Sec17/Sec18 can support membrane fusion without help from completion of SNARE zippering
Figures
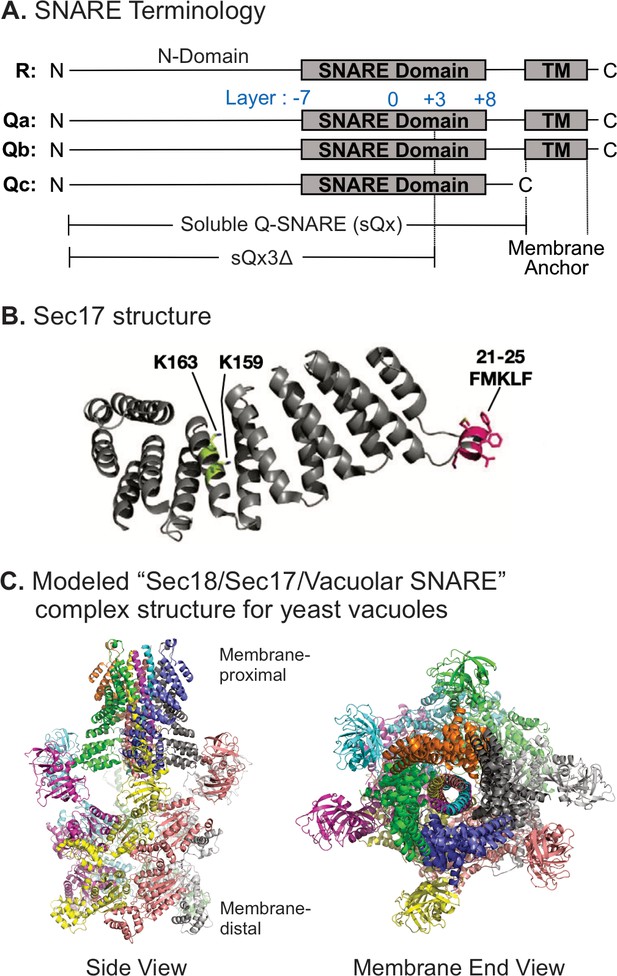
Model of the Sec18/Sec17/vacuolar SNARE complex and Sec17 mutations.
(A) Schematic of the four yeast vacuolar SNAREs, the soluble Q-SNAREs (sQx), and their deletion derivatives sQx3Δ lacking regions C-terminal to the +3 layer of the SNARE domain. (B) Structure of Sec17 (Rice and Brunger, 1999, PDB ID 1QQE). Residues mutated in certain experiments are highlighted as in Schwartz et al., 2017. (C) Modeling of the vacuolar Sec18/Sec17/SNARE complex. MODELLER (Webb and Sali, 2016) was used to create individual homology models of the vacuolar SNARE complex (Nyv1, Vam3, Vti1, Vam7) and of Sec18 starting from the coordinates of synaptobrevin-2, SNAP-25, syntaxin-1A, and NSF in the cryo-EM structure of the neuronal 20S complex (PDB ID 3J96) (Zhao et al., 2015). These homology models, together with the crystal structure of Sec17 (PDB ID 1QQE) (Rice and Brunger, 1999), were fit into the cryo-EM structure of the neuronal 20S complex (PDB ID 3J96) (Zhao et al., 2015). We used PDB ID 3J96 because this cryo-EM structure did not include the SNAP-25 linker and the Habc domain of syntaxin-1A. The vacuolar SNARE complex (Nyv1, Vam3, Vti1, Vam7) also does not include a linker between any of the SNARE motifs; in all structures of NSF/αSNAP/ternary SNARE complexes determined thus far, four αSNAP molecules are observed for SNARE complexes that do not contain a linker connecting two of the SNARE domains (White et al., 2018), and we therefore included four Sec17 molecules in our model of the vacuolar 20S complex. In the PDB coordinate file supplied as Source Data File of the homology model of the Sec18/Sec17/vacuolar SNARE complex, Sec18 molecules, chains A–F; Sec17, chains G–J; Nyv1, chain K; Vam3, chain L; Vti1, chain M; Vam7, chain N are included. Colors: cyan: Nyv1; magenta: Vam3; yellow: Vti1; salmon: Vam7; gray, orange, green, slate: Sec17; yellow, magenta, gray, blue, salmon, green: Sec18. Cartoon representations are shown. Two views related by a 90-degree rotation are included (left: side view; right: membrane-end view).
© 2017, Schwartz et al. Figure 1B reproduced from Figure 5A Schwartz et al., 2017, eLife, published under the Creative Commons Attribution 4.0 International Public License.
-
Figure 1—source data 1
Source data file (PDB) for Figure 1C.
- https://cdn.elifesciences.org/articles/67578/elife-67578-fig1-data1-v2.zip
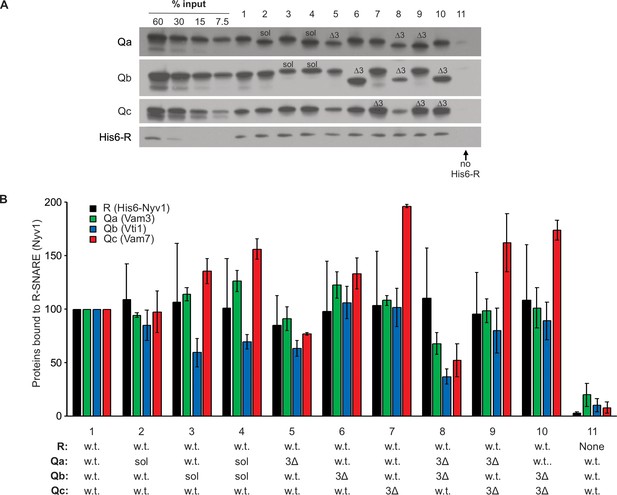
SNARE associations during partial zippering.
Purified full-length vacuolar SNAREs will spontaneously, though slowly, assemble into a parallel 4-helical coiled coil structure, which extends from the N-terminal (−7) layer of the SNARE domain to the C-terminal (+8) end of the SNARE domain. While the association of neuronal SNAREs has been studied extensively, the association of full-length or truncated vacuole SNAREs has received less study. Full-length R-SNARE, with a 6his-tag on its N-terminus, was mixed in octylglucoside with full-length Q-SNAREs, with soluble Q-SNAREs (sQ), or with sQ3Δs. Stable spontaneous complexes were isolated with nickel-NTA beads and examined by immunoblot. Complex formation with full-length SNAREs (lane 1) was not blocked by the absence of transmembrane domain from Qa, Qb, or both (lanes 2–4) or by the 3Δ deletion in the C-terminal end of the SNARE domain in any one or two Q-SNAREs (lanes 5–10). (A) Reactions (50 µl) containing 2.5 µM of each SNARE in Buffer 151 (20 mM HEPES-NaOH, pH 7.4, 150 mM NaCl, 10% glycerol, 1% ß-OG, 20mM imidazole) were nutated at 4°C for 2 hr. A 40 µl portion of each was transferred to a 0.5 ml Eppendorf tube containing 20 µl of a 50% slurry of Qiagen Nickel-NTA Agarose in Buffer 151. Suspensions were nutated at 4°C for 1 hr. Loosely bound proteins were removed by four successive suspensions in 0.5 ml Buffer 151, each followed by centrifugation (500xg, 6 min, 4°C) and removal of the supernatant. Bound proteins were eluted by boiling the agarose in 50 µl of sodium dodecyl sulphate (SDS) sample buffer (50 mM TrisCl, pH 6.8, 5% glycerol, 2% SDS, 0.2% bromophenol blue, 1% ß-mercaptoethanol) for 5 min. Samples were analyzed by SDS-PAGE followed by immunoblotting. (B) Western blots of three identical experiments were scanned and analyzed with UN-SCAN-IT Software (Silk Scientific, Orem, UT). Average pixel values were set at 100 for the all-wild-type positive control (lane 1). Means and standard deviations are shown.
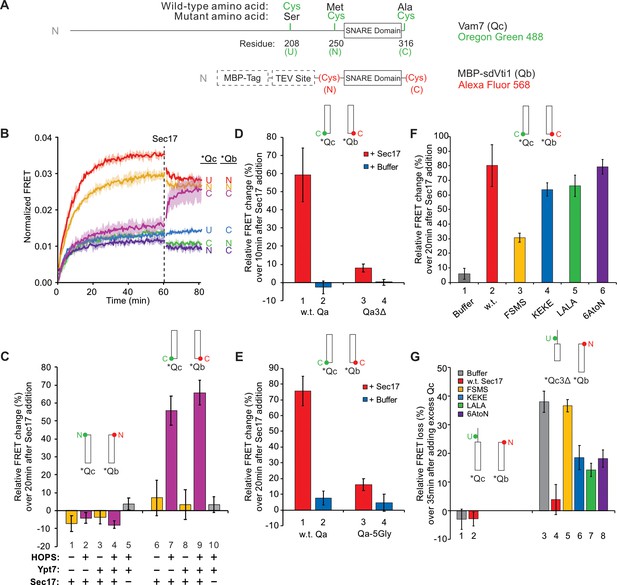
Sec17 modifies SNARE conformation in a HOPS- and zippering-dependent manner and stabilizes complexes with truncated SNARE domains.
(A) Schematic of fluorescently labeled SNARE constructs used in this study. SNAREs were derivatized as described previously (Torng et al., 2020). Wild-type Qc contains a single Cys residue at 208 at the upstream (U) position. (N)- and (C)-labeled constructs replace residues 250 and 316 with Cys, while also replacing Cys208 with Ser. Each Qc construct was derivatized with Oregon Green 488 as described (Torng et al., 2020). A fusion of maltose-binding protein (MBP), a TEV site, and the Qb SNARE domain (residues 133–187) was expressed with an added cysteinyl residue immediately upstream or downstream of the SNARE domain. Each Qb construct was derivatized with Alexa Fluor 568. Qc and Qb labeled with a fluorescent probe at any position are written as *Qc and *Qb. (B–G) Bar graphs are reported as the mean of the relative ensemble fluorescence resonance energy transfer (FRET) change (%) per trial with propagated standard deviation for n = 3 trials. The relative change was calculated by averaging 10 data points, each from just before Sec17 addition and from the end of the measurement period 20 min later, except where indicated. Specific time points used as well as the statistics for the propagation of uncertainty are shown in Supplementary Data. A bar graph representation for (B) and the kinetic curves for (C–G) are provided in Figure 2—figure supplement 1 . (B) Sec17 modifies the conformation of the C-terminus of the SNARE complex. Ypt7/RQa proteoliposomes were incubated with pairs of *Qb and *Qc labeled at the N, C, or upstream (U) locations as indicated in (A). Curves are averages of n = 3 trials, and the shaded regions behind each curve show the standard deviation per time point. (C) Sec17-promoted zippering requires HOPS. RQa proteoliposomes were incubated with either the N-labeled *Qb/*Qc pair (left) or the C-labeled *Qb/*Qc pair (right), with Ypt7 and HOPS as indicated. A reaction with a buffer (RB150) added instead of Sec17 serves as a negative control. (D) Sec17 does not promote C-terminal zippering if the SNARE domain of Qa is truncated. Ypt7/R proteoliposomes were incubated with C-terminally labeled *Qb and *Qc and either soluble Qa or Qa3Δ, and the relative FRET change was calculated over 10 min after Sec17 or buffer addition. (E) Sec17-induced zippering requires the apolar heptad-repeat amino acyl residues in Qa SNARE domain layers +4 to +8. Proteoliposomes bearing Ypt7, R-SNARE, and either wild-type Qa or Qa with the +4 to +8 layers inwardly-oriented apolar residues mutated to Gly were incubated with C-terminally labeled *Qb and *Qc, and then Sec17 or its mutants were added at t = 60 min. (F) Sec17-driven SNARE conformational change is stunted by the F22SM23S mutation of Sec17 (FSMS). Ypt/R proteoliposomes were incubated with sQa and C-terminally labeled *Qb and *Qc, and then Sec17 or mutants as indicated were added at t = 60 min. (G) Sec17 stabilizes incompletely zippered SNARE complexes. Ypt7/R proteoliposomes were incubated with sQa and C-terminally labeled *Qb and *Qc. Sec17 or the indicated mutants were added at t = 60 min. Non-fluorescent Qc (8.5 µM) was added at t = 80 min, and the loss of FRET over 35 min was measured starting immediately after the addition of non-fluorescent Qc.
-
Figure 2—source data 1
Source data file (Excel) for Figure 2B.
- https://cdn.elifesciences.org/articles/67578/elife-67578-fig2-data1-v2.xlsx
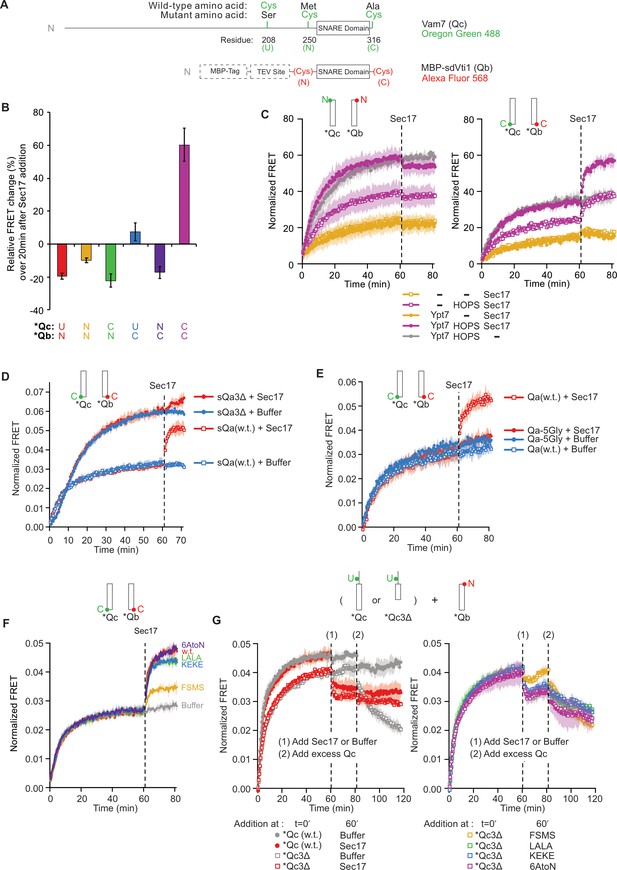
Statistics and representative kinetic data for Figure 2.
(A) Schematic of fluorescently labeled SNARE constructs used in this study. (B–G) Supplementary data for panels (B-G) in Figure 2. Subfigures are presented with the same subfigure labels and matching colors. All kinetic data are averages of three trials, and the shaded regions behind each curve show the standard deviation per time point. (B) The effect of Sec17 on SNARE average ensemble fluorescence resonance energy transfer (FRET) efficiency as a function of fluorophore position. The mean and standard deviation of three trials is shown for each reaction. (C) Representative kinetic data showing the effect of HOPS and Ypt7 on Sec17-induced zippering. Data for the N-terminally labeled *Qb/*Qc pair is shown on the left, and data for the C-terminally labeled pair is shown on the right. Reactions with HOPS are in purple, and reactions without HOPS are in orange. As a negative control, a buffer (RB150) was added instead of Sec17 for the reaction in gray. (D) Representative kinetic data showing that Sec17 has only minor effect on the average FRET efficiency of SNARE complexes bearing truncated Qa SNARE. (E) Representative kinetic data showing that Sec17 does not affect the average FRET efficiency of SNARE complexes bearing Qa SNARE with a mutated C-terminal half. (F) Representative kinetic data for the ability of Sec17 or mutants to promote C-terminal zippering of the SNARE complex. (G) Representative kinetic data showing that Sec17 stabilizes incompletely zippered SNARE complexes. Sec17, mutants, or buffer was added at t = 60 min (1), and non-fluorescent Qc was added at t = 80 min (2). Reactions containing wild-type Qc are shown with filled circles, and reactions with Qc3Δ are shown with open squares. The effect of wild-type Sec17 (red) is contrasted with no Sec17 (gray) in the left-side graph, and the four Sec17 mutants used in this experiment are compared in the right-side graph.
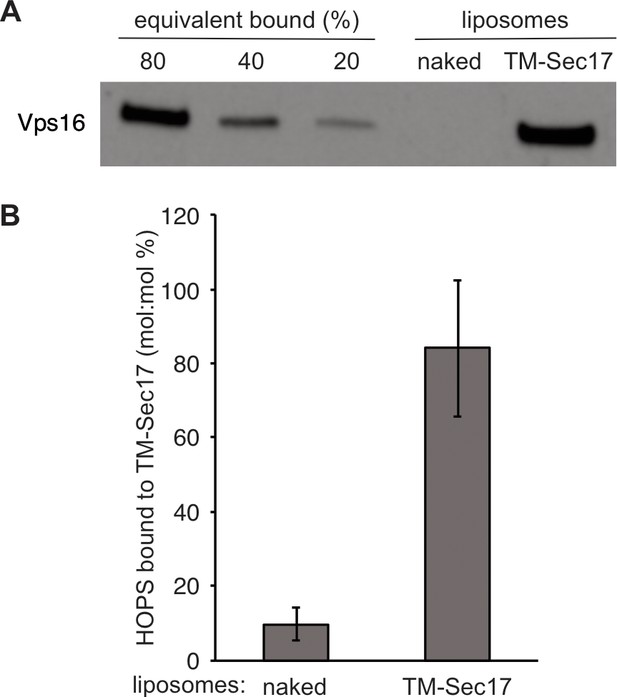
Direct binding of Sec17 to HOPS.
gvSince proteoliposome fusion promoted by synthetic tethers is inhibited by all concentrations of Sec17, whereas HOPS-dependent fusion is only inhibited by very high Sec17 levels (Song and Wickner, 2019) and Sec17-triggered zippering requires HOPS (Figure 2C), we tested whether HOPS might directly associate with Sec17. Phosphatidylcholine liposomes were prepared without protein (‘naked’) or with TM-Sec17, a recombinant form of Sec17 with an N-terminal apolar transmembrane anchor (Song et al., 2017). These liposomes were incubated with HOPS, then floated through a density gradient. HOPS only bound in the presence of anchored Sec17, showing a direct and nearly stoichiometric binding between these proteins. (A) Liposomes composed of PC/PS/NBD (83.5/15/1.5%) were prepared from mixed micellar solutions by detergent dialysis as described in 'Materials and methods'. Liposomes bore either no proteins or TM-Sec17 (Song et al., 2017) at a 1:4000 protein to lipid molar ratio. Floatation assays were conducted as described in Song et al., 2020) (Figure 2). In brief, 30 μl reactions in RB150 contained 0.5 mM liposomal lipid, 125 nM integral TM-Sec17 where present, 0.2% defatted bovine serum albumin (BSA), 1 mM MgCl2, and 500 nM HOPS. Reactions were incubated (1 hr, 30°C) and liposomes floated through Histodenz (Sigma, St Louis, MO) density medium. Collected samples were solubilized in 0.125% Thesit, adjusted for lipid recovered, and analyzed for bound proteins by immunoblot for Vps16, a HOPS subunit. Because the reaction contained four times more HOPS than integrally bound TM-Sec17, we express the standard curve as the percent of molar equivalence (to total liposomal TM-Sec17) of HOPS. (B) Western blots from three repetitions of this experiment were analyzed by UN-SCAN-IT Software with standard curves. Mean values and standard deviations are shown.
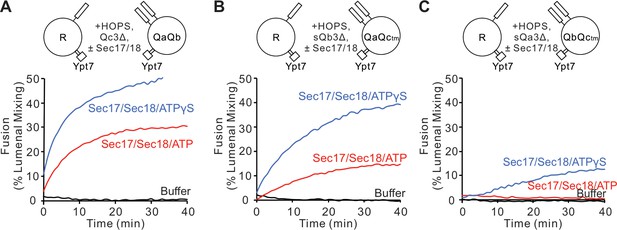
Fusion is blocked by deletion of the last five C-terminal SNARE domain layers from any single Q-SNARE and is restored by Sec17, Sec18, and ATP or ATPγS.
(A) Fusion incubations, as described in 'Materials and methods', had Ypt7/R and Ypt7/QaQb proteoliposomes (1:8000 Ypt7:lipid molar ratio, 1:16,000 SNARE:lipid molar ratio), 2 μM Qc3Δ, and, where indicated, 600 nM Sec17, 300 nM Sec18, 1 mM ATP (red), or ATPγS (blue). (B) Fusion with 2 μM sQb3Δ and with Ypt7/QaQc-tm proteoliposomes, but otherwise as in (A). (C) Fusion with 2 μM sQa3Δ and with Ypt7/QbQc-tm proteoliposomes, but otherwise as in (A). Mean and standard deviations from three independent experiments are shown in Figure 3—figure supplement 1.
-
Figure 3—source data 1
Source data file (Excel) for Figure 3A,B and C.
- https://cdn.elifesciences.org/articles/67578/elife-67578-fig3-data1-v2.xlsx
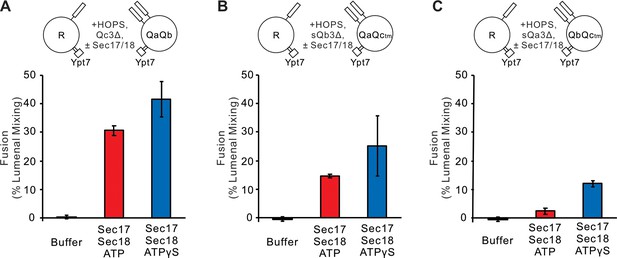
Statistics for Figure 3.
Fusion blocked by deletion of the C-terminal five layers of any Q-SNARE domain is restored by Sec17 and Sec18. (A) Ypt7/R- and Ypt7/QaQb, (B) Ypt7/R- and Ypt7/QaQc-tm, and (C) Ypt7/R- and Ypt7/QbQc-tm. The experiment in Figure 3 was repeated in triplicate; mean values and standard deviations for fusion after 30 min are shown.
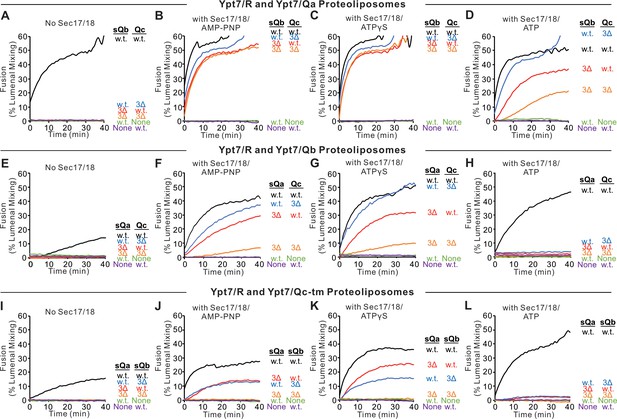
Fusion with single membrane-anchored Q-SNAREs.
(A–D) Fusion incubations, as described in 'Materials and methods', had Ypt7/R and Ypt7/Qa proteoliposomes (1:8000 Ypt7-TM:lipid molar ratio, 1:16,000 SNARE:lipid molar ratio), 50 nM HOPS, 2 μM sQb or sQb3Δ, 2 μM Qc or Qc3Δ, and, where indicated, 600 nM Sec17, 300 nM Sec18, and 1 mM ATP, AMP-PNP, or ATPγS. (E–H) Fusion incubations as for (A), but with Ypt7/Qb proteoliposomes and 2 μM sQa or sQa3Δ, 2 μM Qc or Qc3Δ and Sec17, Sec18, and adenine nucleotide as indicated. (I–L) Fusion incubations as for (A), but with Ypt7/Qc-tm proteoliposomes and 2 μM sQa or sQa3Δ, 2 μM Qb or sQb3Δ and Sec17, Sec18, and adenine nucleotide as indicated. Mean and standard deviations from more than three independent experiments are shown in Figure 4—figure supplement 1.
-
Figure 4—source data 1
Source data file (Excel) for Figure 4A–L.
- https://cdn.elifesciences.org/articles/67578/elife-67578-fig4-data1-v2.xlsx
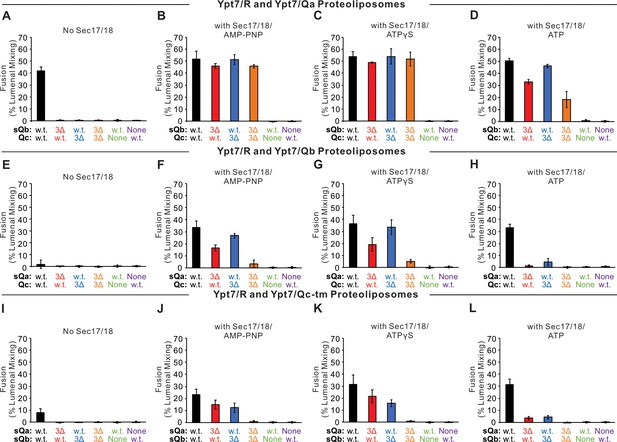
Statistics data for Figure 4.
Fusion between Ypt7/R and (A–D) Ypt/Qa, (E–H) Ypt7/Qb, or (I–L) Ypt7/Qc-tm proteoliposomes. Fusion assays are described in Figure 4, repeated more than three times. Mean values and standard deviations for fusion after 20 min are shown.
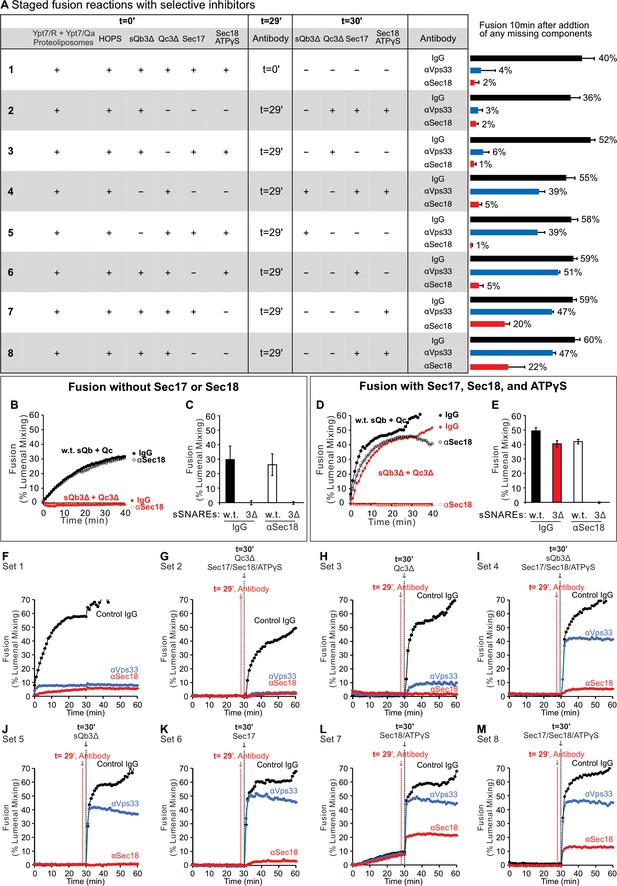
Selective inhibitors reveal successive fusion intermediates.
Ypt7/R and Ypt7/Qa proteoliposomes were co-incubated in the presence of HOPS and other fusion proteins or inhibitors, allowing resolution of an early, HOPS-dependent reaction stage from late, Sec18-dependent fusion. Incubations were in sets of three, with either of the three antibody preparations. When HOPS, Ypt7/R and Ypt7/Qa proteoliposomes were mixed with sQb3Δ, Qc3Δ, Sec17, Sec18, and ATPγS plus control IgG or specific antibody from the start of the incubation, fusion was blocked by affinity-purified antibody to either Sec18 (Haas and Wickner, 1996) or Vps33 (Seals et al., 2000), the SM subunit of HOPS (A, Set 1). In each set, any of these components not present in the initial incubation was added after 30 min. When the proteoliposomes were incubated for 29 min with HOPS, sQb3Δ, and Qc3Δ prior to antibody addition, followed by Sec17, Sec18, and ATPγS 1 min later, the restored fusion remained sensitive to each affinity-purified antibody (Set 2). If, however, the initial 29 min incubation also bore Qc3Δ, alone (Set 4) or in combination with other proteins (Sets 5–8), the restored fusion had acquired resistance to antibody to Vps33 prior to addition of the missing fusion proteins. Incubation with sQb3Δ did not confer resistance to anti-Vps33 (Set 3). Sec17 and Sec18 are not needed to form a reaction intermediate, which is resistant to antibody to Vps33 (Set 4). In contrast, when fusion reactions were blocked by the single omission of either sQb3Δ (Set 5) or Qc3Δ (Set 3), any intermediates that formed were not resistant to antibody to Sec18. A very partial resistance was seen when all the four SNAREs were present during the initial 29 min incubation (Sets 7 and 8). The specificity of inhibition by antibody to Sec18 was confirmed by its failure to inhibit Sec18-independent fusion with full-length sQb and Qc (B and C), but there was complete inhibition of Sec18-dependent fusion reactions with sQb3Δ and Qc3Δ (D and E, red). Sec18 thus acts late in the fusion pathway, as incubations of the proteoliposomes with HOPS and Qc3Δ, which confer fusion-resistance to antibody to Vps33 (Set 4), remain sensitive to antibody to Sec18. (A) Proteoliposomes with Ypt7/R and proteoliposomes with Ypt7/Qa (1:8000 Ypt7-TM:lipid molar ratio, 1:16,000 SNARE:lipid molar ratio) were mixed with 50 nM HOPS at t = 0. At either t = 0 or at t = 30, indicated subsets of 2 μM sQb3Δ, 2 μM Qc3Δ, 400 nM Sec17, 300 nM Sec18, and 1 mM ATPγS were added to the incubations. To block Sec18 or Vps33, affinity-purified antibody (1 μg) to each was added at t = 0 (sample 1) or at t = 29 (samples 2–8). As a control, 1 μg IgG was added to separate samples as indicated. The average and standard deviations of fusion 10 min after addition of any missing components are shown from three independent experiments. (B–E) Sec18 antibody or control IgG inhibition experiments were performed with Ypt7/R and Ypt7/Qa proteoliposomes with 50 nM HOPS, 2 μM sQb and 2 μM Qc (black curves) or 2 μM sQb3Δ and 2 μM Qc3Δ (red curves), 1 μg IgG (filled circles) or αSec18 (open circles), and without (B and C) or with (D and E) 400 nM Sec17, 300 nM Sec18, and 1 mM ATPγS as indicated. Kinetics shown are representative of four experiments. Mean and standard deviations from four independent experiments are shown. (F–M) Representative experiments from (A), Sets 1–8, are shown.
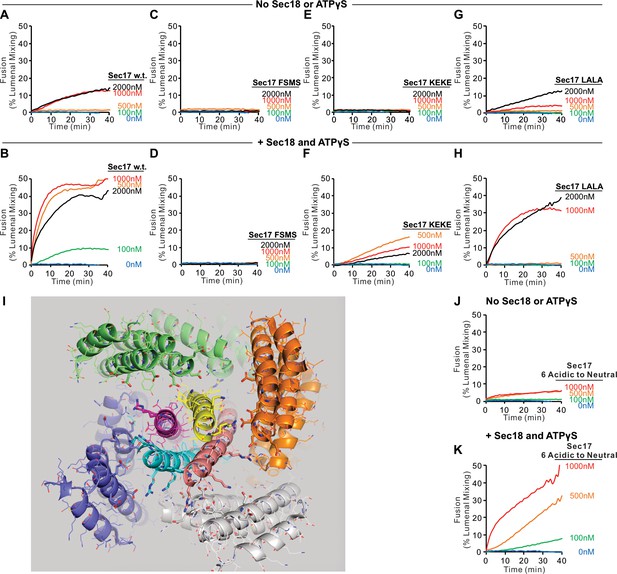
Role of each domain of Sec17 in zippering bypass fusion.
(A-H, J, K) Fusion between Ypt7/R and Ypt7/Qa proteoliposomes (1:8000 Ypt7-TM:lipid and 1:16,000 SNARE/lipid molar ratios) was assayed with 50 nM HOPS, 2 μM sQb3Δ, 2 μM Qc3Δ, the indicated concentration of wild-type or mutant Sec17, and with or without 250 nM Sec18 and 1 mM ATPγS. (I) Ionic interactions between Sec17s and vacuolar SNAREs in the +4 to +8 layers in the model of the Sec18/Sec17/vacuolar SNARE complex (Figure 1). Colors: cyan: R; magenta: Qa; yellow: Qb; salmon: Qc; gray, orange, green, slate: Sec17; red: oxygen atoms; blue: nitrogen atoms. Cartoon representations are shown along with side chains shown as thin lines. Thick lines (sticks) are interacting glutamate and aspartate (acidic) residues on the surface of Sec17 (aminoacyl residues 34, 35, 38, 73, 74, 75) that interact with the vacuolar SNARE complex lysine and arginine basic residues in each of the four SNAREs. Mean and standard deviations from four independent experiments are shown in Figure 5—figure supplement 1.
-
Figure 5—source data 1
Source data file (Excel) for Figure 5A–H,J and K.
- https://cdn.elifesciences.org/articles/67578/elife-67578-fig5-data1-v2.xlsx
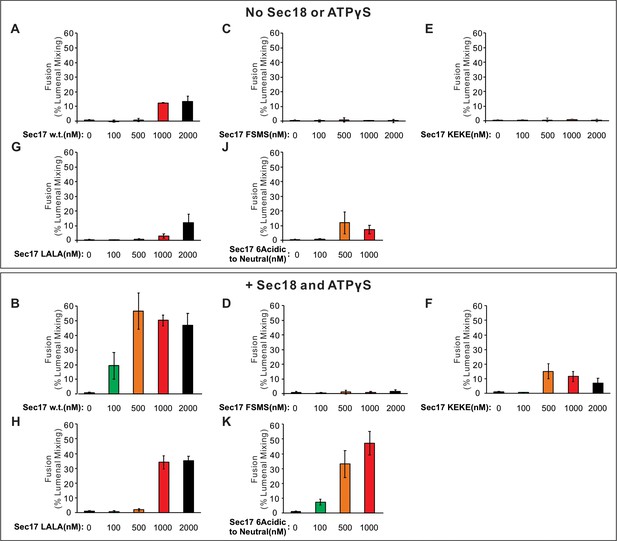
Mean and standard deviations of fusion after 60 min from four independent assays as in Figure 5 are shown.
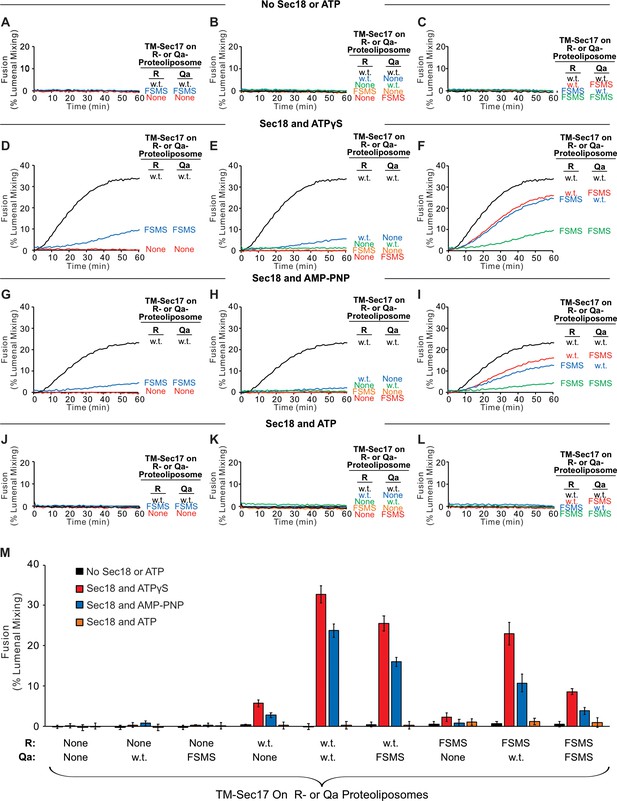
Sec18 does not simply promote fusion by contributing to the affinity of Sec17 for membranes that bear SNARE complexes.
We prepared proteoliposomes with Ypt7, with R or Qa, and with either no Sec17, with an equimolar (to SNAREs) TM-Sec17 that bears an N-terminal hydrophobic transmembrane anchor domain derived from Qb, or with TM-Sec17-F21S,M22S (TM-FSMS hereafter). In the presence of HOPS, sQb3Δ, and Qc3Δ, membrane-anchored Sec17 supported zippering bypass fusion, which was strictly Sec18-dependent (A–C, no Sec18; D–F, with Sec18 and ATPγS). TM-Sec17 on both sets of proteoliposomes supported fusion (D and G, black), but fusion was not seen in the absence of TM-Sec17 (D and G, red), and only limited fusion was seen with TM-Sec17-FSMS (D and G, blue). Fusion was greatly diminished if TM-Sec17 or TM-Sec17-FSMS was only present on one of the fusion partner proteoliposomes (E and H), but there was substantial fusion if one of the proteoliposomes had TM-Sec17 and one had TM-Sec17-FSMS (F and I). The simplest model is that several Sec17 molecules are required to form the Sec17 assembly around the SNAREs but that the hydrophobic Sec17 N-domain loop is only necessary on one membrane for fusion. AMP-PNP functioned as well as ATPγS (compare G–I to D–F), but hydrolyzable ATP completely blocked fusion with membrane-anchored TM-Sec17 (J–L), similar to the lower activity of hydrolyzable ATP with wild-type Sec17 (Figure 4), reflecting the proofreading activity of Sec17/Sec18. (M) Statistics data for (A–L). Fusion reactions had the indicated proteoliposomes, 50 nM HOPS, 2 μM each of sQb3Δ and Qc3Δ, and either Sec18 buffer (black), 1 mM ATPγS and Sec18 (red), 1 mM AMP-PNP and Sec18 (blue), or 1 mM ATP and Sec18 (orange). Experiments were repeated in quadruplicate; mean values and standard deviations for fusion after 60 min are shown.
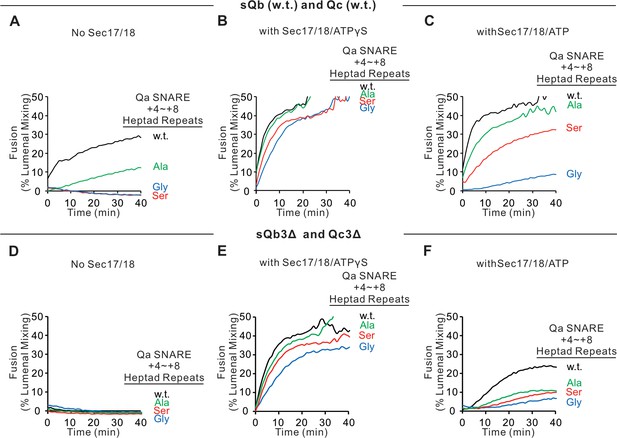
Sec17, Sec18, and ATPγS restore fusion to Ypt7/R and Ypt7/Qa proteoliposomes, which were triply-crippled from completion of SNARE domain zippering by deletion of the +4 to +8 layers of the sQb and Qc SNAREs and by substitution of the apolar residues of the +4 to +8 layers of Qa, substituting Ala, Ser, or Gly for L238, M242, A245, L249, and A252.
Fusion incubations ('Materials and methods') had (A–C) Ypt7/R and Ypt7/Qa (w.t. (black), Gly mutant (blue), Ser mutant (red), or Ala mutant (green)) proteoliposomes (1:8000 Ypt7-TM:lipid molar ratio, 1:16,000 SNARE:lipid molar ratio), 50 nM HOPS, 2 μM sQb (w.t.), and 2 μM Qc (w.t.) (A–C) or (D–F) 2 μM Qc3Δ and 2 μM sQb3Δ. Sec17 and Sec18 buffers (A and D) or 600 nM Sec17, 300 nM Sec18, and 1 mM ATPγS (B and E) or ATP (C and F) were also present. Kinetics shown are representative of four experiments. Mean and standard deviations from four independent experiments are shown in Figure 6—figure supplement 1.
-
Figure 6—source data 1
Source data file (Excel) for Figure 6A–F.
- https://cdn.elifesciences.org/articles/67578/elife-67578-fig6-data1-v2.xlsx
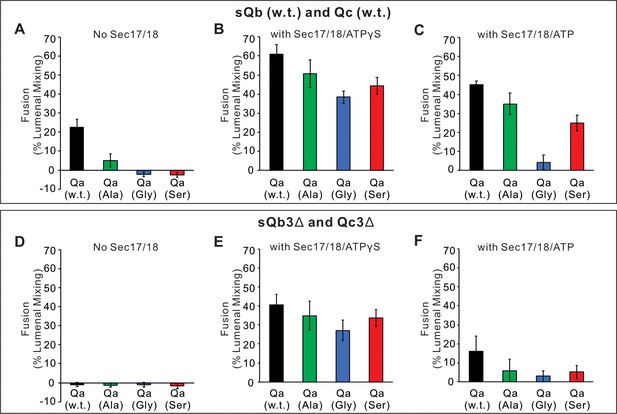
Fusion assays between Ypt7/R- and Ypt7/Qa with Qa w.t. (black), Qa 5Ala mutant (green), Qa 5Gly mutant (blue), or Qa 5Ser mutant (red) as described in Figure 6.
(A–C) Fusion with 2 μM sQb and 2 μM Qc. (D–F) Fusion with 2 μM sQb3Δ and 2 μM Qc3Δ. Experiments were repeated in quadruplicate; mean values and standard deviations for fusion after 30 min are shown.
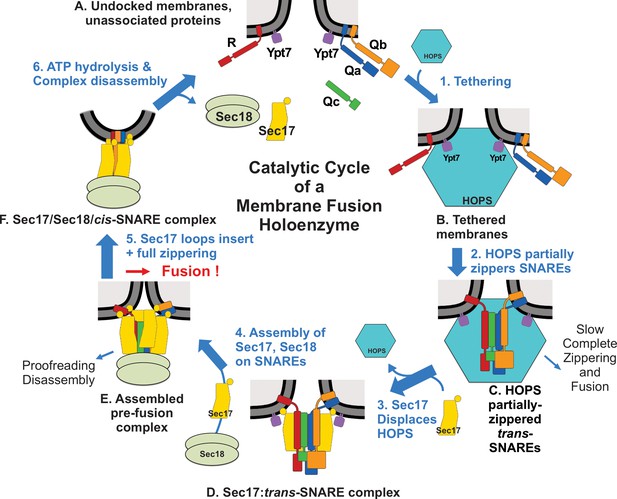
Current working model.
Catalyzed tethering and SNARE assembly (A–C) is followed by HOPS displacement by Sec17 (C and D) and further assembly of Sec17 and Sec18 on the partially zippered SNARE complex, promoting completion of zippering and apolar Sec17 loop insertion (D–F), both of which promote fusion. See text for discussion.
Tables
Reagent type (species) or resource | Designation | Source or reference | Identifiers | Additional information |
---|---|---|---|---|
Gene (Saccharomyces cerevisiae) | Nyv1 | Saccharomyces Genome Database | SGD:S000004083 | |
Gene (Saccharomyces cerevisiae) | Vam3 | Saccharomyces Genome Database | SGD:S000005632 | |
Gene (Saccharomyces cerevisiae) | Vti1 | Saccharomyces Genome Database | SGD:S000004810 | |
Gene (Saccharomyces cerevisiae) | Vam7 | Saccharomyces Genome Database | SGD:S000003180 | |
Gene (Saccharomyces cerevisiae) | Ypt7 | Saccharomyces Genome Database | SGD:S000004460 | |
Gene (Saccharomyces cerevisiae) | Sec17 | Saccharomyces Genome Database | SGD:S000000146 | |
Gene (Saccharomyces cerevisiae) | Sec18 | Saccharomyces Genome Database | SGD:S000000284 | |
Gene (Saccharomyces cerevisiae) | Vps33 | Saccharomyces Genome Database | SGD:S000004388 | |
Gene (Saccharomyces cerevisiae) | Vps39 | Saccharomyces Genome Database | SGD:S000002235 | |
Gene (Saccharomyces cerevisiae) | Vps41 | Saccharomyces Genome Database | SGD:S000002487 | |
Gene (Saccharomyces cerevisiae) | Vps16 | Saccharomyces Genome Database | SGD:S000005966 | |
Gene (Saccharomyces cerevisiae) | Vps11 | Saccharomyces Genome Database | SGD:S000004844 | |
Gene (Saccharomyces cerevisiae) | Vps18 | Saccharomyces Genome Database | SGD:S000004138 | |
Peptide, recombinant protein | GST-R (Nyv1) | PMID:18650938 | Purified from E. coli. | |
Peptide, recombinant protein | GST-Qa (Vam3) | PMID:18650938 | Purified from E. coli. | |
Peptide, recombinant protein | GST-Qb (Vti1) | PMID:18650938 | Purified from E. coli. | |
Peptide, recombinant protein | His-R (Nyv1) | PMID:22174414 | Purified from E. coli. | |
Peptide, recombinant protein | GST-sQa (soluble) | PMID:28637767 | Purified from E. coli. | |
Peptide, recombinant protein | MBP-sQb (soluble) | PMID:24088569 | Purified from E. coli. | |
Peptide, recombinant protein | GST-Qb (Vti1)3Δ | This study | Purified from E. coli. | |
Peptide, recombinant protein | GST-Qa (Vam3)3Δ | This study | Purified from E. coli. | |
Peptide, recombinant protein | Qc (Vam7) | PMID:17699614 | Purified from E. coli. | |
Peptide, recombinant protein | Qc (Vam7)-tm | PMID:23071309 | Purified from E. coli. | |
Peptide, recombinant protein | Qc (Vam7) C208S, M250C | This study | Purified from E. coli. | |
Peptide, recombinant protein | Qc (Vam7) C208S, A316C | This study | Purified from E. coli. | |
Peptide, recombinant protein | MBP-Cys-sQb (SNARE domain) | PMID:28637767 | Purified from E. coli. | |
Peptide, recombinant protein | MBP-Cys-sQb (SNARE domain) | PMID:28637767 | Purified from E. coli. | |
Peptide, recombinant protein | Ypt7 | PMID:24088569 | Purified from E. coli. | |
Peptide, recombinant protein | Ypt7-TM | PMID:31235584 | Purified from E. coli. | |
Peptide, recombinant protein | Sec17 | PMID:19414611 | Purified from E. coli. | |
Peptide, recombinant protein | Sec17 FSMS | PMID:28925353 | Purified from E. coli. | |
Peptide, recombinant protein | Sec17 KEKE | PMID:28925353 | Purified from E. coli. | |
Peptide, recombinant protein | Sec17 LALA | PMID:19414611 | Purified from E. coli. | |
Peptide, recombinant protein | GST-Sec17 6AtoN | This study | Purified from E. coli. | |
Peptide, recombinant protein | GST-Sec17-TM | PMID:28718762 | Purified from E. coli. | |
Peptide, recombinant protein | GST-Sec17-TM FSMS | PMID:28718762 | Purified from E. coli. | |
Peptide, recombinant protein | TEV protease | PMID:18007597 | Purified from E. coli. | |
Peptide, recombinant protein | HOPS | PMID:18385512 | Purified from Saccharomyces cerevisiae. | |
Antibody | Anti-Vam3 (rabbit polyclonal) | PMID:12566429 | Wickner lab stock | WB: 1:2000 |
Antibody | Anti-Nyv1 (rabbit polyclonal) | PMID:10385523 | Wickner lab stock | WB: 0.65 μg/ml |
Antibody | Anti-Vti1 (rabbit polyclonal) | PMID:18007597 | Wickner lab stock | WB: 0.47 μg/ml |
Antibody | Anti-Vam7 (rabbit polyclonal) | PMID:14734531 | Wickner lab stock | WB: 0.1 μg/ml |
Antibody | Anti-Vps16 (rabbit polyclonal) | PMID:18007597 | Wickner lab stock | WB: 1 μg/ml |
Antibody | Anti-Vps33 (rabbit polyclonal) | PMID:10944212 | Wickner lab stock | Inhibition assay: 1 μg |
Antibody | Anti-Sec18 (rabbit polyclonal) | PMID:11483507 | Wickner lab stock | Inhibition assay: 1 μg |
Chemical compound, drug | Cy5-derivatized streptavidin | SeraCare Life Sciences | 5270–0023 | |
Chemical compound, drug | Biotinylated PhycoE | Thermo Fisher Scientific | p811 | |
Chemical compound, drug | Streptavidin | Thermo Fisher Scientific | 434302 | |
Chemical compound, drug | 1,2-dilinoleoyl-sn-glycero-3-phosphocholine | Avanti polar lipids | 850385 | |
Chemical compound, drug | 1,2-dilinoleoyl-sn-glycero-3-phospho-L-serine | Avanti polar lipids | 840040 | |
Chemical compound, drug | 1,2-dilinoleoyl-sn-glycero-3-phosphoethanolamine | Avanti polar lipids | 850755 | |
Chemical compound, drug | 1,2-dilinoleoyl-sn-glycero-3-phosphate | Avanti polar lipids | 840885 | |
Chemical compound, drug | L-α-phosphatidylinositol | Avanti polar lipids | 840044 | |
Chemical compound, drug | 1,2-dipalmitoyl-sn-glycerol | Avanti polar lipids | 800816 | |
Chemical compound, drug | Ergosterol | Sigma | 45480 | |
Chemical compound, drug | PI(3)P | Echelon Bioscience | P-3016 | |
Chemical compound, drug | Rhodamine DHPE | Invitrogen | L1392 | |
Chemical compound, drug | NBD-PE | Invitrogen | N360 | |
Chemical compound,drug | Marina-blue | Invitrogen | M12652 | |
Software and algorithms | UN-SCAN-IT | Silk Scientific | ||
Chemical compound, drug | Alexa Fluor 568 C5-maleimide | Thermo Fisher Scientific | A20341 | |
Chemical compound, drug | Oregon Green 488 Maleimide | Thermo Fisher Scientific | O6034 | |
Chemical compound, drug | Oregon Green 488 Maleimide | Thermo Fisher Scientific | O6034 | |
Chemical compound, drug | Pierce TCEP-HCl | Thermo Fisher Scientific | 20490 | |
Chemical compound, drug | L-cysteine | Sigma-Aldrich | 30089 |