Light sets the brain’s daily clock by regional quickening and slowing of the molecular clockworks at dawn and dusk
Figures
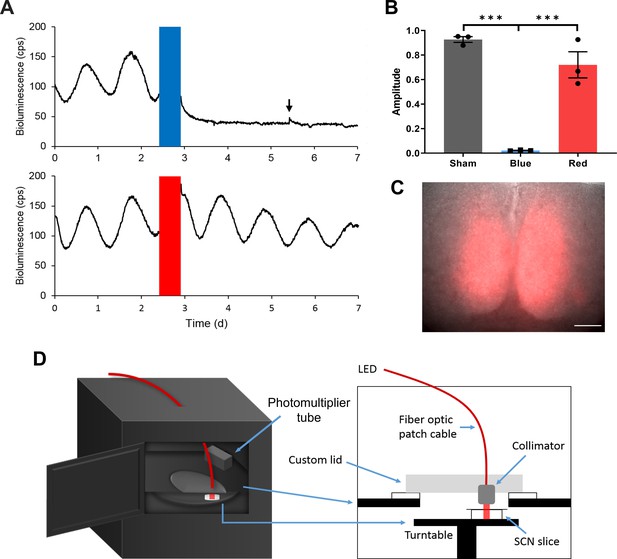
Long-term Optogenetic Stimulation System for Circadian Entrainment Ex Vivo.
(A) Representative PER2::LUC bioluminescence rhythms of adult SCN slices exposed to either red (top) or blue (bottom) 10 Hz light pulses (red or blue bars) for 12 hr. The black arrow indicates the timing of media change. (B) Fold change in the rhythm amplitude following sham, blue, or red light exposure (Student’s t-test, mean ± SEM, n = 3, ***p < 0.001). (C) Merged ChrimsonR-tdT fluorescence and the brightfield images of an SCN slice. Scale = 100 μm. (D) Diagrams showing a multi-channel luminometer integrated with an optogenetic stimulation apparatus.
-
Figure 1—source data 1
Source data for Figure 1B.
- https://cdn.elifesciences.org/articles/70137/elife-70137-fig1-data1-v1.xlsx
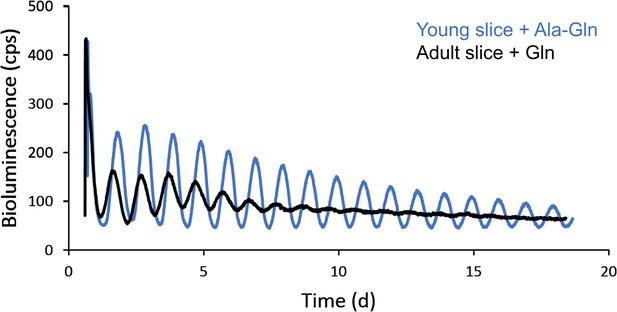
Improved PER2::LUC rhythmicity in SCN slices explanted from young mice to culture medium containing stabilized glutamine.
Representative PER2::LUC rhythms of SCN slice cultures from an adult (P60) and a young (P12) mouse. Young SCN slice cultures with stabilized glutamine (alanyl-glutamine) showed higher amplitude in PER2::LUC rhythms for a longer duration, compared to rhythms from adult SCN slices in culture medium containing regular glutamine.
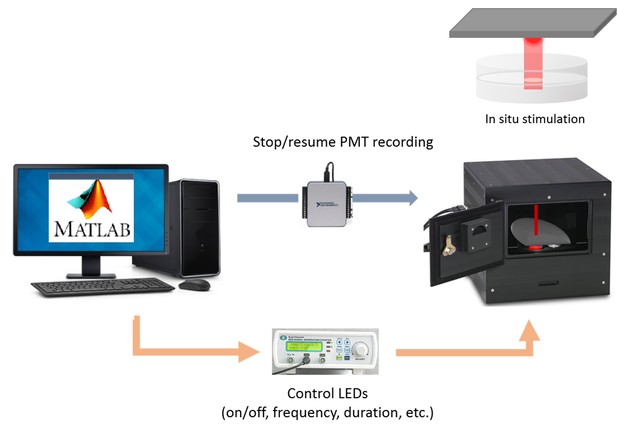
Diagram of an integrated system for long-term luminescence recording and optogenetic stimulation.
Custom-written Matlab code has access to a luminometer data collection software, a multifunction I/O device turning on/off the photomultiplier tubes (PMTs), and a signal generator controlling LEDs. Thus, it can schedule periodic stimulation and execute a series of events during optogenetic stimulation — pause PMT recording, target positioning, LED stimulation, and PMT recording resumption.
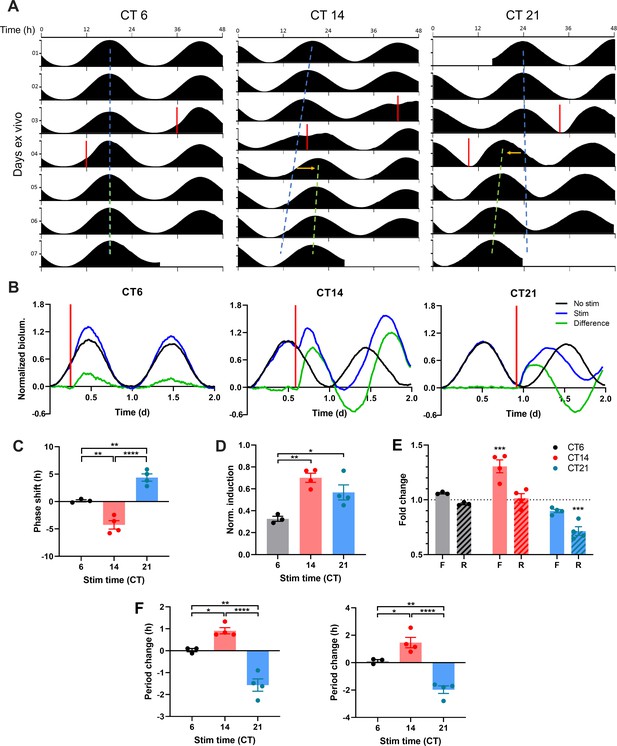
ChrimsonR-driven Optogenetic Stimulation Alters the Waveform of SCN PER2 Rhythms to Reset the SCN Clock.
(A) Representative double-plotted PER2::LUC bioluminescence actograms of SCN slices stimulated with single 15 min 10 Hz optogenetic pulses (red bar) at CT6 (left), 14 (middle), and 21 (right). Linear regressions of the pre-stimulation and post-stimulation cycle peaks are indicated as the blue and green dashed lines, respectively. Phase shifts are depicted by yellow arrows. (B) Representative PER2::LUC rhythms of SCN slices before and after stimulation (red bar). Green traces depict differences in normalized bioluminescence between the pre-stim and post-stim rhythms (black and blue traces, respectively). (C) Quantification of phase shifts following stimulation. Positive and negative phase shifts indicate phase advance and delay, respectively. (One-way ANOVA with Tukey’s multiple comparisons test, mean ± SEM, n = 3–4, **p < 0.01, ****p < 0.0001). (D) Normalized PER2::LUC induction following stimulation. (One-way ANOVA with Tukey’s multiple comparisons test, mean ± SEM, n = 3–4, *p < 0.05, **p < 0.01). (E) Fold change in duration of the rising (R; dashed boxes) and falling (F; open boxes) phases of PER2::LUC rhythms following stimulation. (RM two-way ANOVA with Sidak’s multiple comparisons tests, mean ± SEM, n = 3–4, ***p < 0.001). (F) Quantification of period changes following stimulation using linear regression of peaks (left) and Lomb-Scargle periodogram (right). Positive and negative period changes indicate period lengthening and shortening, respectively. (One-way ANOVA with Tukey’s multiple comparisons test, mean ± SEM, n = 3–4, *p < 0.05, **p < 0.01, ****p < 0.0001).
-
Figure 2—source data 1
Source data for Figure 2C–F.
- https://cdn.elifesciences.org/articles/70137/elife-70137-fig2-data1-v1.xlsx
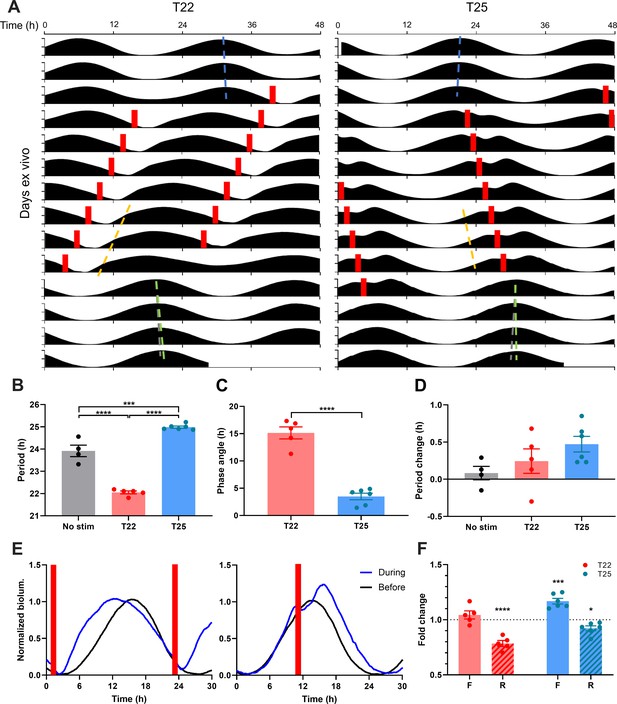
Optogenetic Cycles Entrain PER2::LUC Rhythms in the SCN by Triggering Daily Waveform Changes that Match the SCN Clock to the Cycle Period.
(A) Representative double-plotted PER2::LUC bioluminescence actograms of SCN slices entrained with 1–1.5 hr 10 Hz optogenetic pulse (red bars) every 22 hr (left) or 25 hr (right). Linear regressions of the pre- and post-entrainment cycle peaks are indicated as the blue and green dashed lines, respectively. Yellow dashed lines indicate half-maxes on the rising phase during entrainment. Grey dashed lines indicate the pre-entrainment cycle period as a reference. (B–D) Quantification of period during entrainment (B), phase angle of entrainment (C), period change by entrainment (D). (B and D) were analyzed using one-way ANOVA with Tukey’s multiple comparisons tests (mean ± SEM, n = 4–6, ****p < 0.0001, ***p < 0.001), (C) was analyzed using Student’s t-test (mean ± SEM, n = 5–6, ****p < 0.0001). (E) Representative waveforms of PER2::LUC bioluminescence rhythms before (black trace) and during (blue trace) entrainment to T22 (left) and T25 cycles (right). Red bars depict optogenetic stimulation during entrainment. (F) Fold change in duration of the rising (R; dashed boxes) and falling (F; open boxes) phases of PER2::LUC rhythms during entrainment compared with before entrainment. (RM two-way ANOVA with Sidak’s multiple comparisons tests, mean ± SEM, n = 5–6, ***p < 0.001, ****p < 0.0001).
-
Figure 3—source data 1
Source data for Figure 3B–D and F.
- https://cdn.elifesciences.org/articles/70137/elife-70137-fig3-data1-v1.xlsx
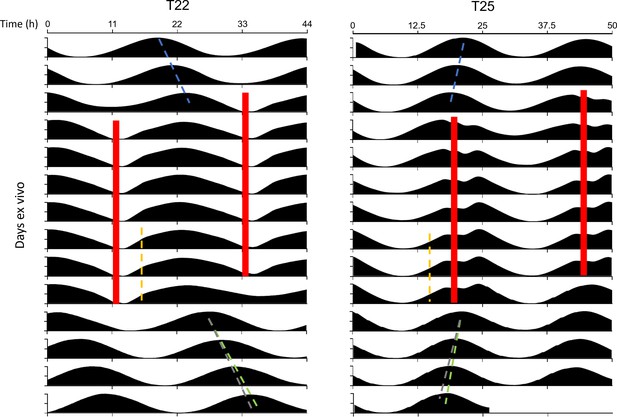
PER2::LUC rhythms in SCN slices entrain to optogenetic T-cycles.
Representative double-plotted PER2::LUC bioluminescence actograms of SCN slices entrained with 1–1.5 hr 10 Hz optogenetic pulse (red bars) every 22 hr (left) or 25 hr (right). Actograms are plotted on a 22 hr (left) or 25 hr (right) time scale. Linear regressions of the pre- and post-entrainment cycle peaks are indicated as the blue and green dashed lines, respectively. Yellow dashed lines indicate half-maxes on the rising phase during entrainment. Gray dashed lines indicate the pre-entrainment cycle period as a reference.
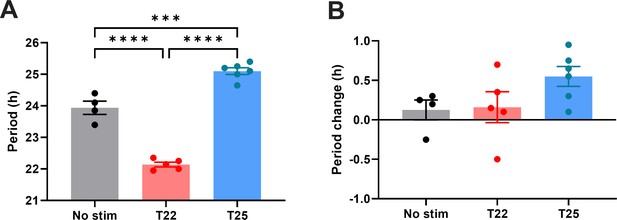
Quantification of period during entrainment (left) and period changes following entrainment (right) using Lomb-Scargle periodogram.
(One-way ANOVA with Tukey’s multiple comparisons tests, mean ± SEM, n = 4–6, ****p < 0.0001, ***p < 0.001).
-
Figure 3—figure supplement 2—source data 1
Source data for Figure 3—figure supplement 2.
- https://cdn.elifesciences.org/articles/70137/elife-70137-fig3-figsupp2-data1-v1.xlsx
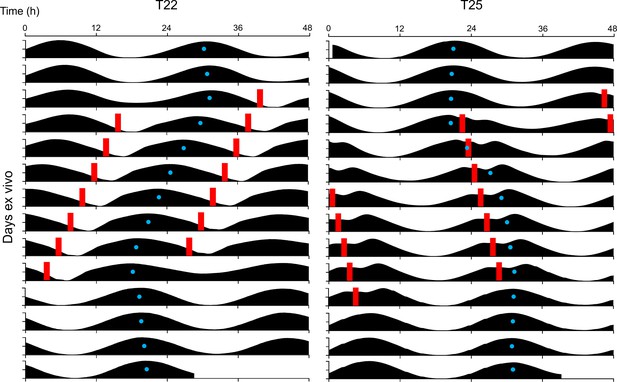
Acrophase fitting of PER2::LUC bioluminescence actograms from Figure 3A.
Blue dots denote acrophases.
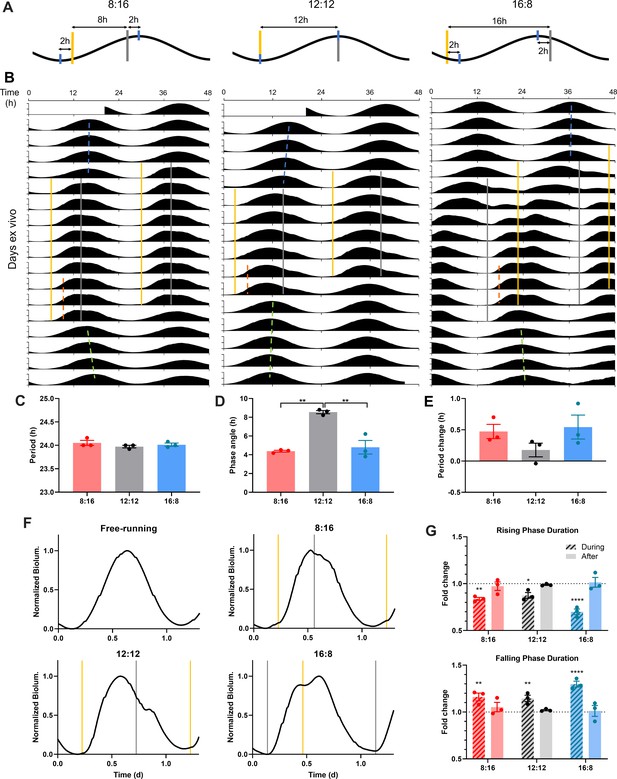
SCN entrain to optogenetic simulation of dawn and dusk via differential PER2::LUC rhythm waveform changes.
(A) Schematic diagram of optogenetic stimulation paradigm for 8:16 (left), 12:12 (middle), and 16:8 (right) skeleton photoperiod entrainment. Optogenetic pulses (yellow and grey bars for nominal dawn and dusk pulses, respectively) were given twice (8 hr, 12 hr, or 16 hr apart) every 24 hr, targeting near the peak and trough (blue ticks) of PER2::LUC rhythms (black). (B) Representative double-plotted PER2::LUC bioluminescence actograms of SCN slices entrained with 15 min 10 Hz red optogenetic pulses at 8:16 (left), 12:12 (middle), or 16:8 (right) interval of 24 hr. Linear regressions of the pre-, post-entrainment cycle peaks and during-entrainment cycle half-maxes are indicated as the blue, green, and orange dashed lines, respectively. (C–E) Quantification of period during entrainment (C), phase angle of entrainment (D), period change following entrainment (E). (One-way ANOVA with Tukey’s multiple comparisons test, mean ± SEM, n = 3, **p < 0.01). (F) Representative waveforms of PER2::LUC rhythms in SCN slices in a free-running condition (before and after entrainment), and those entrained to optogenetic 8:16, 12:12, 16:8 photoperiod entrainment. Optogenetic light pulses were given at times indicated by yellow and grey lines marking nominal dawns and dusks, respectively. (G) Fold changes in the duration of the rising phase (top) and the falling phase (bottom) during and after entrainment, compared to before entrainment. (RM two-way ANOVA with Sidak’s multiple comparisons tests, mean ± SEM, n = 3, *p < 0.05, **p < 0.01, ****p < 0.0001).
-
Figure 4—source data 1
Source data for Figure 4C–E and G.
- https://cdn.elifesciences.org/articles/70137/elife-70137-fig4-data1-v1.xlsx
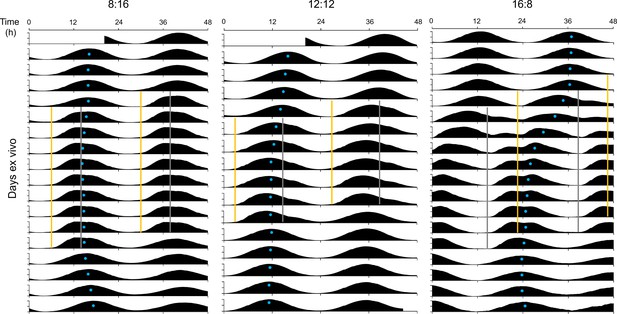
Acrophase fitting of PER2::LUC bioluminescence actograms from Figure 4B.
Blue dots denote acrophases.
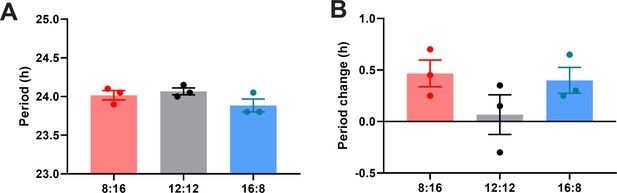
Quantification of (A) period during entrainment and (B) period changes following entrainment using Lomb-Scargle periodogram.
-
Figure 4—figure supplement 2—source data 1
Source data for Figure 4—figure supplement 2.
- https://cdn.elifesciences.org/articles/70137/elife-70137-fig4-figsupp2-data1-v1.xlsx
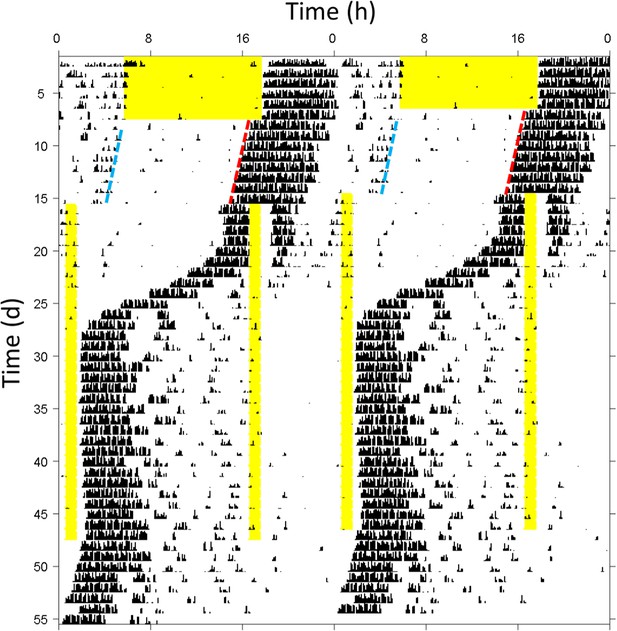
Phase jump in mouse circadian behavior during long skeleton photoperiod entrainment.
Representative double-plotted wheel-running actogram showing a behavioral phase jump to the preferred phase angle of 16:8 long skeleton photoperiod entrainment. The black tick marks indicate 6 min-binned wheel-running activity. A mouse under a light cycle of 12 hr light (yellow bar) and 12 hr darkness was released into constant darkness and then presented with a 16:8 skeleton photoperiod. The 16 hr interval between 1 hr light pulses defining dawn and dusk was initially aligned with the subjective day (i.e. the time from the nocturnal behavioral offset [blue dashed line] to the onset [red dashed line]). As the circadian rhythm rapidly phase-advanced across the nominal dusk pulse, the shorter 8 hr interval became aligned with the subjective day instead of the night.
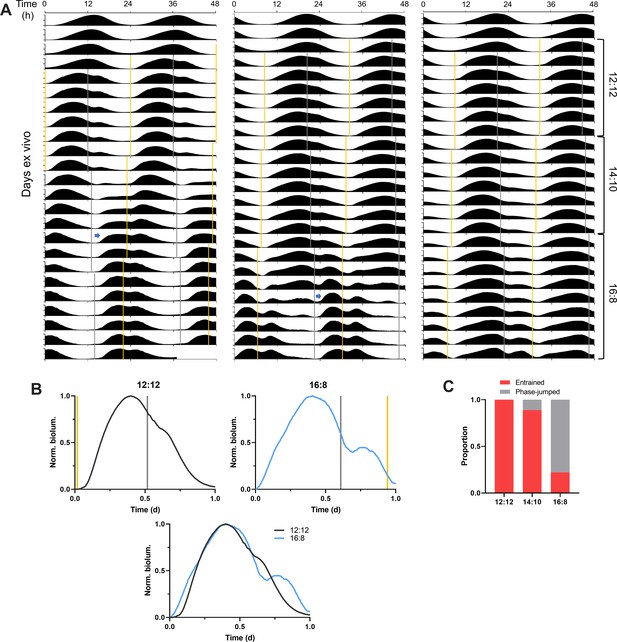
Gradual shifts from equinox to long optogenetic skeleton photoperiods reveal SCN PER2::LUC rhythms stably entrained to long skeleton photoperiods.
(A) Representative double-plotted PER2::LUC bioluminescence actograms of SCN slices entrained to optogenetic skeleton photoperiods (yellow and gray bars for nominal dawn and dusk pulses, respectively) from the 12:12 skeleton to the 14:10 to the 16:8. The left and the middle actogram show that a phase jump (blue arrow) begins during the 14:10 and the 16:8 skeleton photoperiods, respectively. The right actogram depicts SCN PER2::LUC rhythms entrained to all the three skeleton photoperiods without a phase jump. (B) Representative waveforms of the PER2::LUC rhythms entrained to the 12:12 (upper left) and the 16:8 (upper right) skeleton photoperiods simulated by optogenetic stimulation (yellow and grey bars for nominal dawn and dusk pulses, respectively). The bottom plot shows a superimposed image of the two upper plots. (C) Proportion of entrained SCN slices (without a phase jump) and the phase-jumped slices for the 12:12, 14:10, and 16:8 skeleton photoperiods (n = 9).
-
Figure 5—source data 1
Source data for Figure 5C.
- https://cdn.elifesciences.org/articles/70137/elife-70137-fig5-data1-v1.xlsx
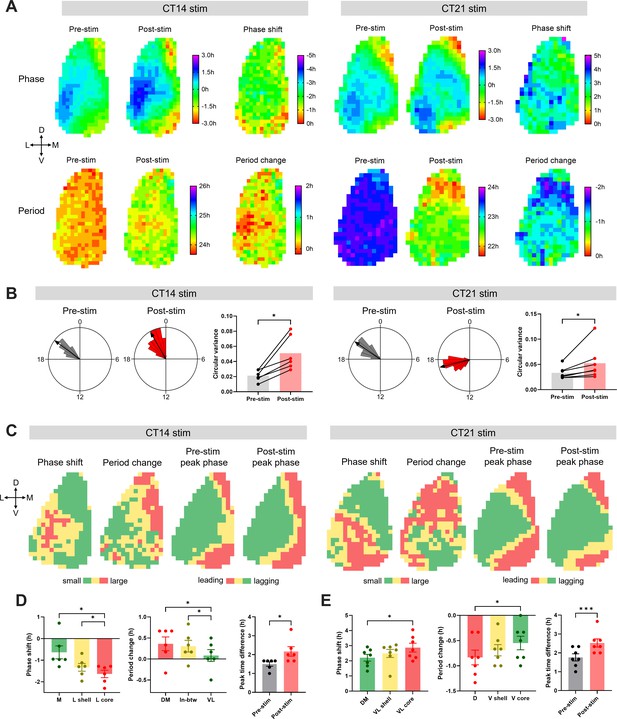
Regional distribution of circadian phase and period in the SCN, and heterogeneity of the phase and the period responses in the SCN to optogenetic light pulses.
(A) The upper row shows phase maps of PER2::LUC bioluminescence rhythms in the unilateral SCN before and after optogenetic stimulation at CT14 (left) or CT21 (right), and regional distribution of the phase shifts in response to corresponding stimulation. The lower row displays the period maps before and after the stimulation, and the period change maps. The crossed arrows denote the SCN orientation. D = dorsal, V = ventral, M = medial, L = lateral. The phase maps depict peak times in hours relative to the mean in each condition. Pre-stim and post-stim phases indicate the last peak before stimulation and the first peak after stimulation, respectively. Positive and negative phase shifts indicate phase advances and delays, respectively. Positive and negative period changes indicate period lengthening and shortening, respectively. (B) Representative Rayleigh plots (24 hr circular plots) for regional peak PER2::LUC phases in the SCN, and bar graphs of the circular variance before and after optogenetic stimulation at CT14 (left) or CT21 (right). (Wilcoxon matched-pairs test, n = 6–7, *p < 0.05). The arrows in the Rayleigh plots indicate mean Rayleigh vectors. (C) Group-averaged clusters of the phase shift, period change, pre-stimulation peak phase, and post-stimulation peak phase for CT14 (left) or CT21 (right) stimulation (n = 6–7). Clusters were hierarchically formed using Ward’s minimum variance method. Different colors indicate different clusters. The crossed arrows denote the SCN orientation. D = dorsal, V = ventral, M = medial, L = lateral. (D–E) Phase shifts (left) and period changes (middle) in corresponding clusters in (C), and peak time differences between the phase-leading and the phase-lagging clusters (right) for CT14 (D) or CT21 stimulation (E). Phase shifts and period changes were analyzed using RM One-way ANOVA with Tukey’s multiple comparisons test, mean ± SEM, n = 6–7, *p < 0.05. Peak time differences were analyzed using paired t-test, mean ± SEM, n = 6–7, *p < 0.05, ***p < 0.001.
-
Figure 6—source data 1
Source data for Figure 6B and D–E.
- https://cdn.elifesciences.org/articles/70137/elife-70137-fig6-data1-v1.xlsx
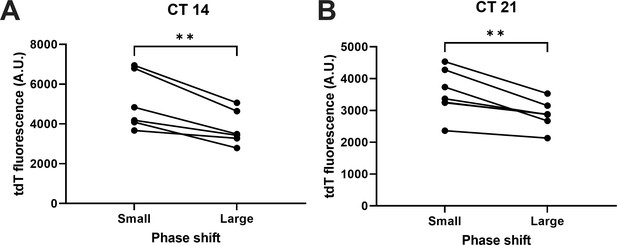
ChrimsonR-tdT fluorescence levels in different phase shift clusters in the Figure 6C for (A) CT14 and (B) CT21 stimulation.
(Paired t-test, n = 6–7, **p < 0.01).
-
Figure 6—figure supplement 1—source data 1
Source data for Figure 6—figure supplement 1.
- https://cdn.elifesciences.org/articles/70137/elife-70137-fig6-figsupp1-data1-v1.xlsx
Tables
Reagent type (species) or resource | Designation | Source or reference | Identifiers | Additional information |
---|---|---|---|---|
Strain, strain background (M. musculus) | PER2::LUC | Yoo et al., 2004 | RRID: IMSR_JAX:006852 | |
Transfected construct (M. musculus) | AAV1-Syn-ChrimsonR-tdT | Klapoetke et al., 2014 | Addgene viral prep # 59171-AAV1 | |
Software, algorithm | MATLAB | Mathworks | RRID:SCR_001622 | |
Software, algorithm | ClockLab Analysis | Actimetrics | Matlab-based ClockLab Analysis ver. 2.72 | |
Software, algorithm | LumiCycle | Actimetrics | ||
Software, algorithm | Signal Generator | Mhinstek | ||
Software, algorithm | Prism | GraphPad | RRID:SCR_002798 | |
Software, algorithm | SPSS | IBM | RRID:SCR_002865 | |
Software, algorithm | OptoLumicycle | This study | https://github.com/SuilKim/OptoLumicycle (Kim, 2021b copy archived at swh:1:rev:fffae11b1135c5775ee40ffa48bed05315f5282e) | Codes for an integrated system of luminometry and optogenetic stimulation |
Other | LED driver | Thorlabs | LEDD1B | |
Other | Fiber-coupled LEDs | Thorlabs | M470F3 (470 nm), M625F2 (625 nm) | |
Other | Fiber collimation package | Thorlabs | F230SMA-B | |
Other | Multi-mode fiber cable (Ø1500μm, 0.39NA) | Thorlabs | M93L | |
Other | Relay switch | Sensata-Crydom | DC60S5-B | |
Other | Multi-functional I/O device | National Instruments | USB-6001 |