluorescence was performed with antibodies to proliferating cell nuclear antigen (PCNA, red) and sections were counterstained with DAPI. (D) Cresyl violet-stained sagittal brain section from 4-month-old control (Hbs1l+/+) and Hbs1ltm1a/tm1a mice. (E) Splicing analysis of correctly spliced Hbs1l and trapped Hbs1l transcripts in various tissues from 4-month-old control (Hbs1l+/+) and Hbs1ltm1a/tm1a mice. β-actin was used as an input control. (F) Quantitative RT-PCR analysis of Hbs1l and isoform II using cDNA from E8.5 embryos. Data were normalized to Gapdh and the fold change in gene expression is relative to that of controls (Hbs1l+/+) from each cross. Data represent mean + SEM. (G) Bright field images of control (Hbs1l+/+ and Pelo+/+), Hbs1l-/- and Pelo-/- embryos at E7.5, E8.5, and E10.5. Scale bars: 500 μm and 200 μm (higher magnification), 50 μm (immunofluorescence image) (C); 500 μm (D); 100 μm (E7.5), 200 μm (E8.5), and 2 mm (E10.5) (G). PTC, premature termination codon; Frt, flippase-mediated recombination site; loxP, Cre recombinase-mediated recombination site; En2(SA), splice acceptor of mouse Engrailed-2 exon 2; SC, spermatocytes; SG, spermatogonia; St, spermatids; LC, Leydig cells. t-tests were corrected for multiple comparisons using Holm-Sidak method (F). ns, not significant; **p≤0.01; ***p≤0.001.
-
Figure 1—source data 1
Hbs1l is required for embryogenesis.
- https://cdn.elifesciences.org/articles/66904/elife-66904-fig1-data1-v1.xlsx
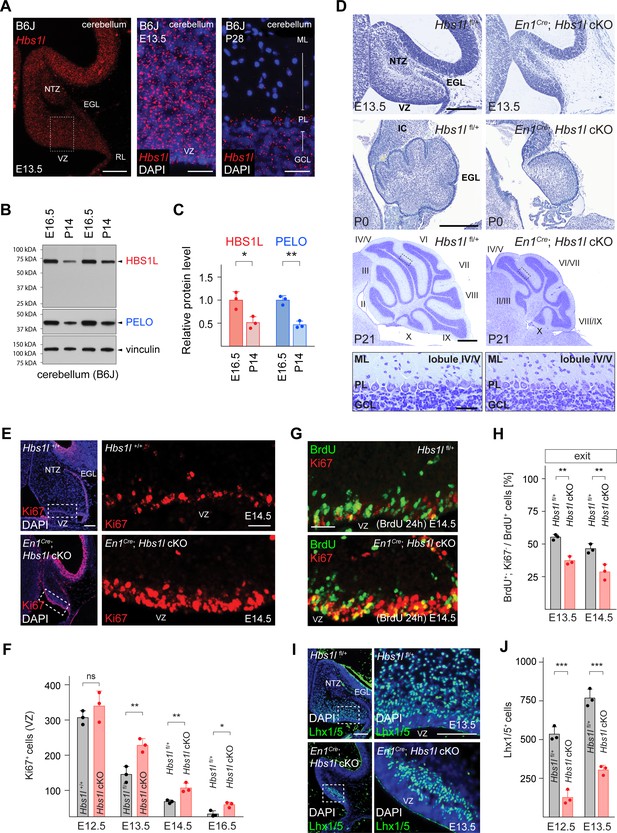
Hbs1l is required for cerebellar development.
(A) In situ hybridization of Hbs1l mRNA (red) on cerebellar sections from E13.5 and P28 control (B6J) mice. Sections were counterstained with DAPI. (B) Western blot analysis using cerebellar lysates from B6J mice. Vinculin was used as a loading control. (C) The relative protein levels of HBS1L and PELO were normalized to levels of vinculin and protein levels are relative to those of E16.5 B6J cerebella. (D) Parasagittal (E13.5) and sagittal (P0 and P21) cerebellar sections from control (Hbs1lfl/+) and En1Cre; Hbs1l cKO mice stained with cresyl violet. Higher magnification images of lobules IV/V at P21 are shown below each genotype. Cerebellar lobules are indicated by Roman numerals. (E) Immunofluorescence using antibodies to Ki67 (red) on cerebellar section from E14.5 control (Hbs1l+/+) and En1Cre; Hbs1l cKO embryos. Sections were counterstained with DAPI and higher magnification images of boxed area are shown. (F) Number of cerebellar VZ-progenitors (Ki67+ cells) from control (Hbs1l+/+ or Hbs1lfl/+) and En1Cre; Hbs1l cKO embryos. Data represent mean + SD. (G) Immunofluorescence using antibodies to BrdU (green) and Ki67 (red) on cerebellar sections from E14.5 control (Hbs1lfl/+) and En1Cre; Hbs1l cKO embryos to determine the fraction of cells that exited the cell cycle. Embryos were injected with BrdU 24 hr prior to harvest. (H) Percentage of cerebellar VZ-progenitors that exited the cell cycle (BrdU+, Ki67- cells). Data represent mean + SD. (I) Immunofluorescence using antibodies to Lhx1/5 on cerebellar sections from E13.5 control (Hbs1l+/+) and En1Cre; Hbs1l cKO embryos. Sections were counterstained with DAPI and higher magnification images of boxed areas are shown. (J) Number of cerebellar Purkinje cell precursors (Lhx1/5+ cells). Data represent mean + SD. Scale bars: 100 μm and 20 μm (higher magnifications) (A); 200 μm (E13.5 and P0), 500 μm and 50 μm (higher magnification) (P21) (D); 100 μm and 20 μm (higher magnification) (E); 20 μm (G); 100 μm and 50 μm (higher magnification) (I). VZ, ventricular zone; NTZ, nuclear transitory zone; RL, rhombic lip; EGL, external granule cell layer; ML, molecular cell layer; PL, Purkinje cell layer; GCL, granule cell layer; IC, inferior colliculus. t-tests were corrected for multiple comparisons using Holm-Sidak method (C, F, H, J). ns, not significant; *p≤0.05; **p≤0.01; ***p≤0.001.
-
Figure 2—source data 1
Hbs1l is required for cerebellar development.
- https://cdn.elifesciences.org/articles/66904/elife-66904-fig2-data1-v1.xlsx
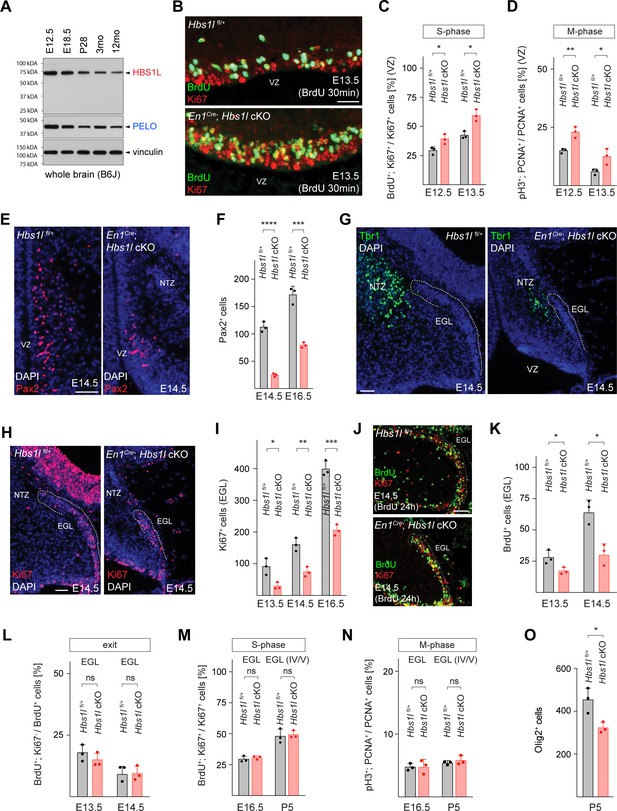
Hbs1l is required for the development of multiple cerebellar linages.
(A) Western blot analysis using brain lysates from B6J mice. Vinculin was used a loading control. (B) Immunofluorescence using antibodies to BrdU (green) and Ki67 (red) from cerebellar sections of E13.5 control (Hbs1lfl/+) and En1Cre; Hbs1l cKO embryos to determine the fraction of cells in S-phase. Embryos were injected with BrdU 30 min prior to harvest. Sections were counterstained with DAPI. (C) Percentage of cerebellar VZ-progenitors in S-phase (BrdU+, Ki67+ cells). Data represent mean + SD. (D) Percentage of cerebellar VZ-progenitors in M-phase (pH3+, PCNA+ cells). Data represent mean + SD. (E) Immunofluorescence using antibodies to Pax2 (red) on cerebellar sections from E13.5 control (Hbs1lfl/+) and En1Cre; Hbs1l cKO embryos. Sections were counterstained with DAPI. (F) Number of cerebellar VZ-derived interneuron precursors (Pax2+ cells). Data represent mean + SD. (G) Immunofluorescence using antibodies to Tbr1 (green) on cerebellar sections from E14.5 control (Hbs1lfl/+) and En1Cre; Hbs1l cKO embryos. Sections were counterstained with DAPI. (H) Immunofluorescence using antibodies to Ki67 (green) on cerebellar sections from E14.5 control (Hbs1lfl/+) and En1Cre; Hbs1l cKO embryos to identify granule cell precursors in the EGL. Sections were counterstained with DAPI. (I) Number of RL-derived granule cell precursors in the EGL (Ki67+ cells). Data represent mean + SD. (J) Immunofluorescence using antibodies to BrdU (green) and Ki67 (red) on cerebellar sections from E14.5 control (Hbs1lfl/+) and En1Cre; Hbs1l cKO embryos. Embryos were injected with BrdU 24 hr prior to harvest. (K) Number of RL-derived granule cell precursors (BrdU+ cells). Data represent mean + SD. (L) Percentage of RL-derived granule cell precursors that exited the cell cycle (BrdU+, Ki67- cells). Data represent mean + SD. (M) Percentage of RL-derived granule cell precursors in S-phase (BrdU+, Ki67+ cells). Embryos were injected with BrdU 30 min prior to harvest. Data represent mean + SD. (N) Percentage of RL-derived granule cell precursors in M-phase (pH3+, PCNA+ cells). Data represent mean + SD. (O) Number of oligodendroglial progenitors (Olig2+ cells) from E14.5 control (Hbs1lfl/+) and En1Cre; Hbs1l cKO cerebella. Scale bars: 20 μm (C); 50 μm (E, G, H, J). VZ, ventricular zone; NTZ; nuclear transitory zone; EGL, external granule cell layer; RL, rhombic lip. t-tests were corrected for multiple comparisons using Holm-Sidak method (C, D, F, I, K, L, M, N); Student’s t-test (O). ns, not significant; *p≤0.05; **p≤0.01; ***p≤0.001; ****p≤0.0001.
-
Figure 2—figure supplement 1—source data 1
Hbs1l is required for the development of multiple cerebellar linages.
- https://cdn.elifesciences.org/articles/66904/elife-66904-fig2-figsupp1-data1-v1.xlsx
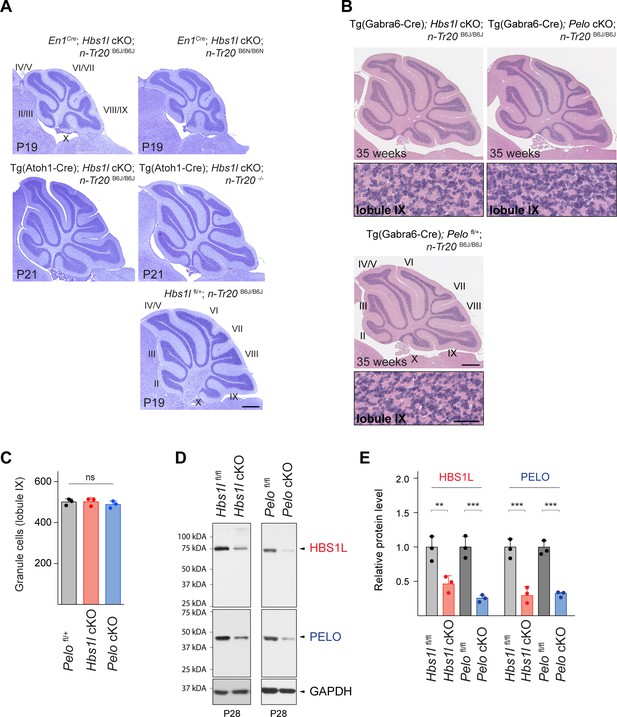
Hbs1l-/--mediated cerebellar defects are independent of the B6J-associated mutation in n-Tr20.
(A) Cresyl violet staining of sagittal sections of En1Cre; Hbs1l cKO cerebellum expressing wild-type levels of n-Tr20 (n-Tr20B6N/B6N) or reduced levels of n-Tr20 (n-Tr20B6J/B6J) and Tg(Atoh1-Cre); Hbs1l cKO cerebella with reduced levels of n-Tr20 (n-Tr20B6J/B6J) or that completely lack n-Tr20 (n-Tr20-/-). Cerebellar lobules are indicated by Roman numerals. (B) Sagittal sections from 35-week-old control (Tg(Gabra6-Cre); Pelofl/+; n-Tr20B6J/B6J), Tg(Gabra6-Cre); Pelo cKO; n-Tr20B6J/B6J and Tg(Gabra6-Cre); Hbs1l cKO; n-Tr20B6J/B6J cerebella stained with hematoxylin and eosin. Higher magnification images of lobule IX are shown below. Cerebellar lobules are indicated by Roman numerals. (C) Number of cerebellar granule cells in lobule IX shown in B. Data represent mean + SD. (D) Western blot analysis using cerebellar lysates from P28 control (Hbs1lfl/fl; n-Tr20B6J/B6J or Pelofl/fl; n-Tr20B6J/B6J), Tg(Gabra6-Cre); Pelo cKO; n-Tr20B6J/B6J and Tg(Gabra6-Cre); Hbs1l cKO; n-Tr20B6J/B6J mice. GAPDH was used a loading control. (E) Quantification of HBS1L and PELO protein levels shown in D. Levelswere normalized to levels of GAPDH. Protein levels are relative to those of controls (Hbs1lfl/fl or Pelofl/fl) from each cross. Scale bars: 500 μm (A); 500 μm and 50 μm (higher magnification) (B). One-way ANOVA (C) and two-way ANOVA (E) were corrected for multiple comparisons using Tukey method. ns, not significant; **p≤0.01; ***p≤0.001.
-
Figure 2—figure supplement 2—source data 1
Hbs1l-/--mediated cerebellar defects are independent of the B6J-associated mutation in n-Tr20.
- https://cdn.elifesciences.org/articles/66904/elife-66904-fig2-figsupp2-data1-v1.xlsx
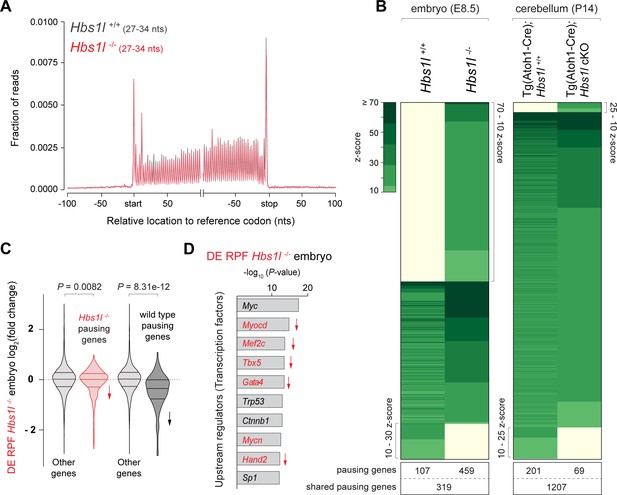
Ribosome pausing correlates with pathology in Hbs1l-deficient mice.
(A) Metagene profiles of RPFs from E8.5 control (Hbs1l+/+, gray traces) and Hbs1l-/- (red traces) embryos. (B) Analysis of significantly increased local ribosome occupancy (z-score ≥10, pause site detected in all three replicates) from E8.5 wild type (Hbs1l+/+) and Hbs1l-/- embryos (left) or P14 control (Tg(Atoh1-Cre); Hbs1l+/+) and Tg(Atoh1-Cre); Hbs1l cKO cerebella (right). The number of genes that pause sites map to is shown below for each genotype. (C) All translated genes (DE RPF Hbs1l-/-) from E8.5 Hbs1l-/- embryos were compared to the translation of genes which contained pauses specific to either Hbs1l-/- or wild-type embryos. Downward direction of arrows indicates significant reduction in translation of pausing genes in E8.5 Hbs1l-/- embryos relative to wild-type embryos. (D) Identification of upstream regulators using Ingenuity Pathway Analysis (IPA) of differentially translated genes of E8.5 Hbs1l-/- embryos (DE RPF Hbs1l-/-). Transcription factors that are involved in heart development are shown in red. Downward direction of arrows indicates predicated activity (downregulation) of transcription factors. RPFs, ribosome-protected fragments; nts, nucleotides. Wilcoxon rank-sum test was used to determine statistical significance (C).
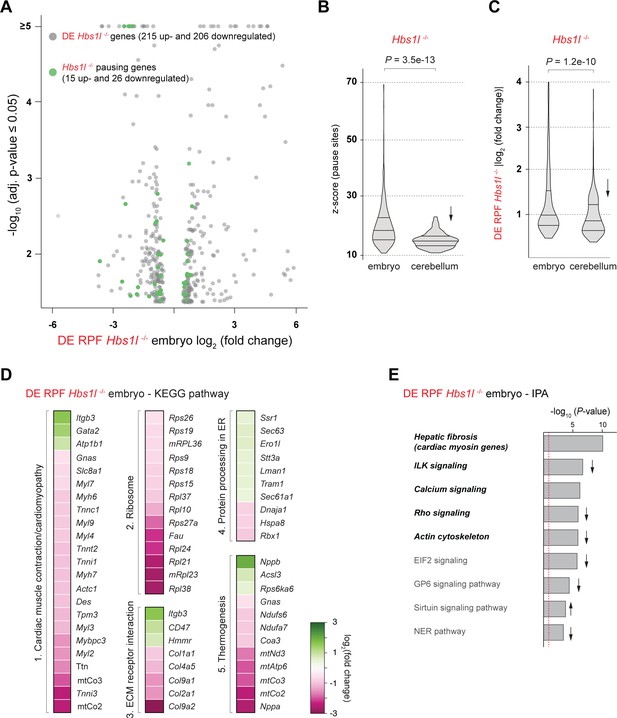
Hbs1l deficiency in embryos alters translation of pathways associated with heart function.
(A) Analysis of differentially translated genes (DE RPF Hbs1l-/-, adj. p≤0.05) of E8.5 Hbs1l-/- embryos (421 genes, gray). Genes that have ribosome pauses specific to Hbs1l-/- embryos with significant (adj. p≤0.05) changes in translation are shown in green (47 genes). (B) Comparison of z-scores of Hbs1l-/--specific pause sites detected in E8.5 Hbs1l-/- embryos and P14 Tg(Atoh1-Cre); Hbs1l cKO cerebella. Downward direction of the arrow indicates significant lower z-scores of these pauses in P14 Tg(Atoh1-Cre); Hbs1l cKO cerebella. (C) Comparison of differentially translated genes (DE RPF Hbs1l-/-, adj. p≤0.05) of E8.5 Hbs1l-/- embryos and P14 Tg(Atoh1-Cre); Hbs1l cKO cerebella. Downward direction of the arrow indicates significant lower fold changes in translation in P14 Tg(Atoh1-Cre); Hbs1l cKO cerebella. (D) KEGG pathway analysis of differentially translated genes (DE RPF Hbs1l-/-, adj. p≤0.05) of E8.5 Hbs1l-/- embryos. Top five significantly (p≤0.05) enriched pathways are shown and the heatmap indicates relative changes in genes associated with the respective pathway. (E) Ingenuity Pathway Analysis (IPA) of differentially translated genes (DE RPF Hbs1l-/-) from E8.5 Hbs1l-/- embryos. Pathways that are involved in heart, muscle contraction, cardiomyocyte, and cytoskeleton function are in italics. The red dashed line indicates the significance threshold (p=0.05). Up- or downward direction of arrows indicates predicated up- or downregulated activity of the pathway, respectively. RPF, ribosome protected fragments. Wilcoxon rank-sum test was used to determine statistical significance (B, C).
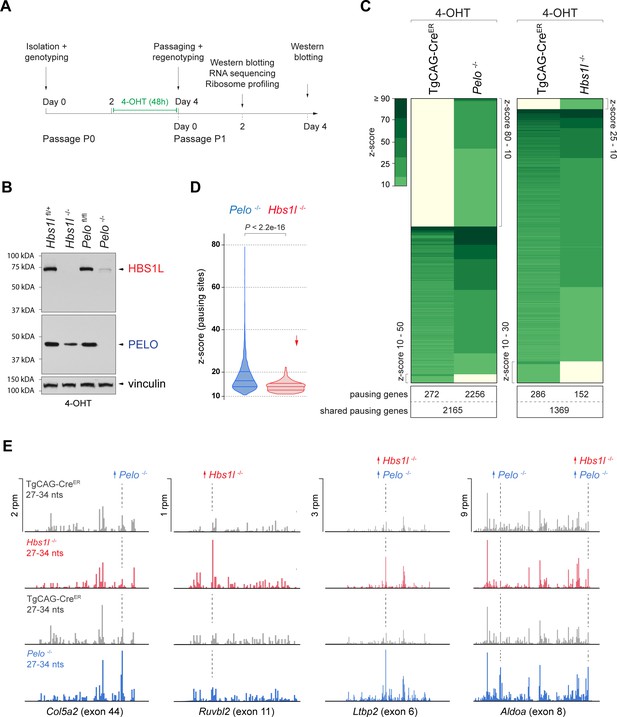
Loss of Pelo induces greater defects in translation elongation then loss of Hbs1l.
(A) Experimental strategy for in vitro studies using tamoxifen (4-OHT) treatment of primary mouse embryonic fibroblasts (MEFs). (B) Western blot analysis of HBS1L and PELO using MEF lysates from tamoxifen-treated control (Hbs1lfl/+ or Pelofl/fl), Hbs1l-/- and Pelo-/- cells. Vinculin was used as a loading control. (C) Analysis of significantly increased local ribosome occupancy (z-score ≥10, pause site detected in all three replicates) from tamoxifen-treated control (TgCAG-CreER) and Pelo-/- (left), or control and Hbs1l-/- cells (right). The number of genes that pause sites map to is shown below for each genotype. (D) Comparison of the z-scores (‘pause score’) for pauses observed in Pelo-/- (blue) and Hbs1l-/- (red) cells. Downward direction of the arrow indicates significant lower pause scores of Hbs1l-/-- compared to Pelo-/--specific pauses. (E) Examples of mapped footprints (27–34 nucleotides) on genes from tamoxifen-treated control (TgCAG-CreER, gray) and Pelo-/- (blue), or control and Hbs1l-/- (red) cells. Upward direction of arrows indicates significant increase in local ribosome occupancy and the dashed line indicates the pause site. 4-OHT, 4-hydroxytamoxifin; nts, nucleotides; rpm, reads per million. Wilcoxon rank-sum test was used to determine statistical significance (D).
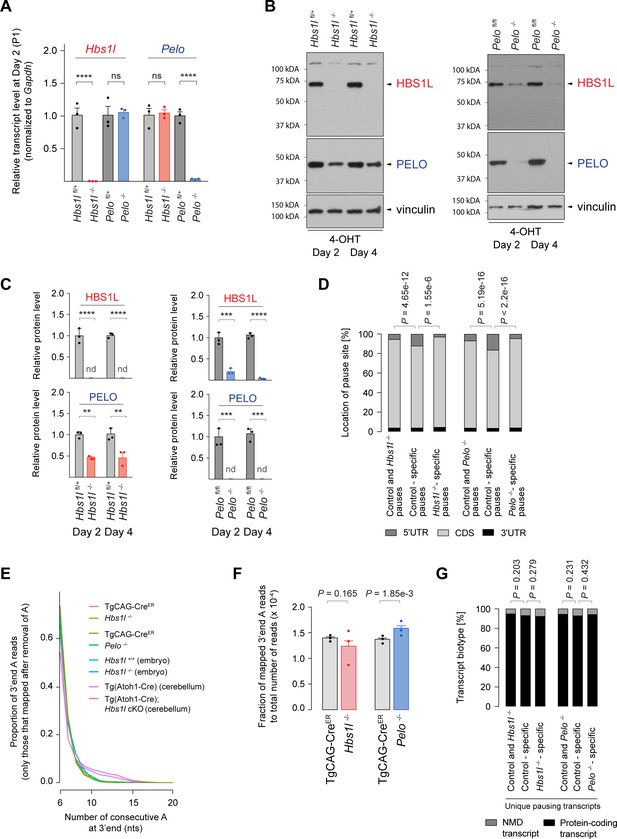
Loss of Hbs1l and Pelo control protein levels of their respective binding partner.
(A) Quantitative RT-PCR analysis of Hbs1l and Pelo transcripts using cDNA from MEFs of tamoxifen-treated control (Hbs1lfl/+ or Pelofl/+), Hbs1l-/- and Pelo-/- cells at Day 2 (Passage P1). Data were normalized to Gapdh and fold change in expression is relative to those of control (Hbs1lfl/+ or Pelofl/+) cells. Data represent mean + SEM. (B) Western blot analysis of HBS1L and PELO using MEF lysates of tamoxifen-treated control (Hbs1lfl/+ or Pelofl/fl), Hbs1l-/- or Pelo-/- cells at Day 2 and 4 (Passage P1). Vinculin was used as a loading control. (C) Quantification of HBS1L and PELO protein levels shown in B. Levels were normalized to levels of vinculin. Protein levels are relative to those of control (Hbs1lfl/+ or Pelofl/fl) cells at Day 2 (Passage P1). Data represent mean + SD. (D) Location of pause sites. (E) Histogram of A count on the 3’end of ribosome footprints that mapped after removal of the 3’end A’s. (F) Fraction of 3’ end A ribosome footprints that mapped after removal of the 3’ end A’s relative to the total number of ribosome footprints from tamoxifen-treated control (TgCAG-CreER) and Hbs1l-/-, or control (TgCAG-CreER) and Pelo-/- cells. (G) Percent of unique pausing transcripts that are protein-coding or NMD transcripts. P1, Passage 1; MEFs, primary mouse embryonic fibroblasts; 4-OHT, 4-hydroxytamoxifin; PTC, premature termination codon; nd, not detected; A, adenosines; nts, nucleotides; CDS, coding sequence; UTR, untranslated region; NMD, nonsense-mediated decay. Two-way ANOVA was corrected for multiple comparisons using Tukey method (A, C); Fisher’s exact test (D, G); Student’s t-test (F). ns, not significant; **p≤0.01; ***p≤0.001; ****p≤0.0001.
-
Figure 4—figure supplement 1—source data 1
Loss of Hbs1l and Pelo control protein levels of their respective binding partner.
- https://cdn.elifesciences.org/articles/66904/elife-66904-fig4-figsupp1-data1-v1.xlsx
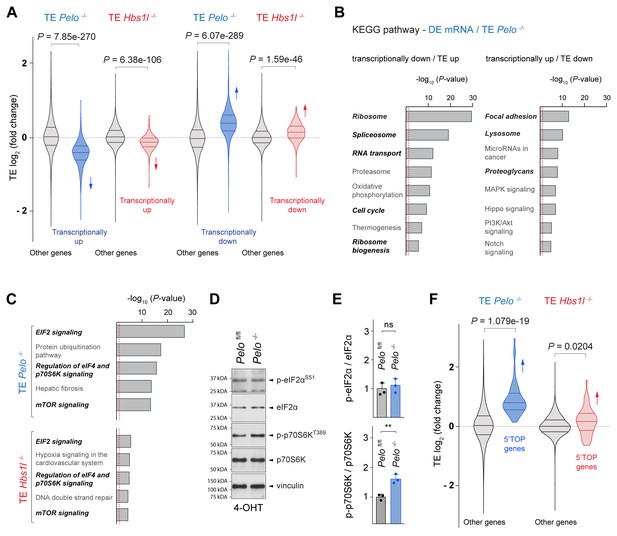
Pelo/Hbs1l deficiency alters translation regulation and reprograms the translatome.
(A) Translational efficiency (TE) of genes that are transcriptionally upregulated or downregulated was compared to the remaining (‘other’) genes from Pelo-/- (blue) and Hbs1l-/- (red) MEFs, respectively. Downward direction of arrows indicates significant decrease in translational efficiency of transcriptionally upregulated genes in Pelo-/- and Hbs1l-/- MEFs. Upward direction of arrows indicates significant increase in translational efficiency of transcriptionally downregulated genes in Pelo-/- and Hbs1l-/- MEFs. (B) KEGG pathway analysis of genes from Pelo-/- MEFs in which the translational efficiency (TE Pelo-/-, adj. p≤0.05) is opposite to their transcriptional expression (DE mRNA Pelo-/-, q-value ≤0.05). Italicized pathways indicate pathways that overlapped with enriched pathways of differentially translated genes in Pelo-/- MEFs (DE RPF Pelo-/-, adj. p≤0.05) (Figure 5—figure supplement 1E). The red dashed line indicates the significance threshold (p=0.05). (C) Ingenuity pathway analysis (IPA) of genes with differential translational efficiency from Pelo-/- (TE Pelo-/-) and Hbs1l-/- (TE Hbs1l-/-) MEFs. EIF2 and mTOR/p70S6K signaling are in italics. The red dashed line indicates the significance threshold (p=0.05). (D) Western blot analysis of p-eIF2αS51 and p-p70S6KT389 using lysates of tamoxifen-treated control (Pelofl/fl) and Pelo-/- MEFs at Day 2 (Passage P1). Vinculin was used as a loading control. (E) Levels of p-eIF2αS51 or p-p70S6KT389 were normalized to total level of eIF2α or p70S6K, and phosphorylation levels are relative to those of control (Pelofl/fl). Data represent mean + SD. (F) Translational efficiency (TE) of genes with translational regulation by mTOR via their 5’TOP motif was compared to the remaining (‘other’) genes from Pelo-/- (blue) and Hbs1l-/- (red) MEFs. Upward direction of arrows indicates significant increase in translational efficiency of 5’TOP genes. 4-OHT, 4-hydroxytamoxifin; 5’TOP, 5’terminal oligopyrimidine motif. Student’s t-test (E); Wilcoxon rank-sum test was used to determine statistical significance (A, F). ns, not significant; **p≤0.01.
-
Figure 5—source data 1
Pelo/Hbs1l deficiency alters translation regulation and reprograms the translatome.
- https://cdn.elifesciences.org/articles/66904/elife-66904-fig5-data1-v1.xlsx
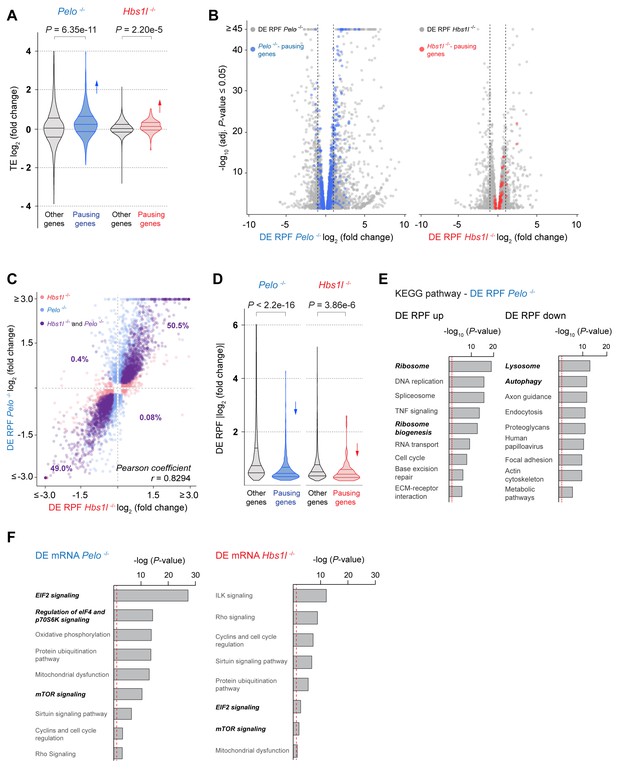
Pelo/Hbs1l deficiency alters translational gene expression of multiple pathways.
(A) Translational efficiency (TE) of Pelo-/-- and Hbs1l-/--pausing genes was compared to the TE of the remaining (‘other’) genes from Pelo-/- (blue) and Hbs1l-/- (red) MEFs. Upward direction of arrows indicates significant increase in translational efficiency of Pelo-/-- and Hbs1l-/--pausing genes. (B) Analysis of differentially translated genes in Pelo-/- (DE RPF Pelo-/-, adj. p≤0.05, left) and Hbs1l-/- (DE RPF Hbs1l-/-, adj. p≤0.05, right) MEFs are shown in gray. Pelo-/-- and Hbs1l-/--specific pausing genes (z-score ≥10, detected in all three biological replicates) that are differentially translated are highlighted. Twofold changes in expression are indicated by the black dashed lines. (C) Differentially translated genes in Hbs1l-/- MEFs (DE RPF Hbs1l-/-, adj. p≤0.05, x-axis, red) were plotted against genes that are differentially translated in Pelo-/- MEFs (DE RPF Pelo-/-, adj. p≤0.05, y-axis, blue). Genes those translation was significantly different in both Hbs1l-/- and Pelo-/- MEFs are shown in purple. (D) Differential translation of genes in Pelo (DE RPF Pelo-/-, adj. p≤0.05) and Hbs1l (DE RPF Hbs1l-/-, adj. p≤0.05) was compared to that of Pelo-/-- and Hbs1l-/--specific pausing genes. Downward direction of arrows indicates significantly reduced changes in RPFs from the pausing genes compared to non-pausing (‘other’) genes. (E) KEGG pathway analysis of differentially translated (up- and downregulated) genes (DE RPF Pelo-/-, adj. p≤0.05) in Pelo-/- MEFs. Top nine of the significantly enriched pathways are shown. The red dashed line indicates the significance threshold (p=0.05). Pathways that are positively (ribosome, ribosome biogenesis) and negatively (lysosome, autophagy) regulated by mTORC1 are in italics. (F) Ingenuity pathway analysis (IPA) of differentially transcribed genes in Pelo (DE mRNA Pelo-/-) and Hbs1l (DE mRNA Hbs1l-/-). EIF2 and mTOR/p70S6K signaling are in italics. The red dashed line indicates the significance threshold (p=0.05). RPF, ribosome protected fragments. Wilcoxon rank-sum test was used to determine statistical significance (A, D); Pearson coefficient (r) was determined to analyze linearity of gene expression changes (C).
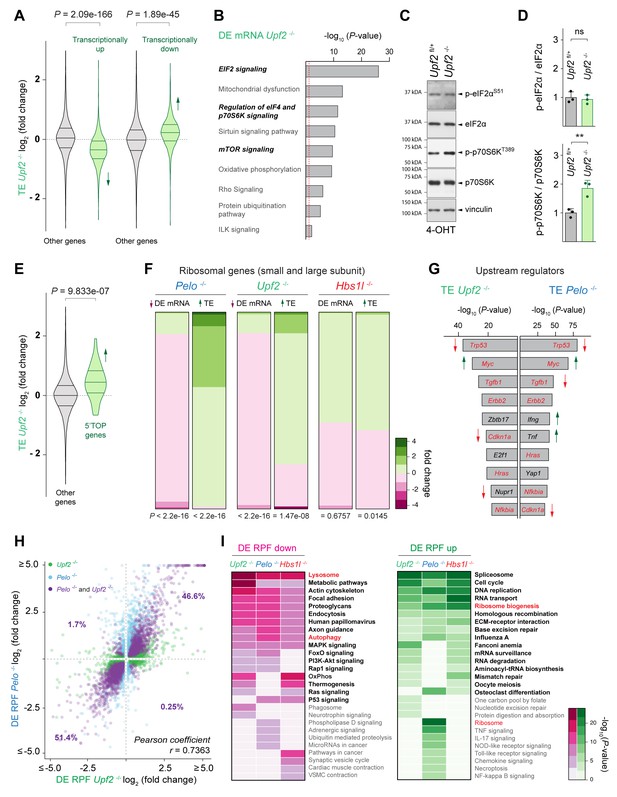
Defects in different translation-dependent quality control pathways similarly alter translation regulation and the translatome.
(A) Translational efficiency (TE) of genes that are transcriptionally upregulated or downregulated was compared to the remaining (‘other’) genes from Upf2-/- (green) MEFs. Downward direction of the arrow indicates significant decrease in translational efficiency of transcriptionally upregulated genes in Upf2-/- MEFs. Upward direction of the arrow indicates significant increase in translational efficiency of transcriptionally downregulated genes in Upf2-/- MEFs. (B) Ingenuity pathway analysis (IPA) of differentially transcribed genes from Upf2-/- MEFs (DE mRNA Upf2-/-). EIF2 and mTOR/p70S6K signaling are in italics. The red dashed line indicates the significance threshold (p=0.05). (C) Western blot analysis of p-eIF2αS51 and p-p70S6KT389 of lysates from tamoxifen-treated control (Upf2fl/+) and Upf2-/- MEFs at Day 2 (Passage P1). Vinculin was used as a loading control. (D) Levels of p-eIF2αS51 or p-p70S6KT389 were normalized to total level of eIF2α or p70S6K, and phosphorylation levels are relative to those of control (Upf2fl/+) MEFs. Data represent mean + SD. (E) Translational efficiency (TE) of genes those translation is regulated by mTOR via their 5’TOP motif was compared to the remaining (‘other’) genes from Upf2-/- (green) MEFs. Upward direction of the arrow indicates significant increase in translational efficiency of 5’TOP genes. (F) Differential transcription (DE mRNA) or translational efficiency (TE) of ribosomal protein genes (small and large ribosomal subunit) was compared to the remaining (‘other’) genes from Pelo-/- (blue), Upf2-/- (green), and Hbs1l-/- (red) MEFs. Up- and downward direction of arrows indicates significant up- and downregulation of ribosomal protein genes, respectively. The heatmap indicates the gene expression changes of ribosomal protein genes. (G) Identification of upstream regulators of genes with differential translational efficiency in Upf2-/- (TE Upf2-/-) and Pelo-/- (TE Pelo-/-) MEFs. Top ten transcription factors are shown. Those enriched in both Upf2-/- and Pelo-/- MEFs and shown in red. Up- or downward direction of arrows indicates predicted up- or downregulation of transcription factors, respectively. (H) Differentially translated genes in Upf2-/- MEFs (DE RPF Upf2-/-, adj. p≤0.05, x-axis, green) were plotted against genes that are differentially translated in Pelo-/- MEFs (DE RPF Pelo-/-, adj. p≤0.05, y-axis, blue). Genes those translation was significantly different in both Upf2-/- and Pelo-/- MEFs are shown in purple. (I) KEGG pathway analysis of differentially translated (up- and downregulated) genes (DE RPF, adj. p≤0.05) in Upf2-/- (green), Pelo-/- (blue) and Hbs1l-/- (red) MEFs. Significantly (p≤0.05) enriched pathways are shown and pathways in bold indicate pathways that are shared between any of the mutant MEFs. Pathways known to be positively or negatively regulated by mTORC1 are in red. 4-OHT, 4-hydroxytamoxifin; 5’TOP, 5’terminal oligopyrimidine motif. Student’s t-test (D); Wilcoxon rank-sum test was used to determine statistical significance (A, E, F); Pearson coefficient (r) was determined to analyze linearity of gene expression changes (H). ns, not significant; **p≤0.01.
-
Figure 6—source data 1
Defects in different translation-dependent quality control pathways similarly alter translation regulation and the translatome.
- https://cdn.elifesciences.org/articles/66904/elife-66904-fig6-data1-v1.xlsx
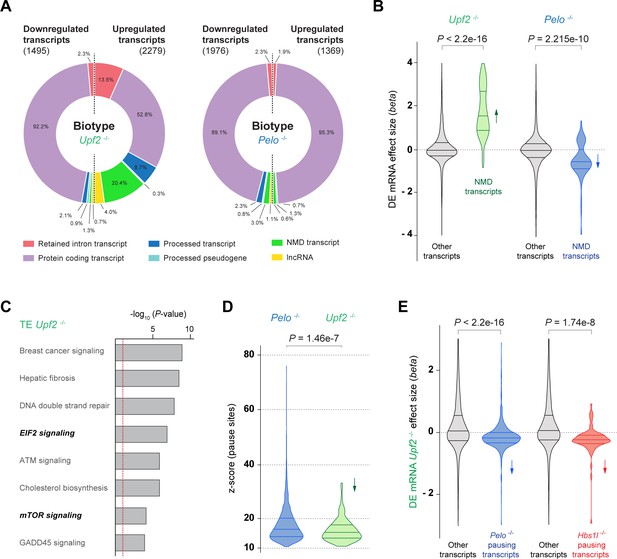
Loss of Upf2 leads to an increase of NMD targets.
(A) Ensembl-annotated transcript biotypes of differentially transcribed mRNAs in Upf2-/- (DE mRNA Upf2-/-, q-value ≤0.05) and Pelo-/- (DE mRNA Pelo-/-, q-value ≤0.05) MEFs. (B) Transcriptional expression of NMD transcripts with significant (q-value ≤0.05) changes in expression in Upf2-/- (DE mRNA Upf2-/-) and Pelo-/- (DE mRNA Pelo-/-) was compared to expression of the remaining (‘other’) transcripts. The effect size (beta) is analogous to the natural log fold change in expression. Up- or downward direction of arrows indicates significant increase or decrease in expression of NMD transcripts in Upf2-/- or Pelo-/- MEFs. (C) Ingenuity pathway analysis (IPA) of genes with differential translational efficiency (TE) in Upf2-/- MEFs (TE Upf2-/-). EIF2 and mTOR signaling are in italics. The red dashed line indicates the significance threshold (p=0.05). (D) Comparison of z-scores (‘pause score’) of specific pauses observed in Pelo-/- (blue) and Upf2-/- (green) MEFs. Downward direction of the arrow indicates significantly lower pause scores specific to Upf2-/- compared to Pelo-/- MEFs. (E) Transcriptional expression of either Pelo- or Hbs1l-specific pausing transcripts (unique protein-coding pausing transcripts) was compared to the remaining (‘other’) transcripts in Upf2-/- (DE mRNA Upf2-/-) MEFs. The effect size (beta) is analogous to the natural log fold change in expression. Downward direction of arrows indicates a significant decrease in expression of Pelo- or Hbs1l-specific pausing transcripts in Upf2-/- MEFs. NMD, nonsense-mediated transcript; lncRNA, long noncoding RNA. Wilcoxon rank-sum test was used to determine statistical significance (B, D, E).
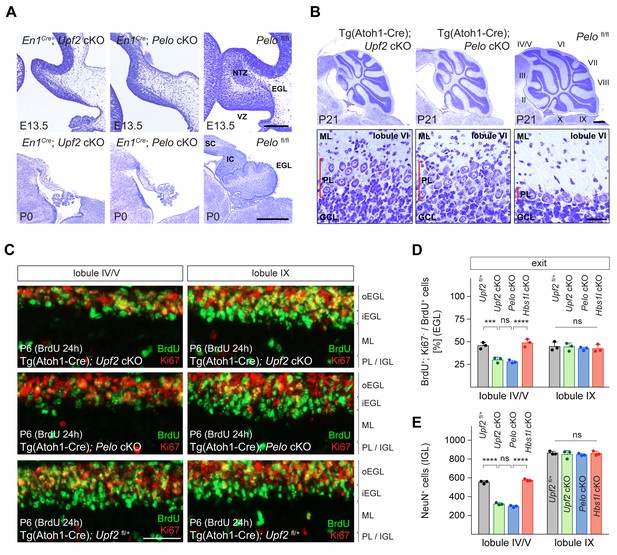
Loss of Upf2 and Pelo cause similar cerebellar developmental defects.
(A) Parasagittal (E13.5) and sagittal (P0) cerebellar sections of control (Pelofl/fl), En1Cre; Upf2 cKO and En1Cre; Pelo cKO mice stained with cresyl violet. (B) Sagittal cerebellar sections of P21 control (Pelofl/fl), Tg(Atoh1-Cre); Upf2 cKO and Tg(Atoh1-Cre); Pelo cKO mice stained with cresyl violet. Higher magnification images of lobule VI are shown below for each genotype. Cerebellar lobules are indicated by Roman numerals. (C) Immunofluorescence with antibodies to BrdU (green) and Ki67 (red) on sections of P6 control (Tg(Atoh1-Cre); Upf2fl/+), Tg(Atoh1-Cre); Upf2 cKO and Tg(Atoh1-Cre); Pelo cKO cerebellum. Mice were injected with BrdU 24 hr prior to harvest to determine the fraction of granule cell precursors in the EGL that exited the cell cycle. Images are shown for anterior (IV/V) and posterior (IX) lobules. (D) Quantification of the fraction of granule cell precursors in the EGL (lobules IV/V and IX) that exited the cell cycle (BrdU+, Ki67- cells) of control (Tg(Atoh1-Cre); Upf2fl/+), Tg(Atoh1-Cre); Upf2 cKO, Tg(Atoh1-Cre); Pelo cKO and Tg(Atoh1-Cre); Hbs1l cKO mice. Data represent mean + SD. (E) Quantification of terminally differentiated granule cells (NeuN+ cells) in the IGL in lobules IV/V and IX of control (Tg(Atoh1-Cre); Upf2fl/+), Tg(Atoh1-Cre); Upf2 cKO, Tg(Atoh1-Cre); Pelo cKO and Tg(Atoh1-Cre); Hbs1l cKO mice. Data represent mean + SD. Scale bars: 200 μm (E13.5) and 500 μm (P0) (A); 500 μm and 20 μm (higher magnification) (B); 50 μm (C). VZ, ventricular zone, EGL, external granule cell layer; NTZ, nuclear transitory zone; SC, superior colliculus; IC, inferior colliculus; ML, molecular cell layer; PL, Purkinje cell layer; GCL, granule cell layer; oEGL, outer external granule cell layer; iEGL, inner external granule cell layer; IGL, internal granule cell layer. Two-way ANOVA was corrected for multiple comparisons using Tukey method (D, E). ns, not significant; ***p≤0.001; ****p≤0.0001.
-
Figure 7—source data 1
Loss of Upf2 and Pelo cause similar cerebellar developmental defects.
- https://cdn.elifesciences.org/articles/66904/elife-66904-fig7-data1-v1.xlsx
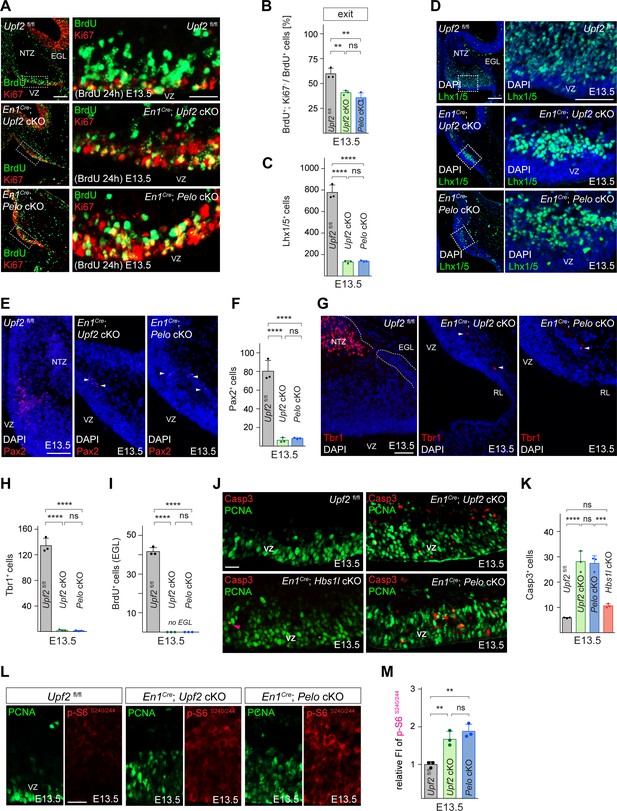
Upf2 and Pelo are required for the development of multiple cerebellar linages.
(A) Immunofluorescence using antibodies to BrdU (green) and Ki67 (red) on cerebellar sections from E13.5 control (Upf2fl/fl), En1Cre; Upf2 cKO and En1Cre; Pelo cKO embryos to determine the fraction of cells in VZ that exited the cell cycle. Embryos were injected with BrdU 24 hr prior to harvest. (B) Percentage of cerebellar VZ-progenitors that exited the cell cycle (BrdU+, Ki67- cells). Data represent mean + SD. (C) Number of cerebellar Purkinje cell precursors (Lhx1/5+ cells). Data represent mean + SD. (D) Immunofluorescence using antibodies to Lhx1/5 on cerebellar sections from E13.5 control (Upf2fl/fl), En1Cre; Upf2 cKO and En1Cre; Pelo cKO embryos. Sections were counterstained with DAPI and higher magnification images of boxed areas are shown. (E) Immunofluorescence using antibodies to Pax2 (red) on cerebellar sections from E13.5 control (Upf2fl/fl), En1Cre; Upf2 cKO and En1Cre; Pelo cKO embryos. Sections were counterstained with DAPI. Arrowheads denote occasional Pax2+ cells. (F) Number of cerebellar VZ-derived interneuron precursors (Pax2+ cells). Data represent mean + SD. (G) Immunofluorescence using antibodies to Tbr1 (red) on cerebellar sections from E13.5 control (Upf2fl/fl), En1Cre; Upf2 cKO and En1Cre; Pelo cKO embryos. Sections were counterstained with DAPI. Arrowheads denote occasional Tbr1+ cells. (H) Number of RL-derived Tbr1+ deep cerebellar neurons. Data represent mean + SD. (I) Quantification of newborn granule cell precursors (BrdU+ cells) in E13.5 control (Upf2fl/fl), En1Cre; Upf2 cKO and En1Cre; Pelo cKO EGL. Data represent mean + SD. Embryos were injected with BrdU 24 hr prior to harvest. (J) Immunofluorescence using antibodies to cleaved caspase 3 (Casp3, red) on cerebellar sections from E13.5 control (Upf2fl/fl), En1Cre; Upf2 cKO, En1Cre; Pelo cKO and En1Cre; Hbs1l cKO embryos. Sections were counterstained with DAPI. Note: Because the number of apoptotic cells was noticeably higher in En1Cre; Upf2 cKO and En1Cre; Pelo cKO cerebella, arrowheads (magenta) to indicate these cells were not included. (K) Number of Casp3+ cells. Data represent mean + SD. (L) Immunofluorescence on sections of E13.5 control (Upf2fl/fl), En1Cre; Upf2 cKO and En1Cre; Pelo cKO cerebellum with antibodies to p-S6S240/244 (red) and PCNA (green). (M) Relative fluorescence intensity (FI) of p-S6S240/244 of control (Upf2fl/fl), En1Cre; Upf2 cKO and En1Cre; Pelo cKO embryos. Levels of p-S6S240/244 are relative to those of control (Upf2fl/fl). Data represent mean + SD. Scale bars: 100 μm and 20 μm (higher magnification) (A); 100 μm and 50 μm (higher magnification) (D); 50 μm (E, G); 20 μm (J); 25 μm (L). VZ, ventricular zone; RL, rhombic lip; NTZ; nuclear transitory zone; EGL, external granule cell layer. One-way ANOVA was corrected for multiple comparisons using Tukey method (B, C, F, H, I, K, M). ns, not significant; **p≤0.01; ***p≤0.001; ****p≤0.0001.
-
Figure 7—figure supplement 1—source data 1
Upf2 and Pelo are required for the development of multiple cerebellar linages.
- https://cdn.elifesciences.org/articles/66904/elife-66904-fig7-figsupp1-data1-v1.xlsx
Tables
Reagent type (species) or resource | Designation | Source or reference | Identifiers | Additional information |
---|---|---|---|---|
Strain, strain background (mouse) | Hbs1lGTC | This study (see Materials and methods) | MMRRC #007694-UCD; RRID:MMRRC007694-UCD | International Gene Trap Consortium, IGTC, cell line ID: XE494 |
Strain, strain background (mouse) | Hbs1ltm1a(C57BL/6N-Atm1BrdHbs1ltm1a(KOMP)Wtsi) | Skarnes et al., 2011 | MMRRC #048037-UCD, RRID:MMRRC048037-UCD | |
Strain, strain background (mouse) | B6N.129S4-Gt(ROSA)26Sortm1(FLP1)Dym/J | The Jackson Laboratory | JAX:016226; RRID:IMSRJAX:016226 | |
Strain, strain background (mouse) | B6N.Cg-Edil3Tg(Sox2-Cre)1Amc/J | The Jackson Laboratory | JAX:014094; RRID:IMSRJAX:014094 | |
Strain, strain background (mouse) | B6.FVB-Tg(EIIa-Cre)C5379Lmgd/J | The Jackson Laboratory | JAX:003724; RRID:IMSRJAX:003724 | |
Strain, strain background (mouse) | En1tm2(Cre)Wrst/J | The Jackson Laboratory | JAX:007916; RRID:IMSRJAX:007916 | |
Strain, strain background (mouse) | B6.Cg-Tg(Atoh1-Cre)1Bfri/J | The Jackson Laboratory | JAX:011104; RRID:IMSRJAX:011104 | |
Strain, strain background (mouse) | B6.Cg-Tg(CAG-Cre/Esr1*)5Amc/J | JAX:004682; RRID:IMSRJAX:004682 | ||
Strain, strain background (mouse) | B6.Tg(Gabra6-Cre)B1Lfr | Fünfschilling and Reichardt, 2002 | N/A | |
Strain, strain background (mouse) | B6J-Pelofl/fl | This study (see Materials and methods) | N/A | |
Strain, strain background (mouse) | Upf2fl/fl | Weischenfeldt et al., 2008 | N/A | |
Strain, strain background (mouse) | B6J.B6Nn-Tr20 | Ishimura et al., 2014 | N/A | |
Strain, strain background (mouse) | B6J-n-Tr20-/- | Ishimura et al., 2016 | N/A | |
Antibody | Anti-phospho-eIF2alphaS51 (Rabbit polyclonal) | Cell Signaling Technology | CST #9721; RRID:AB_330951 | WB (1:1000) |
Antibody | Anti-eIF2alpha (Rabbit polyclonal) | Cell Signaling Technology | CST #9722; RRID:AB_2230924 | WB (1:2000) |
Antibody | Anti-phospho-p70S6KT389 (Rabbit monoclonal) | Cell Signaling Technology | CST #9234; RRID:AB_2269803 | WB (1:1000) |
Antibody | Anti-p70S6K (Rabbit monoclonal) | Cell Signaling Technology | CST #2708; RRID:AB_390722 | WB (1:1000) |
Antibody | Anti-phospho-S6S240/244 (Rabbit monoclonal) | Cell Signaling Technology | CST #5364; RRID:AB_10694233 | IF (1:1000) |
Antibody | Anti-cleaved caspase 3 (Rabbit polyclonal) | Cell Signaling Technology | CST #9661; RRID:AB_2341188 | IF (1:100) |
Antibody | Anti-BrdU (Mouse monoclonal) | Dako/Agilent | M0744; RRID:AB_10013660 | IF (1:50) |
Antibody | Anti-Hbs1l (Rabbit polyclonal) | Proteintech | #10359–1-AP; RRID:AB_2114730 | WB (1:1000) |
Antibody | Anti-Pelo (Rabbit polyclonal) | Proteintech | #10582–1-AP; RRID:AB_2236833 | WB (1:2000) |
Antibody | Anti-Vinculin (Mouse monoclonal) | Sigma-Aldrich | V9131; RRID:AB_477629 | WB (1:20,000) |
Antibody | Anti-GAPDH (Rabbit monoclonal) | Cell Signaling Technology | CST #2118; RRID:AB_561053 | WB (1:10,000) |
Antibody | Anti-Ki67 (Rabbit Polyclonal) | Abcam | ab15580; RRID:AB_443209 | IF (1:100) |
Antibody | Anti-Olig2 (Rabbit monoclonal) | Abcam | ab109186; RRID:AB_10861310 | IF (1:200) |
Antibody | Anti-Lhx1/5 (Mouse monoclonal) | DSHB | #4F2-c; RRID:AB_531784 | IF (1:100) |
Antibody | Anti-NeuN (Mouse monoclonal) | Millipore | MAB377; RRID:AB_2298772 | IF (1:500) |
Antibody | Anti-Tbr1 (Rabbit polyclonal) | Millipore | AB9616; RRID:AB_2200223 | IF (1:1000) |
Antibody | Anti-Pax2 (Rabbit polyclonal) | Thermo Fisher Scientific | #71–6000; RRID:AB_2533990 | IF (1:50) |
Antibody | Anti-pH3 (Rabbit polyclonal) | Upstate, Millipore | #06–570; RRID:AB_310177 | IF (1:1000) |
Antibody | Anti-PCNA (Mouse monoclonal) | Invitrogen, Thermo Fisher Scientific | MA5-11358; RRID:AB_10982348 | IF (1:100) |
Chemical compound, drug | DNase I | Worthington | LS002139 | |
Chemical compound, drug | Bromodeoxyuridine (BrdU) | Sigma-Aldrich | B9285 | |
Chemical compound, drug | 5-Bromo-4-chloro-3-indolyl-β-D-galactopyranoside (X-Gal) | Sigma-Aldrich | B4252 | |
Chemical compound, drug | Z-4-hydroxytamoxifin (4OHT) | Sigma-Aldrich | H7905 | |
Commercial assay or kit | RNAscope Multiplex Fluorescent Reagent Kit v2 | Advanced Cell Diagnostics | #323100 | |
Commercial assay or kit | KAPA Stranded mRNA-seq. Kit | Roche | KR0960 | |
Commercial assay or kit | iQ SYBR Green Supermix | Bio-Rad | #1708880 | |
Commercial assay or kit | SuperScript III First-Strand Synthesis System | Invitrogen | #18080051 | |
Commercial assay or kit | DNA-free DNA Removal Kit | Life Technologies | AM1906 | |
Commercial assay or kit | TSA Plus Cyanine 3 | PerkinElmer | NEL744001KT | |
Other | RNA-seq. and ribosome profiling data | This study (see Materials and methods) | GSE162556 | Deposited Data |
Sequence-based reagent | Hbs1l GTC (Genotyping) | This study (see Materials and methods) | N/A | Common Forward:5’AGTCCAGGTGTTTCCTCACG3’; Wild type Reverse:5’CCCTGGCCTATTTTTGGTTT3’; GTC Reverse:5’TGTCCTCCAGTCTCCTCCAC3’ |
Sequence-based reagent | Hbs1l cKO (Genotyping) | This study (see Materials and methods) | N/A | Forward I: 5’CATGGCCTCCTATGGGTTGA3’; Forward II: 5’GCCTACAGTGAGCACAGAGT3’; Reverse: 5’TAGGTGCTGGGATTTGAACC3’ |
Sequence-based reagent | Pelo cKO (Genotyping) | This study (see Materials and methods) | N/A | Forward:5’TGTAACTGAACCCTGCAGTATCT3’; Reverse I: 5’GTGGAGCATGAAATGAAATTCGG3’; Reverse II: 5’ATCCAAGGCTTTTACTTCGCC3’ |
Sequence-based reagent | RNAscope probe Hbs1l-C2 | Advanced Cell Diagnostics | #527471-C2 | |
Sequence-based reagent | Hbs1l Exon 3–6 (RT-PCR) | This study (see Materials and methods) | N/A | Forward Primer:5’GAAATTGACCAAGCTCGCCTGTA3’; Reverse Primer:5’CTCAGAAGTTAAGCCAGGCACT3’ |
Sequence-based reagent | β-actin (RT-PCR) | Terrey et al., 2020 | N/A | Forward Primer:5’GGCTGTATTCCCCTCCATCG3’; Reverse Primer:5’CCAGTTGGTAACAATGCCATGT3’ |
Sequence-based reagent | Hbs1l Isoform I (quantitative RT-PCR) | This study (see Materials and methods) | N/A | Forward Primer:5’AGACCATGGGATTTGAAGTGC3’; Reverse Primer:5’CCGGTCTCAGGAATGTTAGGA3’ |
Sequence-based reagent | Hbs1l Isoform II (quantitative RT-PCR) | This study (see Materials and methods) | N/A | Forward Primer:5’TGAAGTTGAACAAAGTGCCAAG 3’; Reverse Primer:5’CTGCTTCCTCTGTGTTCCTC3’ |
Sequence-based reagent | Pelo (quantitative RT-PCR) | This study (see Materials and methods) | N/A | Forward Primer:5’CCCCAGGAAACGGAAAGGC3’; Reverse Primer:5’ACGCACTTTACAACCTCGAAG3’ |
Sequence-based reagent | Gapdh (quantitative RT-PCR) | Ishimura et al., 2016 | N/A | Forward Primer:5’CATTGTCATACCAGGAAATG3’; Reverse Primer:5’GGAGAAACCTGCCAAGTATG3’ |
Software, algorithm | Image J | NIH | RRID:SCR_003070; https://imagej.nih.gov/ij | |
Software, algorithm | GraphPad Prism 7 | GraphPad Prism | RRID:SCR_002798 | |
Software, algorithm | Pause site identification algorithm | Ishimura et al., 2014 | N/A | |
Software, algorithm | biomaRt version 2.42.1 | Durinck et al., 2005 | RRID:SCR_019214; https://bioconductor.org/packages/release/bioc/html/biomaRt.html | |
Software, algorithm | ShinyGO v0.61 (KEGG pathway) | Ge et al., 2020 | RRID:SCR_019213; http://bioinformatics.sdstate.edu/go | |
Software, algorithm | Ingenuity Pathway Analysis (IPA) | QIAGEN Inc | RRID:SCR_008653; https://www.qiagenbioinformatics.com/products/ingenuity-pathway-analysis | |
Software, algorithm | DESeq2 v1.26.0 | Love et al., 2014 | RRID:SCR_015687; https://bioconductor.org/packages/release/bioc/html/DESeq2.html | |
Software, algorithm | ensembldb v2.6.8 | Rainer et al., 2019 | RRID:SCR_019103; https://www.bioconductor.org/packages/release/bioc/html/ensembldb.html | |
Software, algorithm | riborex v2.3.4 | Li et al., 2017 | RRID:SCR_019104; https://github.com/smithlabcode/riborex | |
Software, algorithm | RiboWaltz v1.0.1 | Lauria et al., 2018 | RRID:SCR_016948; https://github.com/LabTranslationalArchitectomics/RiboWaltz | |
Software, algorithm | bowtie2 v 2.2.3 | Langmead and Salzberg, 2012 | RRID:SCR_005476; http://bowtie-bio.sourceforge.net/bowtie2/index.shtml | |
Software, algorithm | fastx_trimmer | Hannon Lab | http://hannonlab.cshl.edu/fastx_toolkit/ | |
Software, algorithm | fastx_clipper | Hannon Lab | http://hannonlab.cshl.edu/fastx_toolkit/ | |
Software, algorithm | hisat2 v2.1.0 | Kim et al., 2019 | RRID:SCR_015530;https://daehwankimlab.github.io/hisat2/ | |
Software, algorithm | featureCounts | Liao et al., 2014 | RRID:SCR_012919; http://bioinf.wehi.edu.au/featureCounts | |
Software, algorithm | sleuth v0.30.0 | Pimentel et al., 2017 | RRID:SCR_016883;https://pachterlab.github.io/sleuth/about | |
Software, algorithm | kallisto v0.42.4 | Bray et al., 2016 | RRID:SCR_016582;https://pachterlab.github.io/kallisto/about |
Additional files
-
Supplementary file 1
Embryonic lethality.
- https://cdn.elifesciences.org/articles/66904/elife-66904-supp1-v1.xlsx
-
Supplementary file 2
Locus specific pausing genome level.
- https://cdn.elifesciences.org/articles/66904/elife-66904-supp2-v1.xlsx
-
Supplementary file 3
DE RPF footprints.
- https://cdn.elifesciences.org/articles/66904/elife-66904-supp3-v1.xlsx
-
Supplementary file 4
A-site pausing.
- https://cdn.elifesciences.org/articles/66904/elife-66904-supp4-v1.xlsx
-
Supplementary file 5
Locus-specific pausing transcript level.
- https://cdn.elifesciences.org/articles/66904/elife-66904-supp5-v1.xlsx
-
Supplementary file 6
TE MEFs.
- https://cdn.elifesciences.org/articles/66904/elife-66904-supp6-v1.xlsx
-
Supplementary file 7
DE mRNA MEFs.
- https://cdn.elifesciences.org/articles/66904/elife-66904-supp7-v1.xlsx
-
Transparent reporting form
- https://cdn.elifesciences.org/articles/66904/elife-66904-transrepform-v1.pdf