Columnar processing of border ownership in primate visual cortex
Figures
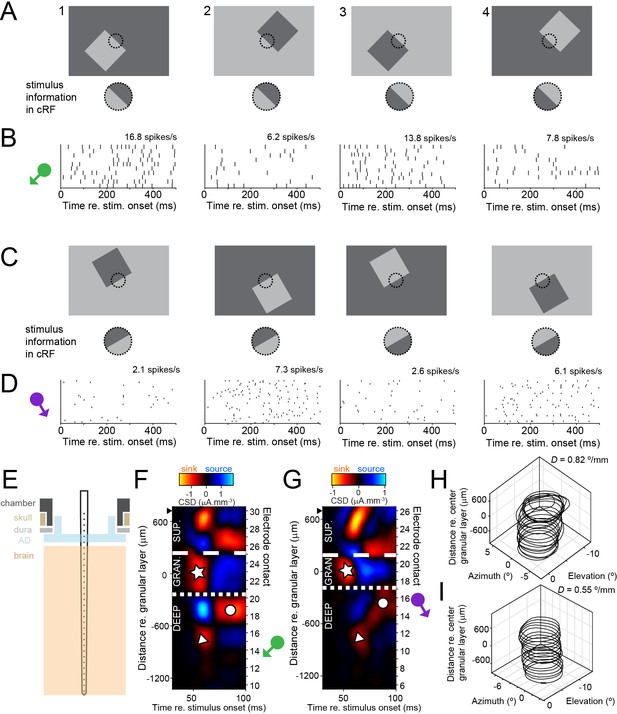
Laminar multielectrode recordings from border ownership-selective units in area V4.
(A) Top row shows set of border ownership stimuli. Black dotted outline represents the classical receptive field (cRF). Bottom row shows that the stimulus information in the cRF is identical for stimuli 1 and 2, and for stimuli 3 and 4. (B) Dot rasters showing responses to the stimuli in A from a border ownership-selective well-isolated unit. The symbol on the left indicates the preferred side of border ownership for the unit. Average spike rates in the stimulus window are indicated above the panels. (C, D) Similar to A and B, for a multiunit cluster recorded during a different penetration. (E) Cartoon showing the recording setup. A laminar multielectrode probe with 32 channels was lowered through a transparent artificial dura (AD), orthogonal relative to the cortical surface. (F) Laminar compartments (superficial; granular [input]; deep layers) were estimated using current source density (CSD) analysis. See text for definitions of the compartments and explanation of symbols. Distance from center of granular layer is shown along ordinate on the left, and the number of electrode contact on the right (higher numbers correspond to more superficial contacts). Black solid triangle indicates position of most superficial electrode contact on which multiunit activity was recorded. Data are from the same penetration during which the unit in B was recorded. The position of the green symbol indicates that this unit was positioned in the deep layers. See also Figure 1—figure supplements 1–3. (G). Similar to F, for the penetration during which the unit shown in D was recorded. (H) Receptive field contours for multiunit activity recorded on different electrode contacts from the penetration shown in F. Contours are drawn at z = 3. Orthogonality of the penetration was evaluated by fitting a line through the centers of the receptive field contours and computing D, the distance between pairs of azimuth and elevation coordinates of receptive field centers per mm depth (Methods). (I) Similar to H, for the penetration shown in G.
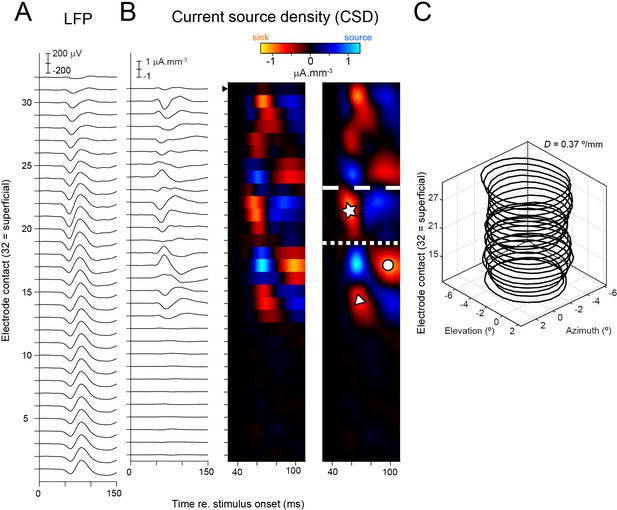
Construction of current source density (CSD) map.
Data shown from an example penetration. (A) Average local field potential (LFP) from each electrode contact in response to small rings positioned in the classical receptive field (cRF). (B) CSD is computed as the second spatial derivative of the LFP. Left panel: CSD traces. Negative values correspond to current sinks, positive values to current sources. Middle panel: CSD traces plotted on a color scale. Right panel: smoothed CSD map (Methods). Symbols and lines between compartments drawn as in Figure 1F and G. Black solid triangle indicates position of most superficial electrode contact on which multiunit activity was recorded. (C) cRF contours for different electrodes from this penetration (contours drawn at z = 3). (D) is an estimate of the orthogonality as the distance between the receptive field centers per mm depth (as in Figure 1H and I; Methods).
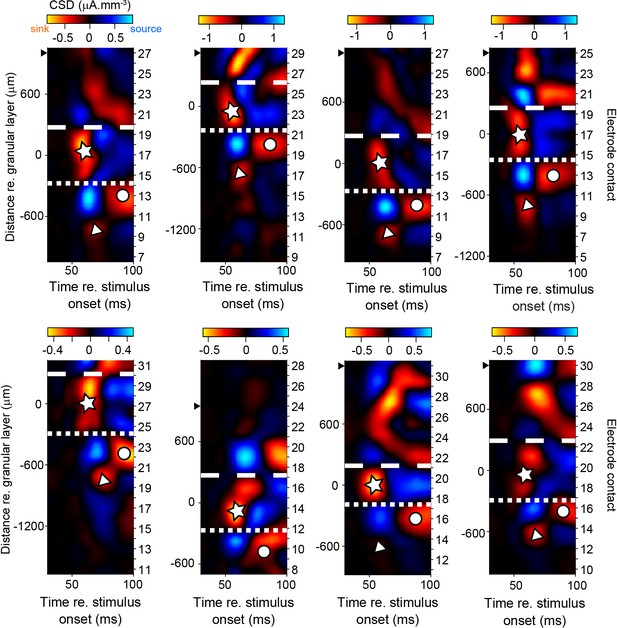
Additional examples of current source density (CSD) maps.
Each panel shows a CSD map from a different penetration. Distance from center of granular layer is shown along ordinate on the left, and the number of electrode contact on the right (higher numbers correspond to more superficial contacts). Reddish colors are current sinks, blueish colors are current sources. Symbols as in Figure 1F and G. Black solid triangles indicate position of most superficial electrode contact on which multiunit activity was recorded (in cases where this could be determined, that is for which the most superficial contact did not record multiunit activity). Sink–source patterns are strikingly similar to those reported in the behaving macaque in V4 by other laboratories (Pettine et al., 2019, their Figure 1C Lu et al., 2018, their Figure S4B), and in another downstream cortical area (area 36: Takeuchi et al., 2011, their Figure S1). Top row panels are from penetrations from animal D, bottom panels are from penetrations from animal Z.
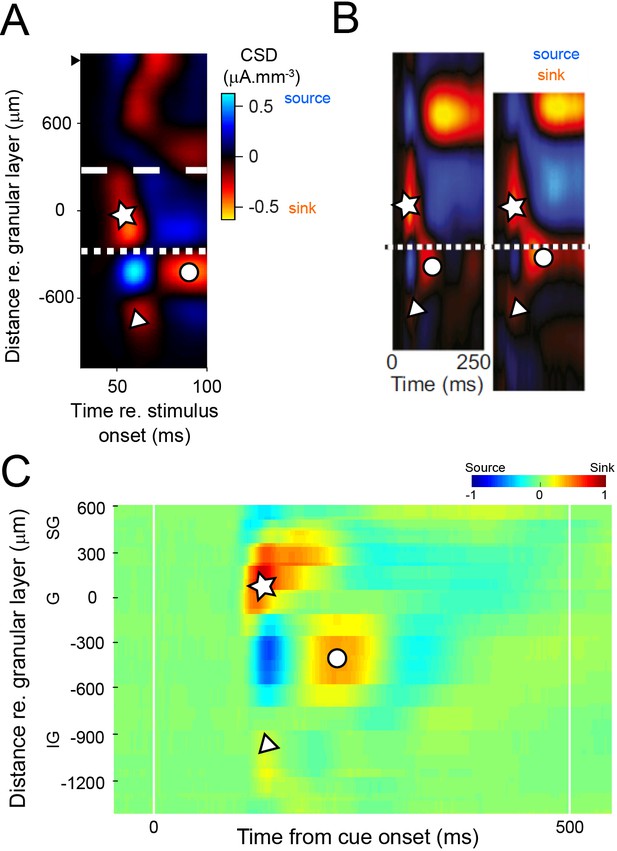
Consistency of current source density (CSD) maps between studies and cortical areas.
(A) Population average of CSD maps. Individual CSD maps were aligned relative to the border between granular layer and deep layers. The average of these aligned maps was computed for the 81 penetrations that were used in the laminar analyses (interpretable CSD maps). Black solid triangle indicates the average position of most superficial electrode contact on which multiunit activity was recorded for these penetrations. (B) Two examples of CSD maps from another study in V4 in the awake macaque (Pettine et al., 2019, their Figure 1C). Reddish colors are current sinks, blueish colors are current sources. Current sink/source pattern (symbols) is consistent with the population average in our study (panel A) as well as with individual penetrations (Figure 1; Figure 1—figure supplements 1 and 2). Dotted line drawn by Pettine et al., 2019 below the prominent current sink with short latency (star). Modified from Laminar segregation of sensory coding and behavioral readout in macaque V4. Pettine WW, Steinmetz NA, Moore T. Proc Natl Acad Sci U S A. 2019 Jul 16;116(29):14749–14754. doi:10.1073/pnas.1819398116. Reprinted with permission from PNAS. Further reproduction of this panel would need permission from the copyright holder. (C) Population average of CSD from a study in medial temporal cortex in the awake macaque (Takeuchi et al., 2011, their Figure S1A). Current sink–source pattern (symbols) is consistent with the population average in our study (panel A). Takeuchi et al., 2011 applied electrolytic lesion marks on electrode contacts in six penetrations, and were able to verify histologically that the earliest current sink (indicated by white star) corresponds to the granular layer. Reprinted from Reversal of interlaminar signal between sensory and memory processing in monkey temporal cortex. Takeuchi D, Hirabayashi T, Tamura K, Miyashita Y. Science. 2011 Mar 18;331(6023):1443–1447. doi:10.1126/science.1199967. Reprinted with permission from AAAS. This panel is not covered by the CC-BY 4.0 licence and further reproduction of it would need permission from the copyright holder.
© 2019, National Academy of Sciences permissions. Modified from Laminar segregation of sensory coding and behavioral readout in macaque V4. Pettine WW, Steinmetz NA, Moore T. Proc Natl Acad Sci U S A. This panel is not covered by the CC-BY 4.0 licence and further reproduction of it would need permission from the copyright holder.
© 2011, American Association for the Advancement of Science permissions. Reprinted with permission from AAAS: Takeuchi D, Hirabayashi T, Tamura K, Miyashita Y. Science. 2011 Mar 18;331(6023):1443-7. This panel is not covered by the CC-BY 4.0 licence and further reproduction of it would need permission from the copyright holder.
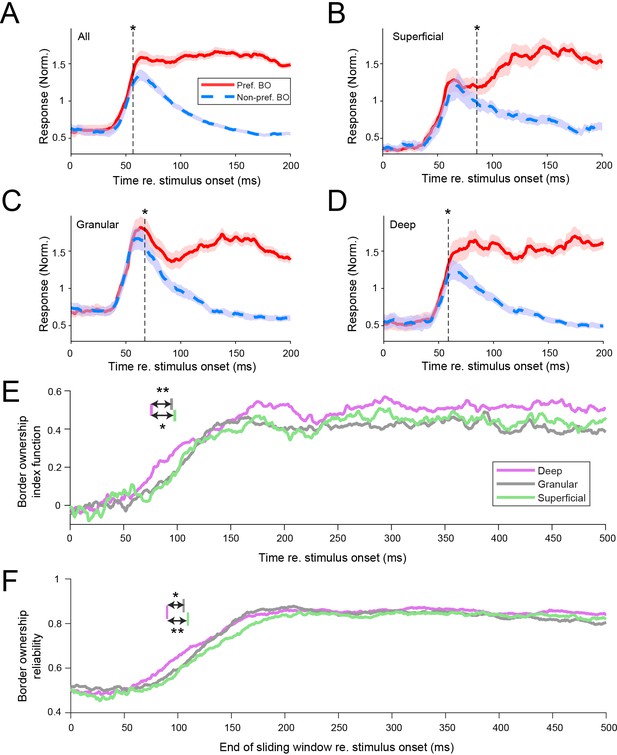
Border ownership selectivity occurs first in deep cortical layers.
(A) Response time courses for the population of border ownership-selective well-isolated units (n = 350). Functions are plotted separately for the responses to the preferred side of border ownership (solid red line; mean ± standard error of the mean [SEM]) and the non-preferred side of border ownership (dashed blue line). The vertical dashed line indicates when the difference between the functions first becomes significant (Wilcoxon signed rank test, p < 0.05 for ≥ 20 ms). (B–D) Similar to A, for the subpopulations of well-isolated units selective for border ownership that could be located, respectively, to superficial (B), granular (C), and deep (D) layers (see Methods for criteria of layer assignment). Superficial: n = 58 units; granular: n = 102 units; deep: n = 121 units. Analysis as in panel A. See also Figure 2—figure supplement 1. (E) Border ownership index functions of the different laminar compartments (see Methods). Colored vertical lines indicate latency for the different layers, defined as the earliest crossing of the border ownership index function for ≥20 ms with the threshold. The threshold was set at the level for which <1% of functions obtained after shuffling the stimulus labels had a defined latency (0.156). Latency, deep: 75.8 ms, 95% CI [68.4 85.2]; granular: 94.7 ms, 95% CI [82.2 105.7]; superficial: 97.7 ms, 95% CI [78.0 103.7]. **Bootstrap procedure (see Methods) p = 0.006; *p = 0.018. See also Figure 2—figure supplements 2 and 3. (F) Border ownership reliability calculated in a 100 ms sliding window for the three laminar compartments. Colors as in E. Colored lines in top of panel indicate the earliest crossing for ≥20 adjacent ms with the threshold, defined similarly as for panel E. Threshold crossings: deep 89.9 ms, 95% CI [81.5 99.9]; granular 105.4 ms, 95% CI [94.9 114.6]; superficial 109.4 ms, 95% CI [98.9 124.9]. **Bootstrap procedure p = 0.006; *p = 0.015.
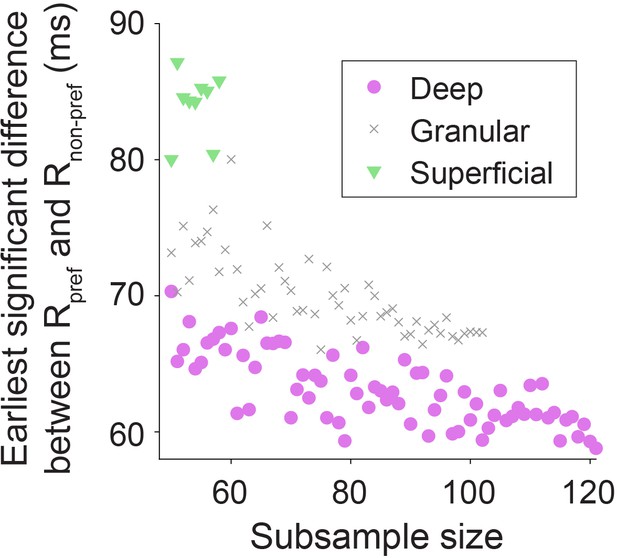
Differences in sample size do not explain differences in latency between layers.
The latency of significant difference between responses to preferred and non-preferred sides of border ownership was determined as in Figure 2B–D, for different subsample sizes (subsampling well-isolated units without replacement). Each subsample size was sampled five times and the average latency is plotted. The result shows that while the total number of units in each layer did differ, the shorter latency for deep layer units than for granular or superficial layer units consistently appeared at all subsample sizes (consistent with data shown in Figure 2B–D), and is not explained by differences in sample size between compartments.
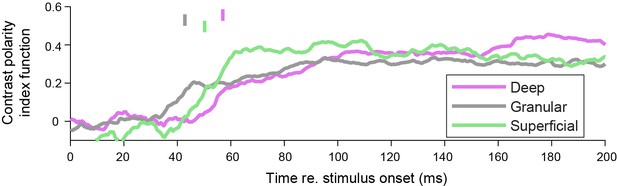
Selectivity for contrast polarity does not occur earliest in the deep layers.
Same analysis as Figure 2E, but for contrast polarity instead of border ownership, for the same units as in Figure 2E. Latency at same threshold as in Figure 2E: deep: 57.0 ms, 95% CI [49.9 75.0]; granular: 42.8 ms, 95% CI [35.4 60.8]; superficial: 50.2, 95% CI [40.4 56.4]. Granular versus deep: bootstrap procedure p = 0.14. Granular versus superficial: p = 0.12. That border ownership units are often selective for contrast polarity is consistent with a prior study (Zhou et al., 2000).
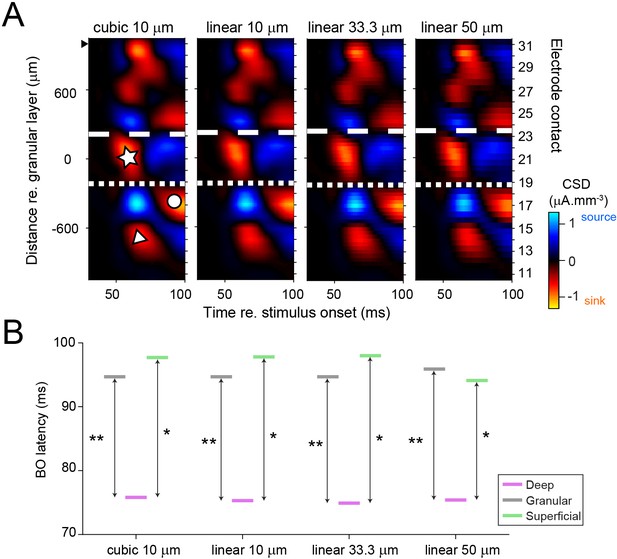
Laminar analysis is not affected by how the current source density (CSD) maps were smoothed.
(A) Example CSD map smoothed with different methods. Leftmost panel corresponds to the cubic interpolation with 10 µm spatial resolution that was used in the main analysis. Other panels are computed with 2D linear interpolation (MATLAB function interp2 with option linear), with varying degrees of spatial resolution (respectively, 10, 33.3, and 50 µm). Boundaries between laminar compartments are drawn for each smoothing method separately for the population of penetrations (to ensure that we did not bias the results we blinded ourselves to the original layer assignment when applying each method). White dashed and dotted lines show boundary positions for the example penetration for each smoothing method. In the population, the assigned laminar compartment was the same as that when the standard interpolation method was used (cubic 10 µm) for 98.1 % (linear 10 µm), 97.7 % (linear 33.3 µm), and 97.1 % (linear 50 µm) of well-isolated units (n = 685). (B) After performing the laminar assignment separately for the different smoothing methods (see A), latencies were computed on border ownership (BO) index functions for the population of well-isolated units (as in Figure 2E). Irrespective of the method used, the BO latency of deep layer units was always significantly shorter than that of granular layer units and superficial layer units. *p < 0.05 (exact p values from left to right: 0.018, 0.018, 0.015, and 0.025); **p < 0.01 (exact p values from left to right: 0.006, 0.004, 0.005, and 0.0025).
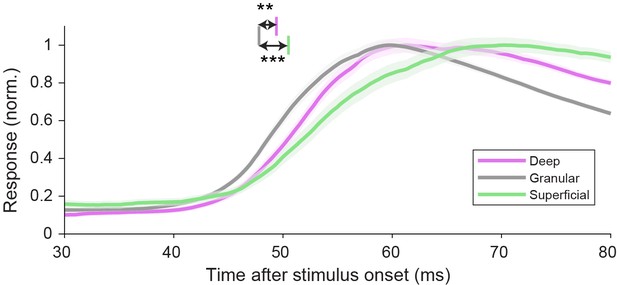
Spike latencies evoked by flashed stimuli appearing in the classical receptive field are shortest in the granular layer.
Response time courses of spikes evoked by small rings centered on the classical receptive field, for well-isolated units recorded in each laminar compartment (mean ± standard error of the mean [SEM]). Vertical lines indicate mean latencies for each laminar compartment (defined as crossing of the functions with a threshold value a third of the way from baseline to peak, 0.435). Deep: 49.4 ms, 95% CI [48.3 50.3], 173 units; granular: 47.8 ms, 95% CI [47.2 48.5], 152 units; superficial: 50.5 ms, 95% CI [49.1 52.2], 101 units. ***Bootstrap procedure p = 0.0005; **p = 0.002.
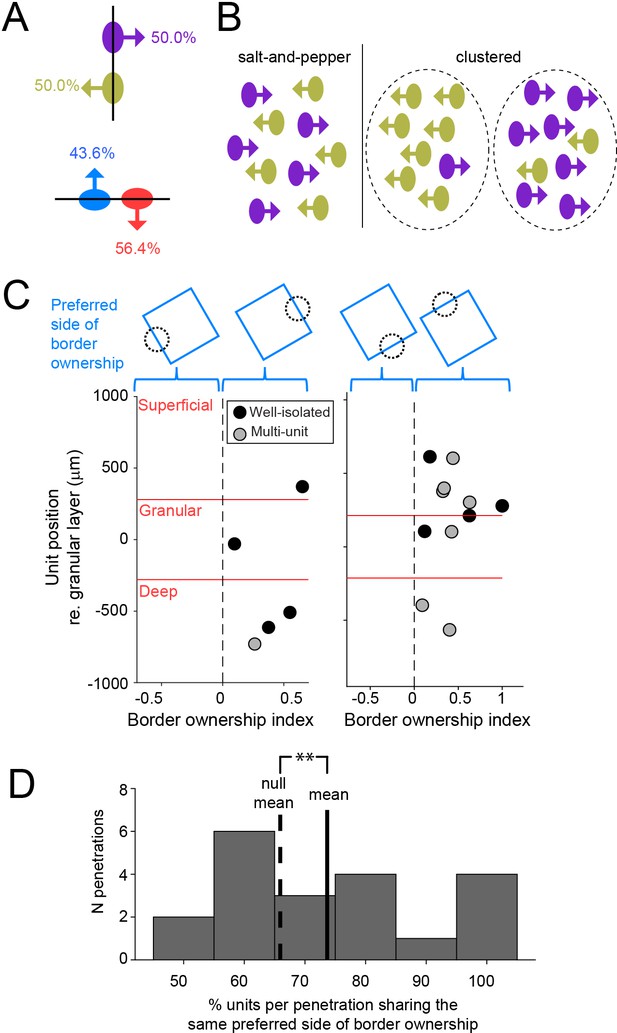
Preferred side of border ownership is clustered.
(A) For a given edge, there are two possibilities for preferred side of border ownership. Percentages indicate the fraction of well-isolated units selective for border ownership that have the preferred side of border ownership indicated by the arrow (vertical edge: n = 64; horizontal edge: n = 78). See also Figure 4—figure supplement 1. (B) Cartoon showing possibilities for spatial organization of neurons with opposite preferred sides of border ownership, for a vertical edge. (C) Each panel shows border ownership-selective units recorded during one penetration. The abscissa shows the signed BOI of each unit (sign indicates preferred side of border ownership, shown above the panels). Ordinate shows the position of the units relative to the center of the granular layer. (D) Histogram of the proportion of units per penetration that shares the same preferred side of border ownership, for all penetrations with at least four border ownership-selective well-isolated and multiunits spanning from superficial to deep cortical layers. Solid line is distribution mean, dashed line shows null distribution mean. **p = 0.003 (20 penetrations with 136 units).
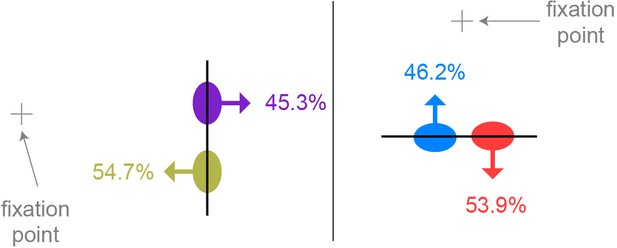
Similar to Figure 4A, but expressing preferred side of border ownership relative to the position of the fixation point.
Together with Figure 4A, this indicates that similarly sized populations of border ownership units with opposite preferences occur in the same visual quadrants. Vertical edge: n = 64 well-isolated units selective for border ownership; horizontal edge: n = 78 well-isolated units selective for border ownership.
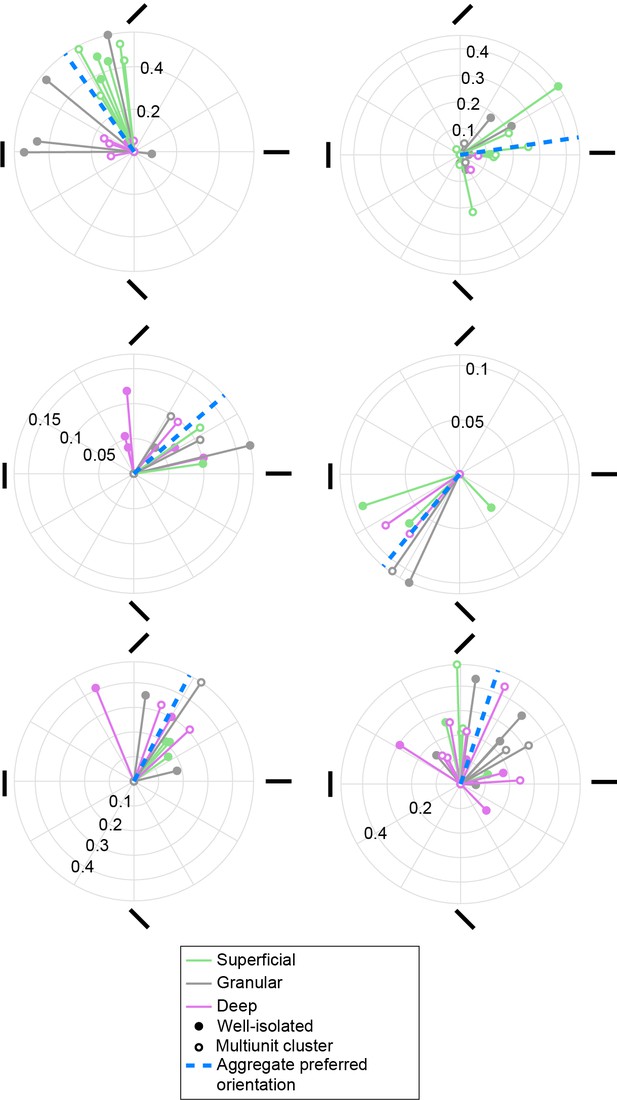
Columnar clusters of preferred orientation in V4.
Polar plots are shown for six penetrations with at least four orientation-selective units distributed across all three laminar compartments. Each solid vector corresponds to one unit and indicates the preferred orientation (direction) and the degree of orientation tuning (magnitude), quantified, respectively, as the direction and magnitude of the resultant vector of the responses to each orientation. Color indicates the laminar compartment. Open symbols indicate multiunits, closed symbols indicate well-isolated units. Blue dashed line indicates the preferred orientation across units in a penetration (aggregate preferred orientation). All six penetrations shown have a significant aggregate preferred orientation.
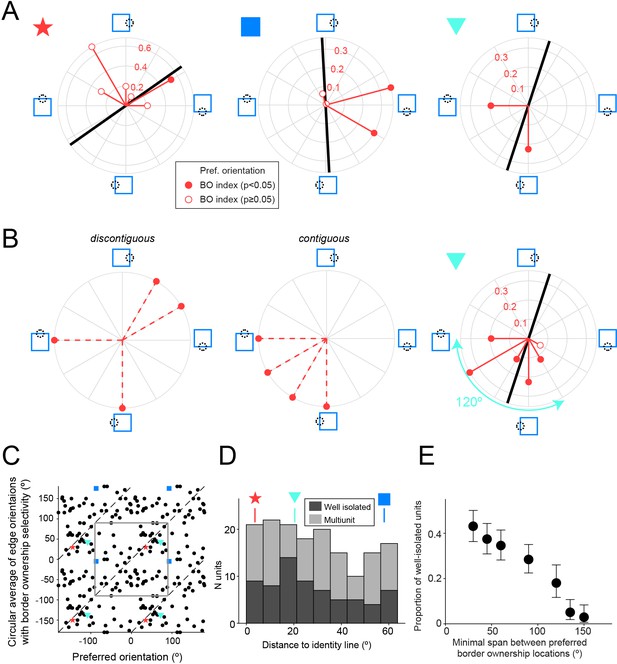
Border ownership selectivity often occurs far away from the preferred orientation.
(A) Polar plots for three example units that were tested for border ownership selectivity with edges of multiple orientations (orientations of red vectors indicate tested orientations). Polarity of red vector relative to origin indicates the preferred side of border ownership for each edge orientation, according to the stimulus cartoons surrounding the plot, and filled circles indicate cases with statistically significant border ownership selectivity (adjusted for multiple comparisons using Bonferroni correction). Magnitude of red vectors corresponds to |BOI|. Black line indicates preferred edge orientation. Colored symbols on the upper left side of each polar plot correspond to the symbols in C and D. See also Figure 6—figure supplement 1. (B) Left and middle: for units showing border ownership selectivity to orthogonal edges there are two possibilities for the intermediate edge orientations. Either the preferred sides of border ownership are spatially discontiguous with those for the orthogonal edges (left), or they are contiguous (middle). Right: polar plot for the same unit as in A (right polar plot), now including data for the orientations that were not shown in A. (C) Scatter plot comparing the preferred orientation for edges (abscissa) with the circular mean of edge orientations for which border ownership selectivity is significant (ordinate), for all units that were tested for border ownership for at least four orientations (n = 68 well-isolated units). Note that each data point is plotted four times, because both variables are periodic and two periods are shown for each. The gray square outlines an area corresponding to one period for both variables. Dashed lines indicate identical values. Colored symbols correspond to the units in A, B, and D. (D) Smallest orthogonal distance to the identity line for the units shown in C. The data are significantly biased toward zero (see Results). Distance values for the example units shown by colored symbols in A–C are indicated above the histogram. (E) Proportion of border ownership well-isolated units where the preferred sides of border ownership cover an angular span at least as wide as the value indicated along the abscissa (for all units tested with such spans). For example, the unit in B (right) has a span of preferred sides of border ownership of 120°. Note that the span between spatially contiguous preferred sides of border ownership is necessarily <180°. N units tested for each value of on the abscissa: ≥30°: 211; ≥45°: 211; ≥60°: 211; ≥90°: 211; ≥120°: 122; ≥135°: 118; ≥150°: 102.
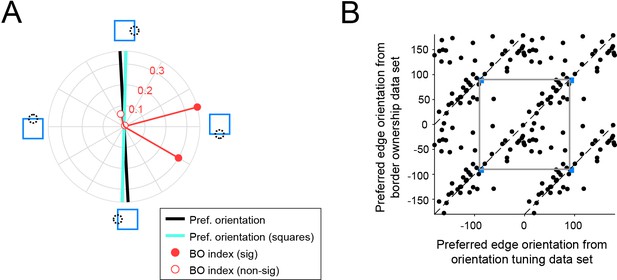
Consistent orientation tuning for isolated edges and for edges that are part of squares.
(A) Same plot as in Figure 6A (middle), but including preferred edge orientation derived from the border ownership stimulus set (cyan line). (B) Preferred edge orientation derived from the border ownership stimulus set (edges that are part of squares) plotted against preferred edge orientation derived from the orientation tuning data set (isolated contrast edges). As in Figure 6C, data points are plotted repeatedly every 180° for these periodic variables and two periods are shown. The gray square outlines an area corresponding to one period for both variables. Dashed lines indicate identical values. Blue square indicates the unit shown in A. Values are significantly clustered along the identity line (randomization test p = 0.0005; n = 51 units).
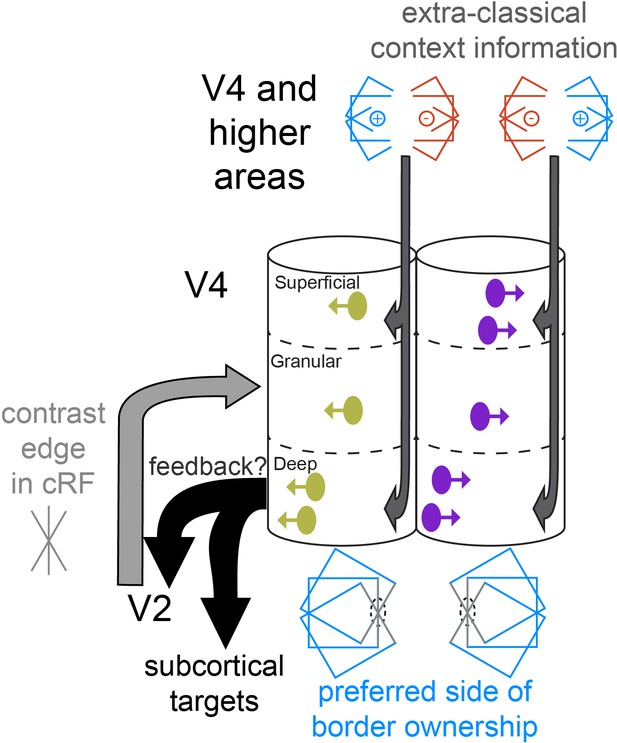
Cartoon of a conceptual model of columnar processing of border ownership, supported by our data.
Two columnar structures are shown, each of which responds to vertical edges in the classical receptive field (cRF), but they have opposite preferred sides of border ownership (indicated by the direction of the arrows on the units in the columns, and by the symbol below each column). Such columns may or may not be adjacent. The earliest occurrence of border ownership selectivity in the deep layers is symbolized by the positon of deep layer units near the left edge of the column. Light and dark gray arrows indicate where different types of stimulus information likely arrive first. Stimulus information in the cRF (gray edge) arrives first in the granular layer. Deep layer neurons may first compute border ownership selectivity by integrating this information with contextual information that arrives in superficial and deep layers, possibly provided by corticocortical feedback and horizontal connections. The content of these context signals is necessarily asymmetric to result in border ownership preference, and different for the two columns shown (indicated by the symbols above the columns). Because of their prominence in deep layers, early border ownership signals may be part of the feedback that projects from V4 to upstream areas (e.g., V2), and to subcortical targets, such as the superior colliculus (black arrows). Later on during the response, after border ownership signals have first been established in V4, the feedforward input from V2 to V4 likely also contains border ownership signals, since roughly half of V2 units are selective for border ownership (Zhou et al., 2000).
Tables
Border ownership selectivity is more common in orientation-selective well-isolated units but often occurs in units that are not selective for orientation.
Border ownership selectivity | |||
---|---|---|---|
− | + | ||
Orientation selectivity | − | 79 | 46 |
+ | 133 | 152 |
Reagent type (species) or resource | Designation | Source or reference | Identifiers | Additional information |
Strain, strain background (Macaca mulatta, male) | Macaque | UC Davis | ||
Software, algorithm | MATLAB | The Mathworks | ||
Software, algorithm | MonkeyLogic | Hwang et al., 2019 | https://monkeylogic.nimh.nih.gov/; https://www.brown.edu/Research/monkeylogic/ | |
Software, algorithm | CircStat | Berens, 2009 | ||
Software, algorithm | SpyKING CIRCUS | Yger et al., 2018 | ||
Other | 32-channel multielectrode probe | ATLAS Neuroengineering | E32+ R-100-S1-L10 NT |