Ripples reflect a spectrum of synchronous spiking activity in human anterior temporal lobe
Figures
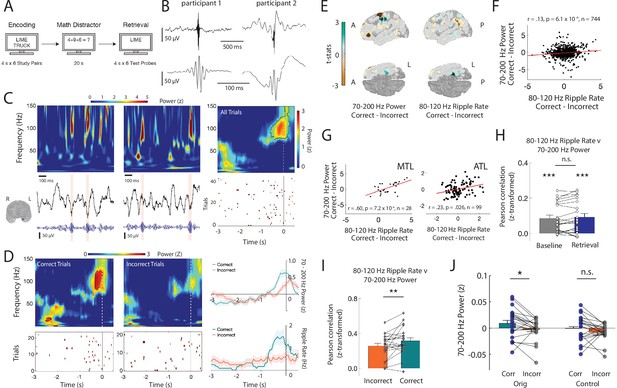
High-frequency activity reflects discrete ripples.
(A) Paired-associates verbal episodic memory task. (B) Average iEEG signal locked to detected ripples in an anterior temporal lobe electrode in two participants. (C) Time-frequency power spectrograms for two clips of iEEG data from one electrode in medial temporal lobe (MTL) with corresponding iEEG voltage signal (black), 80–120 Hz band signal (blue), and detected ripple events (shaded). Location of the representative channel is shown. Trial-averaged power spectrogram of the single channel in medial temporal lobe during retrieval (top right) and corresponding spike raster of iEEG ripples across trials prior to vocalization (bottom right). Black contour indicates significant clusters (cluster-based permutation, p < .01). (D) Trial-averaged power spectrograms and corresponding ripple raster plots for correct and incorrect retrieval. Average 70–200 Hz power time series (top right) and average ripple rate time series (bottom right) for correct and incorrect retrieval. Black contour indicates significant clusters (cluster-based permutation, p < .01). (E) Cortical topographic plots of difference in 70-200 Hz power and 80-120 Hz ripple rate between correct and incorrect memory retrieval. Each data point reflects the across-participant t-statistic for a region of interest (ROI). (F) Pearson correlation between 70-200 Hz power and 80-120 Hz ripple rate across all ROIs. Each data point represents the average across participants for each ROI. Line represents the least-squares regression. (G) Pearson correlation between 70–200 Hz power and 80–120 Hz ripple rate across all ROIs in the medial temporal lobe (MTL) and anterior temporal lobe (ATL). Lines represent least-squares regression. (H) Fisher z-transformed Pearson correlation between 70–200 Hz power and 80–120 Hz ripple rate across all electrodes at baseline and during memory retrieval. Each participant is represented by a data point (*** p < .001). (I) Fisher z-transformed Pearson correlation between 70–200 Hz power and 80–120 Hz ripple rate across all electrodes during correct and incorrect memory retrieval (* p < .05). (J) Average 70–200 Hz power across all electrodes during correct compared to incorrect memory retrieval in true data (Orig) and after removal of the temporal indices of detected ripples (Control); (*** p< .001; * p < .05). Code and data is provided inFigure 1—source code 1 and at https://doi.org/10.5061/dryad.5qfttdz6t.
-
Figure 1—source code 1
Matlab code of ripple events in the iEEG signal.
- https://cdn.elifesciences.org/articles/68401/elife-68401-fig1-code1-v2.zip
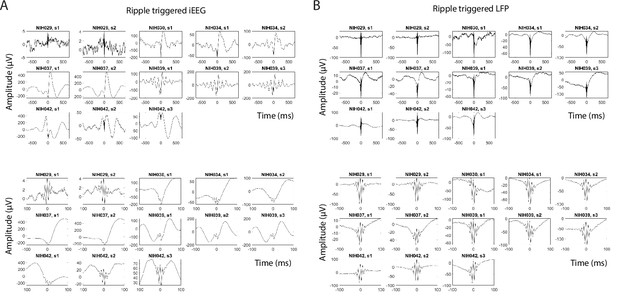
Ripple-triggered average iEEG and LFP signals.
(A) Average intracranial EEG (iEEG) signal locked to each ripple detected in the anterior temporal lobe iEEG electrodes in each session across six participants. (B) Average local field potential (LFP) signal captured using microelectrode recordings locked to each LFP ripple detected using MEAs in the anterior temporal lobe in each session across six participants.
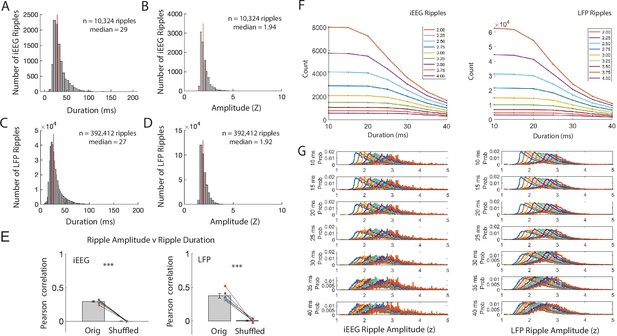
iEEG and LFP ripple characteristics with different detection thresholds.
(A) Distribution of iEEG ripple durations from all electrodes in all participants. (B) Distribution of iEEG ripple amplitudes from all electrodes in all participants. (C) Distribution of LFP ripple durations from all electrodes in all participants. (D) Distribution of LFP ripple amplitudes from all electrodes in all participants. (E) Pearson correlation between amplitude and duration of iEEG ripples (left) and LFP ripples (right) compared to Pearson correlations after random circular shifts of ripple indices by trial. The true relation between ripple band amplitude and duration is significantly greater than the shuffled distribution (true r = .372 ± .033; true-shuffled t(5) = 9.07, p = 1.4 x 10-4, paired one-tailed t-test). (F) Total count of iEEG ripple events (left) and LFP ripple events (right) detected using different amplitude and duration thresholds. (G) iEEG ripple amplitude (left) and LFP ripple amplitude (right) distributions of all events detected using different amplitude and duration thresholds.
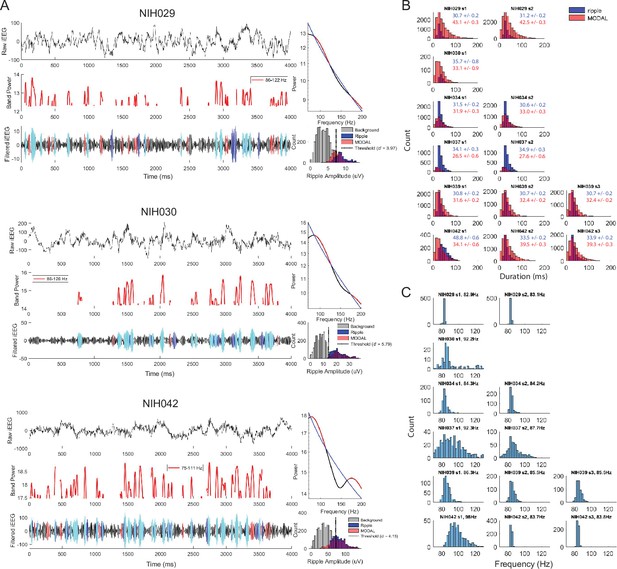
Multiple oscillations detection algorithm detected narrowband oscillations.
To complement our ripple detection method, we used the Multiple Oscillations Detection Algorithm, or MODAL, to detect narrowband oscillations (Watrous et al., 2018). This method identifies discrete periods in which narrow band oscillations exceed the background noise. (A) Example trials for three participants, each showing raw iEEG signal (top left) of one electrode in anterior temporal lobe, envelope of MODAL detected narrowband oscillations (middle left), and ripple band iEEG signal (bottom left) with periods of ripple events detected by ripple threshold (dark blue), MODAL detected events (red), and overlapping ripple and MODAL events (cyan). MODAL events are characterized by periods in which narrow band oscillations exceed 1/f noise. A power spectral density of the trial from 70 to 200 Hz (black), 1/f fit (blue), and frequencies exceeding 1/f (red) is shown on the top right. The ripple amplitude distribution composed of all samples in the trial (grey), amplitudes within detected ripples (blue), and amplitudes within MODAL detected events (red). The dotted line in the distribution is the amplitude threshold that maximizes d’ when considering the hit rate and false alarm rate of detected ripples when compared to the MODAL detected events. (B) Distribution of duration of iEEG ripples (blue) and MODAL events (red) detected across all iEEG electrodes, showing similar range in duration of events. Individual subplots are labeled with participant ID and session number; text shows mean ± SEM duration of ripples (blue) and MODAL events (red) for each participant. The mean duration of the events detected by the MODAL method was 33.5 ± 3.0 ms across participants, compared to a duration of 32.8 ± 6.3 ms for the ripples that we detected using our standard approach. (C) Distribution of center frequency of MODAL narrow band oscillations across all trials for ATL iEEG electrodes in each session in the six participants with MEAs. The mean center frequency of the identified events was 87.3 ± 3.5 Hz.
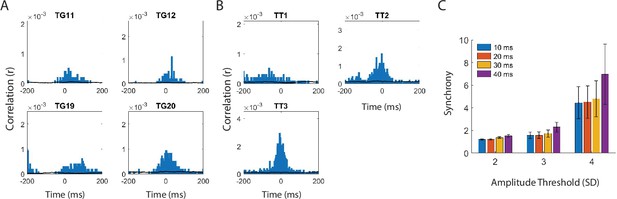
MTL-ATL cross-correlograms with different detection thresholds.
To assess whether the ripples we detect in the cortex are associated with ripples observed in the medial temporal lobe (MTL), we measured the coupling of ripples between MTL and anterior temporal lobe (ATL) by computing the cross-correlogram for events detected across these regions. (A) Mean ripple cross-correlograms for one participant for each channel in ATL (electrodes labeled as temporal grid, TG) to all channels in MTL. (B) Average ripple cross-correlograms for one participant for each channel in MTL (electrodes labeled as temporal tip, TT) to all channels in ATL. (C) Synchronization metric average across all channel pairs for each participant. We computed the average synchronization across participants using ripple detection amplitude thresholds ranging from 2 to 4 SD and duration thresholds ranging from 10 to 40 ms. Ripples are coincident across these regions above chance for all detection parameters tested, as indicated by a synchronization metric above one.
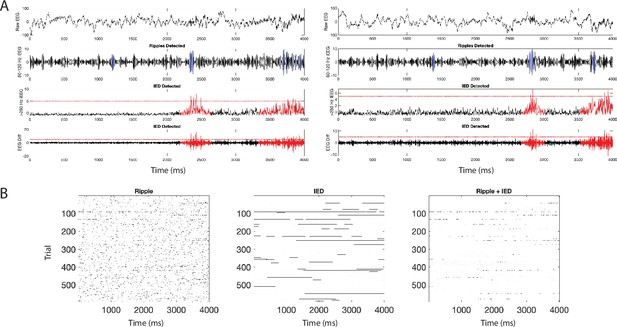
Interictal epileptiform discharge detection and overlap with ripples.
To confirm that the detected ripples are not an artifact of interictal epileptiform discharges (IEDs), we separately detected IEDs (see Materials and methods) and computed the overlap between detected ripples and detected IEDs. (A) From top to bottom, two example trials of raw iEEG, 80–120 Hz filtered iEEG with detected ripples shown in blue, 250 Hz filtered iEEG with detected IED windows in red, and first derivative of iEEG with detected IEDs in red. Horizontal red lines represent a threshold of 5 SD above the mean. The periods indicated as an IED represent a 500 ms window around time points when the threshold is crossed. (B) Example ripple raster before removal of ripples that overlap with IEDs (left), raster of IED events (middle), and overlap between ripples and IED (right). IEDs overlapped with 0.79% ± 0.11% of iEEG ripples and with 1.38% ± 0.11% of LFP ripples across participants.
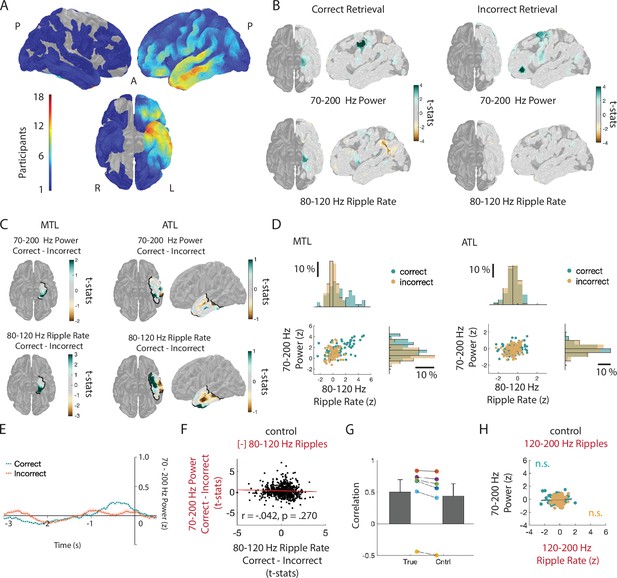
High-frequency activity reflects discrete ripples.
(A) Surface-based regions of interest (ROIs) showing electrode coverage across 21 participants. (B) ROI plots of across-participant t-statistic for 70–200 Hz power and 80–120 Hz ripple rate for correct (left) and incorrect (right) retrieval. (C) Across-participant t-statistic ROI plots from (B) for medial temporal lobe (MTL) and anterior temporal lobe (ATL) ROIs. (D) Relation between 70–200 Hz power and 80–120 Hz ripple rate for across-participant t-statistic ROIs in MTL and ATL for correct and incorrect memory retrieval. Each data point represents the average across participants for each ROI in the two brain regions. (E) 70–200 Hz power spectra after removal of temporal indices of ripples for correct and incorrect retrieval for representative iEEG electrode in MTL shown in Figure 1C. (F) Correlation between difference in 70–200 Hz power between correct and incorrect memory retrieval after removal of temporal indices of ripples with the difference in 80–120 Hz ripple rate between conditions. Each data point represents the average across participants for each ROI. (G) The true correlation between the difference in 70–200 Hz power and the difference in 80–120 Hz ripple rate is significantly greater than the correlation when ripples are removed (t(5) = 3.89, p = .0115). We also compared the two correlations as dependent groups and found a significant difference in correlation (r(true) - r(control) = .172, 95% CI = [.0691, .2764], z = 3.2677, p = .0011). We accounted for potential interaction effects using the correlation between 70–200 Hz and 70–200 Hz with ripple removed (r = -.031). (H) Correlation between 70–200 Hz Power and 120–200 Hz ripple rate separately for correct and incorrect memory retrieval. Each point represents the across-participant average in each ROI.
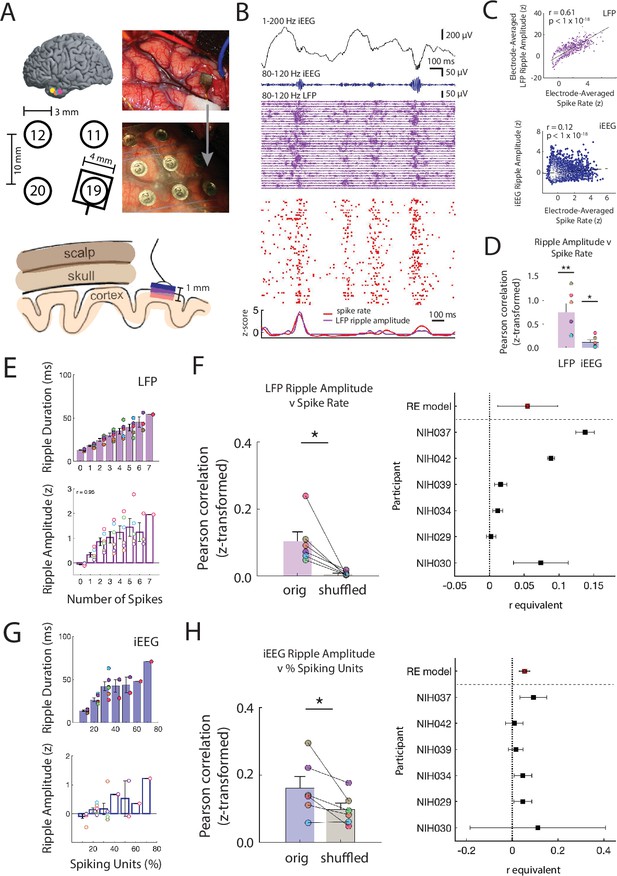
Ripple amplitudes reflect a spectrum of underlying local spiking activity.
(A) Locations of the microelectrode arrays (MEA) in six participants (top left). Location of the MEA with respect to four nearby iEEG channels in one participant (bottom left). Intraoperative photo of implanted MEA in the ATL (top right) and after placement of an iEEG grid over the MEA (bottom right). Schematic of scalp, skull and cortex with respect to one iEEG channel on the cortical surface and one MEA that extends into cortex (bottom). (B) Brief 1500 ms window of 1-200 Hz iEEG signal (black), 80-120 Hz band iEEG signal (blue), 80-120 Hz band LFP signals across all MEA electrodes (purple), and spike raster for sorted units (red). (C) Pearson correlation between average spike rate and average LFP ripple amplitude across all MEA electrodes in one participant (blue). Pearson correlation between average spike rate and iEEG ripple band amplitude for one nearby iEEG electrode in one participant (purple). Each data point represents a 100 ms non-overlapping window. (D) Fisher -transformed Pearson correlation between continuous spike rate and LFP and iEEG ripple amplitude. Group level statistics are shown as mean ± SEM across six participants. Each data point represents a participant (** p < .01, * p < .05). (E) Average duration and amplitude of ripples in the LFP signal related to the number of spikes during the ripple. Each data point represents a participant. (F) Fisher z-transformed Pearson correlation between spike rate and amplitude of coincident LFP ripple. Group level results are shown as mean ± SEM across six participants. Each data point represents a participant. True data (orig) compared to correlations when shuffling the spike time indices (shuffled; * p < .05). Forest plot of the r equivalent effect size and 95% CI for each participant and random-effect (RE) mean estimate across all participants (right). (G) Average duration and amplitude of ripples in the iEEG signal related to the number of spikes during the ripple. Each data point represents a participant. (H) Fisher -transformed Pearson correlation between percentage of spiking units and amplitude of coincident iEEG ripple. Group level results are reported as mean ± SEM across participants. True data (orig) compared to correlations when shuffling the spike time indices (shuffled; * p < .05). Forest plot of the r equivalent effect size and 95% CI for each participant and random-effect (RE) mean estimate across all participants (right). Code and data is provided inFigure 2—source code 1 and at https://doi.org/10.5061/dryad.5qfttdz6t.
-
Figure 2—source code 1
Matlab code of correlations between continuous spiking, LFP and iEEG.
- https://cdn.elifesciences.org/articles/68401/elife-68401-fig2-code1-v2.zip
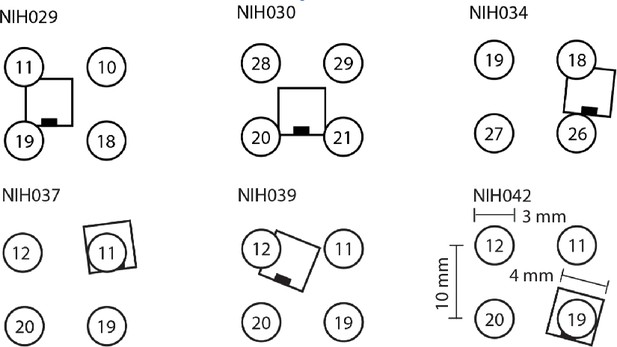
MEA position with respect to iEEG channels.
Position of MEA with respect to nearby iEEG channels in each participant.
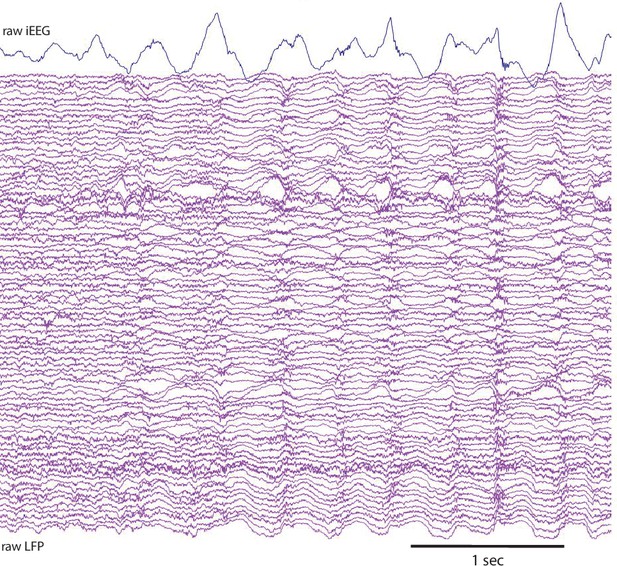
Raw iEEG and LFP Trace.
Example raw iEEG in an anterior temporal lobe electrode and simultaneous LFP traces for one representative trial.
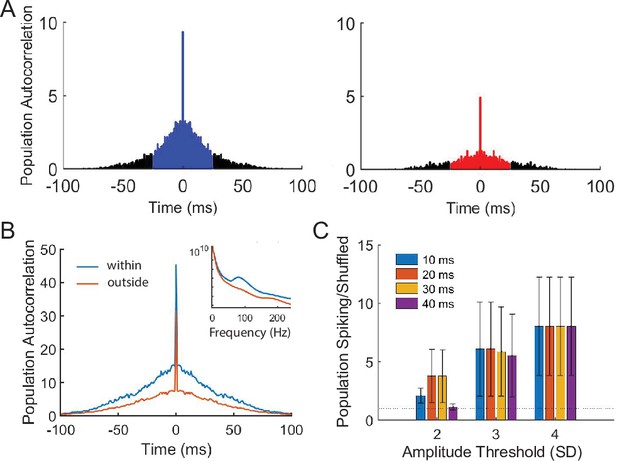
Spiking auto-correlograms within and outside ripples.
To confirm that ripples correspond to underlying bursts of spiking activity, we computed the population spiking auto-correlogram within and outside of each detected ripple event. (A) Population spiking auto-correlogram within and outside detected iEEG ripples in one representative participant. Shaded ± 25 ms values of the correlogram was used to compute the extent to which spiking activity bursts within ripples compared to outside of ripples (see Materials and methods). (B) Mean spiking auto-correlogram across participants within and outside ripples. Inset plot shows power spectral density for windows within and outside ripple events that were used to compute population spiking auto-correlogram in one representative participant. (C) Ratio of spike auto-correlograms within compared to outside of ripples when using different ripple duration and amplitude detection thresholds. Data represent mean ± SEM across participants for different detection thresholds.
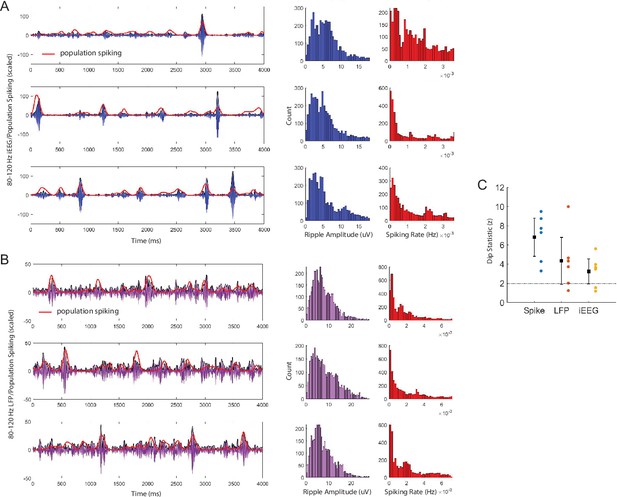
Ripple power and spike rate distributions.
We examiend whether the distribution of ripple band power in the iEEG and LFP signals, and the distribution of spiking activity, exhibit evidence of bimodality (see Materials and methods). (A) Representative trials of 80–120 Hz band iEEG signal (blue) in anterior temporal lobe electrodes and concurrent population spiking (red), showing macro-scale ripple amplitude increases are coincident with bursts of spiking. Distribution of ripple amplitude (blue) and population spiking (red) for each representative trial. (B) Representative trials of ripple LFP signal (purple) for an MEA channel and concurrent spiking (red) of units recorded in the channel, showing micro-scale ripple amplitude increases are coincident with bursts of spiking. Distribution of ripple amplitude (purple) and local spiking (red) for each representative trial. (C) Dip statistics (z-score) characterizing bimodality of population spiking, LFP ripple amplitude, and iEEG ripple amplitude averaged over channels for each participant, represented by different colors. The dip test for the bimodality of population spiking is significant in and across all participants (6.802 ± 1.013 z). The test for the bimodality of iEEG and LFP ripple amplitude is significant across participants, and significant within individual participants in at least four of the six participants with MEAs (LFP: 4.323 ± 1.257 z; iEEG: 3.236 ± .669 z).
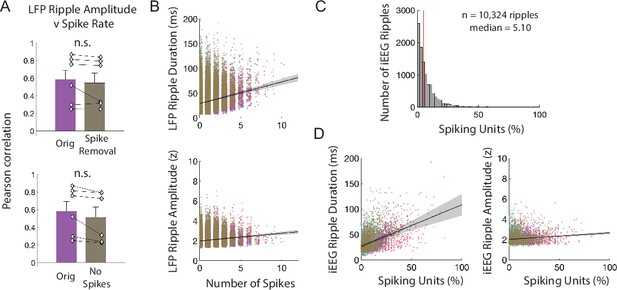
Ripples reflect underlying neuronal spiking.
(A) Pearson correlation between spike rate and continuous measures of the average LFP ripple amplitude over all micro-electrodes. Group level statistics are shown as mean ± SEM across six participants. Given the concern that spikes in the signal may generate spectral artifacts in the ripple band, we performed two control analyses to confirm that the significant correlation between LFP ripple amplitude and spike rate was not due to bandpass filtering over spikes. In the first, we removed spikes from the LFP data and in the second we restricted our analysis only to MEA electrodes that exhibited no spiking. We compared the true correlation between spike rate and LFP ripple amplitude with the correlations observed after spike removal and interpolation (top) and channels without spiking (bottom; paired t-test, t(5) = 1.29, p = .254; orig, t(5) = 5.32, p = .003; spike removal, t(5) = 4.78, p = .005). (B) Relation between number of spikes and LFP ripple duration (top) and amplitude (bottom) for all ripples in all participants. Each color represents a participant (n = 6). (C) Distribution of percentage of spiking units that co-occur with iEEG ripples across all ripples in all participants. (D) Relation between percentage of spiking units and iEEG ripple duration (left plot) and amplitude (right plot) for all ripples in all participants. Each data point represents a ripple and each color represents a participant (n = 6).
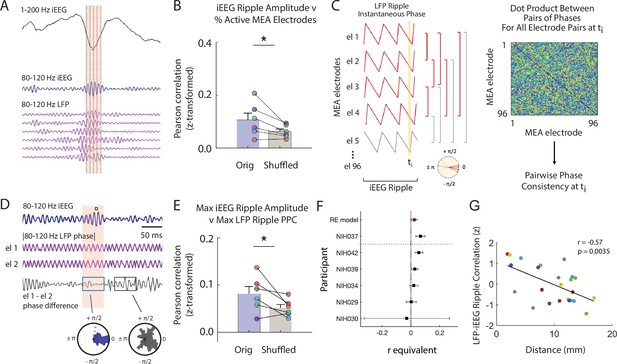
Macro-scale ripple amplitude reflects number and alignment of micro-scale ripples.
(A) Brief window around one iEEG ripple showing unfiltered iEEG signal (black), ripple band iEEG signal (blue) and ripple LFPs for one nearby iEEG channel and six microelectrode array (MEA) electrodes with coincident LFP ripples. (B) Fisher -transformed Pearson correlations for percentage of MEA electrodes containing LFP ripples and amplitude of coincident iEEG ripple. Group level results reported as mean ± SEM persists when duration of the iEEG ripple is accounted for by shuffling (* p < .05). Each data point represents a participant. (C) Schematic of calculation of pairwise phase differences across all microelectrodes to compute pairwise phase consistency. (D) Brief window around one iEEG ripple showing ripple band iEEG signal (blue), instantaneous phase of a pair of MEA electrodes (purple; out of many pairs, not shown) and instantaneous phase difference of the ch 1 and ch 2 pair (black). Maximum of iEEG ripple indicated with small black square in the shaded window above the ripple band iEEG signal. Polar histogram of all pairwise phase differences during a detected iEEG ripple is centered around 0 (blue). Polar histogram all pairwise phase differences outside of a iEEG ripple is more uniform (black). (E) Fisher -transformed Pearson correlations between maximum pairwise phase consistency across all MEA electrode pairs and maximum amplitude of iEEG ripples. Group level results, reported as mean ± SEM, persists when duration of the iEEG ripple is accounted for by shuffling (* p < .05). Each data point represents a participant. (F) Forest plot of the r equivalent effect size and 95% CI for each participant and random-effect (RE) mean estimate across all participants. (G) Relation between distance between MEA and iEEG electrode and LFP-iEEG ripple synchrony. Each data point represents the relation between a MEA and iEEG electrode in the MTL or ATL, and each color represents a different patient. Code and data is provided in Figure 3—source code 1 and at https://doi.org/10.5061/dryad.5qfttdz6t.
-
Figure 3—source code 1
Matlab code of pairwise phase consistency between LFP ripple signal and iEEG ripple amplitude.
- https://cdn.elifesciences.org/articles/68401/elife-68401-fig3-code1-v2.zip
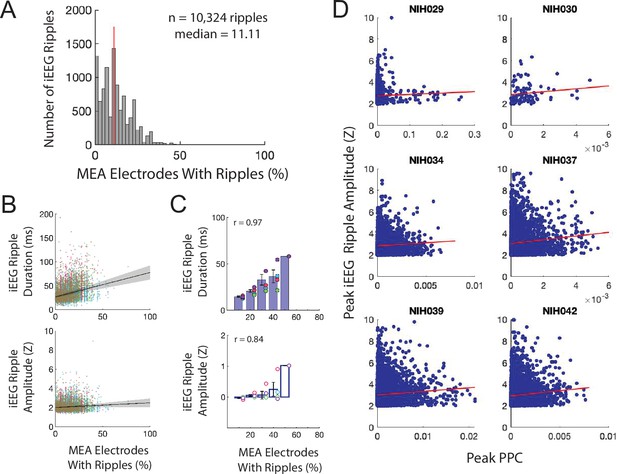
Macro-scale ripple amplitude reflects number and alignment of micro-scale ripples.
(A) Distribution of percentage of MEA electrodes containing LFP ripples that co-occur with iEEG ripples across all iEEG ripples in all participants. (B) Relation between percentage of MEA electrodes with LFP ripples and iEEG ripple duration (top) and amplitude (bottom) for all iEEG ripples in all participants. Each color represents a different participant (n = 6). (C) Relation between percentage of MEA electrodes containing LFP ripples and iEEG ripple duration (top) and amplitude (bottom). Each data point represents an average amplitude for each percentage and each color represents a participant (n = 6). (D) Relation between peak pairwise phase consistency (PPC) and peak iEEG ripple amplitude for each participant. Each data point represents a LFP ripple in each participant.
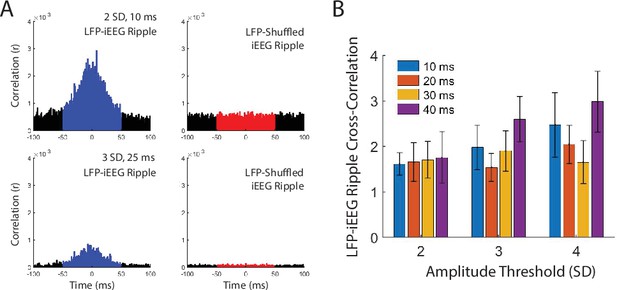
LFP-iEEG ripple cross-correlations for different detection thresholds.
(A) Example LFP-iEEG ripple cross-correlogram of true and shuffled events for one participant using a 2 SD amplitude and 10 ms duration ripple detect threshold (top) and a 3 SD amplitude and 25 ms duration threshold (bottom). (B) LFP-iEEG ripple synchrony using ripple detection thresholds with amplitudes ranging from 2 to 4 SD and durations ranging from 10 to 40 ms.
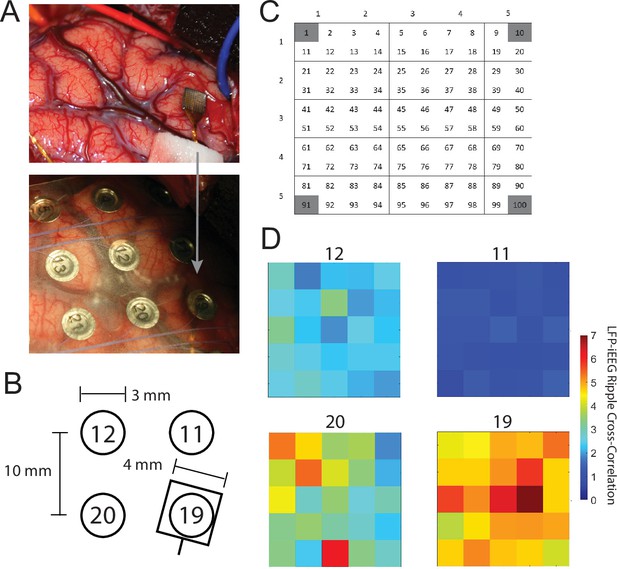
LFP-iEEG ripple cross-correlations with respect to distance.
(A) Intraoperative photo of implanted MEA in the ATL (top) and after placement of an iEEG grid over the MEA (bottom). (B) Location of the MEA with respect to four nearby iEEG channels. (C) Subgroups of micro-electrodes in one MEA for assessment of LFP-iEEG cross-correlograms across the MEA. (D) LFP-iEEG ripple synchronization, defined as the ratio of the true cross-correlogram over a chance correlogram (see Materials and methods), between each subgroup of micro-electrodes in the MEA and four nearby iEEG electrodes.
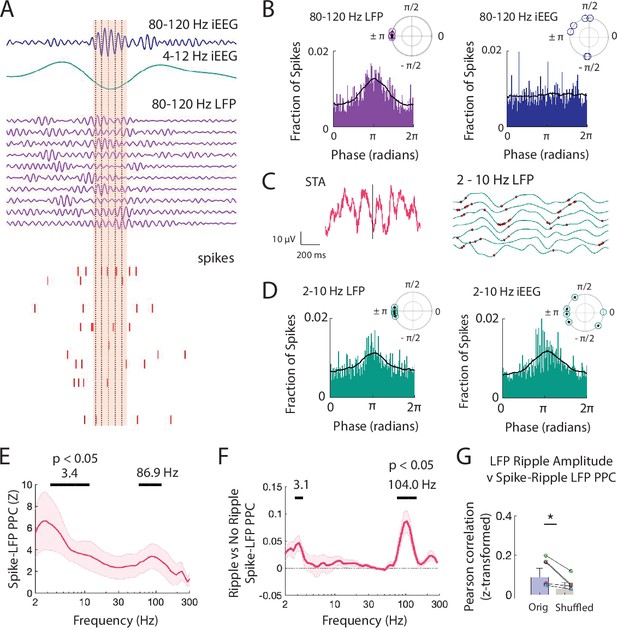
Spiking activity is phase-locked to ripples and low frequencies.
(A) Brief window around one iEEG ripple and underlying LFP ripple and spiking activity. Dashed black lines indicate trough of iEEG ripple cycles compared to concurrent LFP ripple cycles and spiking. (B) Distribution of phases of LFP ripple (left) and iEEG ripple (right) across spike times for all units. (inset) Complex mean of the distribution of phases for each participant is depicted in a polar plot. Circles filled with a star if the distribution within a participant shows significant phase-locking (Rayleigh test, p < .001). Black line shows the average of six distributions across participants. (C) Spike triggered average (STA) for spikes detected within LFP ripples, in pink, with the 2-10 Hz bandpass filtered signal, in black. Bandpass filtered STA during correct (green) and incorrect (orange) trials are also shown. (right), Brief 500 ms window of 2-10 Hz filtered LFP (green) across MEA electrodes with neuronal activity. Red dots mark spikes occurring preferentially at trough of local LFP. (D) Distribution of phases of LFP low-frequency (left) and iEEG low-frequency (right) signals across spike times for all units. (inset) Complex mean of the distribution of phases for each participant is depicted in a polar plot. Circles filled with a star if the distribution within a participant shows significant phase-locking (Rayleigh test, p < .001). Black line shows the average of six distributions across participants. (E) Mean ± SEM spike-LFP PPC across participants for all spikes to LFP for every frequency between 2 and 300 Hz. Peak frequencies of significant clusters are shown. (F) Mean ± SEM difference in spike-LFP PPC between spikes that co-occur with LFP ripples and spikes that do not across participants. Peak frequencies of significant clusters are shown. (G) Fisher -transformed Pearson correlations between spike-LFP ripple PPC and LFP ripple amplitude. Each data point represents a participant. True data (orig) compared to correlations when shuffling the spike time indices (shuffled; * p < .05). Code and data is provided inFigure 4—source code 1 and at https://doi.org/10.5061/dryad.5qfttdz6t.
-
Figure 4—source code 1
Matlab code of pairwise phase consistency between spiking and LFP.
- https://cdn.elifesciences.org/articles/68401/elife-68401-fig4-code1-v2.zip
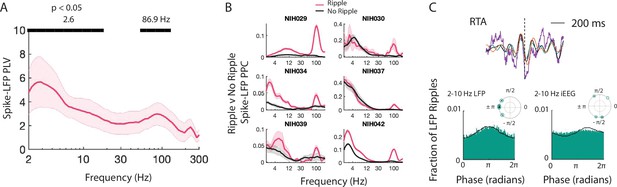
Spiking activity is phase-locked to ripples and low frequencies.
(A) Spike-LFP phase-locking value (PLV) for each frequency shown as mean ± SEM across participants. PLV confirms that spiking activity is locked to low and high frequency activity across participants (peak at 2.6 Hz and 86.9 Hz, p < .05, permutation test). (B) Spike-LFP pairwise phase consistency (PPC) for spikes that occur with LFP ripples and for spikes that do not occur with LFP ripples for each participant, shown as mean ± SEM across MEA electrodes. (C) LFP ripple-triggered average (RTA) for LFP ripples that co-occur with iEEG ripples, in purple, with the bandpass filtered signal, in black, for one representative participant. Also shown is the bandpass filtered RTA during correct memory retrieval (green) and incorrect trials (orange). Distribution of phases of LFP low frequency (lower left histogram) and iEEG low-frequency (lower right histogram) signals across LFP ripple times for all MEA electrodes. Complex mean of the distribution of phases for each participant is depicted in a polar plot with circles filled with a star if the distribution shows significant phase-locking (Rayleigh test, p < .001). Black line shows the average of six distributions across participants.
Additional files
-
Transparent reporting form
- https://cdn.elifesciences.org/articles/68401/elife-68401-transrepform1-v2.pdf
-
Source code 1
Matlab scripts to generate main figures.
- https://cdn.elifesciences.org/articles/68401/elife-68401-supp1-v2.zip