Chemoreceptor co-expression in Drosophila melanogaster olfactory neurons
Figures
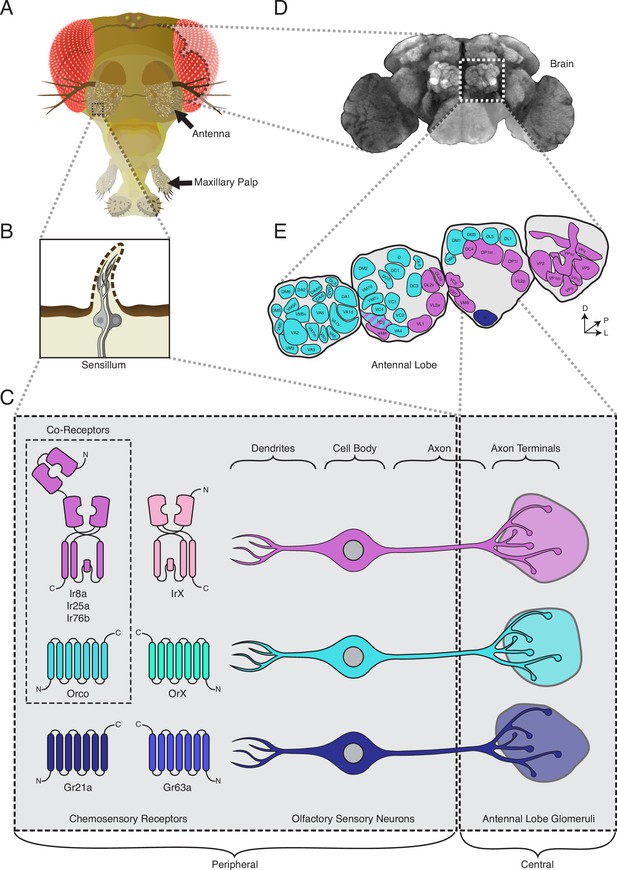
The standard view of olfactory receptor expression in Drosophila melanogaster.
(A) The adult fly head (left) has two olfactory organs: the antennae and the maxillary palps (arrows). Olfactory neurons from these organs project to the fly brain (D), to the first center involved in processing of olfactory information, the antennal lobes (E). (B) The olfactory organs are covered by sensory hairs called sensilla (left). Each sensillum contains between one and four olfactory sensory neurons (two example neurons are shown in gray). The dendrites of these neurons extend into the sensilla, and the axons target discrete regions of the antennal lobes called glomeruli (E). Neuronal compartments (dendrites, cell body, axon, axon terminals) are labeled in (C). ( C) Left: in the periphery, each olfactory sensory neuron is traditionally thought to express chemosensory receptors from only one of three gene families on its dendrites: ionotropic receptors (IRs, pink and purple), odorant receptors (ORs, teal and green), or gustatory receptors (GRs, light and dark blue). IRs and ORs require obligate co-receptors (dotted box outline) to form functional ion channels. All ORs utilize a single co-receptor, Orco (teal), while IRs can utilize one (or a combination) of three possible co-receptors (purple): Ir8a, Ir25a, or Ir76b. The two GRs form a functional carbon dioxide detecting channel expressed in only one class of neurons. All other olfactory neurons express one of the four co-receptors. Right: olfactory sensory neurons expressing ORs, IRs, and GRs are thought to project to mutually exclusive glomeruli in the antennal lobe (AL) of the central brain, forming the olfactory map shown in (E). (D) Fly brain stained with anti-brp synaptic marker (nc82), with left AL outlined by the dotted white box. (E) AL map with glomeruli color-coded by the chemosensory receptors (ORs, IRs, or GRs) expressed in the olfactory sensory neurons projecting to them. Only one glomerulus (VC3, striped) receives inputs from neurons expressing chemoreceptors from multiple gene families (ORs and IRs). Compass: D = dorsal, L = lateral, P = posterior.
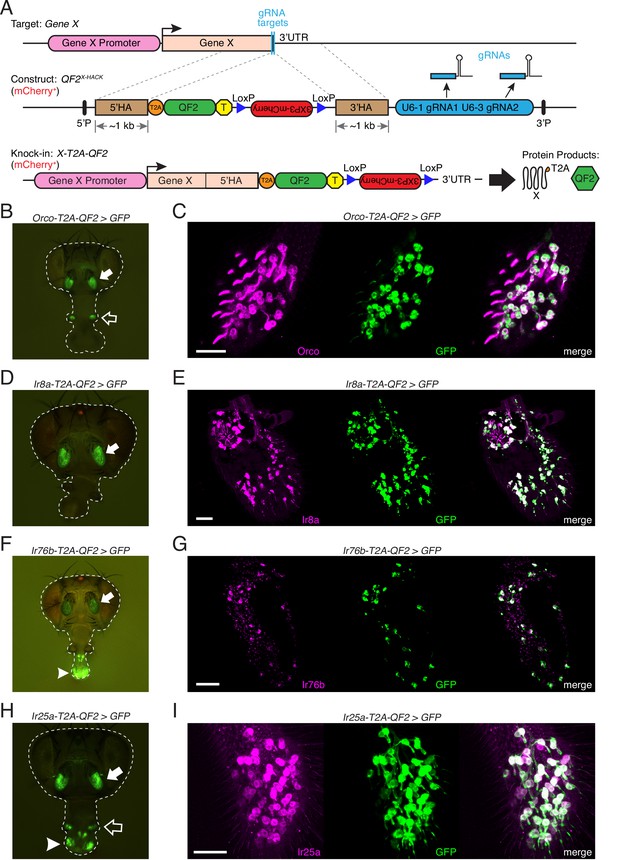
Generation and validation of chemosensory co-receptor knock-in lines.
(A) Schematic of HACK knock-in approach. Top: two double-stranded breaks are induced on either side of the target gene stop codon with gRNAs (blue) expressed from the QF2X-HACK construct (middle) in the presence of Cas9. The construct includes T2A-QF2 and a floxed 3XP3-mCherry marker. The knock-in introduces a transcriptional stop (yellow T) after QF2. Bottom: the knock-in produces two protein products (right) from the targeted mRNA: target X and the QF2 transcription factor (Diao and White, 2012). The X-T2A-QF2 knock-in can be crossed to a reporter (e.g., QUAS-GFP) to examine the endogenous expression pattern of the target gene. (B) Orco-T2A-QF2 driving QUAS-GFP in adult fly head. GFP expression is found in the antennae (filled arrow) and maxillary palps (hollow arrow), as previously reported (Larsson et al., 2004). (C) Whole-mount anti-Orco antibody staining in Orco-T2A-QF2>GFP maxillary palps reveals a high degree of overlap of Orco+ and GFP+ cells. N = 3. (D) Ir8a-T2A-QF2 drives GFP in the antennae (arrow), as previously reported (Abuin et al., 2011). (E) Anti-Ir8a antibody staining of Ir8a-T2A-QF2>GFP antennal cryosections shows high correspondence between Ir8a+ and GFP+ cells. N = 7. (F) Ir76b-T2A-QF2 drives GFP expression in the antennae (filled arrow) and labella (hollow arrow), reflecting Ir76b’s role in olfaction and gustation, respectively (Benton et al., 2009; Zhang et al., 2013). (G) In situs on Ir76b-T2A-QF2>GFP antennal cryosections to validate that the knock-in faithfully recapitulates the endogenous expression pattern. N = 3. (H) Ir25a-T2A-QF2 drives GFP in the antennae (filled arrow) and labella (hollow arrow), which has been reported previously (Benton et al., 2009; Croset et al., 2010). Expression in the maxillary palps (arrowhead) has not been previously reported. (I) Whole-mount maxillary palp staining with an anti-Ir25a antibody in Ir25a-T2A-QF2>GFP flies. The knock-in and Ir25a antibody co-labeled the majority of olfactory neurons in the palps. N = 5. Scale bars = 25 µm. In (D) and (F), the 3XP3-mCherry knock-in marker can be weakly detected in the eyes and ocelli (red spot) of both Ir8a-T2A-QF2 and Ir76b-T2A-QF2. See also Figure 2—figure supplements 1–4, Tables 1 and 2, Table 1—source data 1, Figure 2—source data 1, and Materials and methods.
-
Figure 2—source data 1
Single sensillum recordings (SSRs) of knock-in lines.
- https://cdn.elifesciences.org/articles/72599/elife-72599-fig2-data1-v2.xlsx
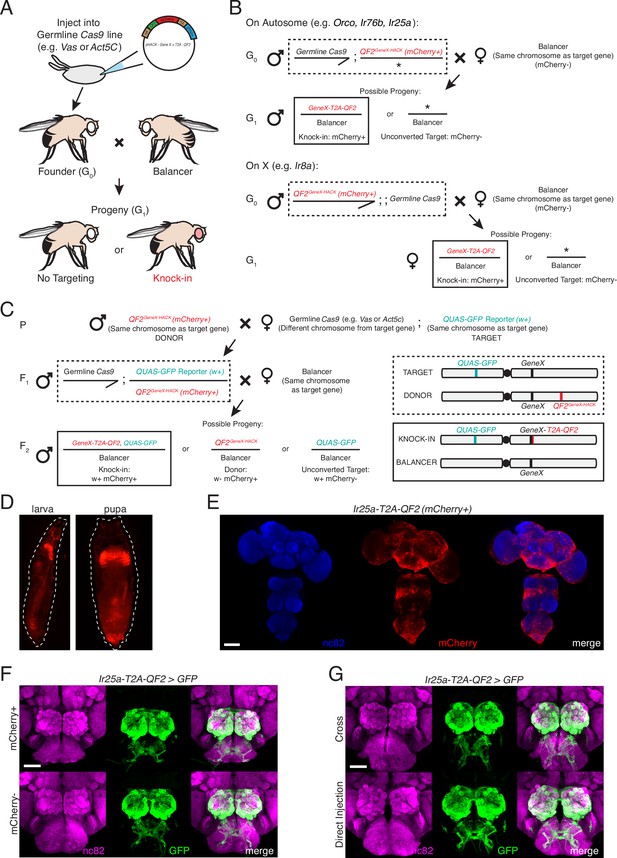
HACK crossing schematics, marker expression, and approach comparison.
(A) Schematic of the direct injection method to generate a HACK knock-in. The HACK KI construct is injected into a germline Cas9-expressing embryo (both Act5C-Cas9 and Vas-Cas9 yielded successful knock-ins; see Materials and methods for more details). This approach requires one genetic cross to generate a knock-in: injected founder flies (G0) are individually crossed to balancer flies, and progeny (G1) are screened for the mCherry knock-in marker. Each mCherry+ G1 represents an independent knock-in event; thus, single mCherry+ flies are used to establish knock-in lines. (B) Details of the genetic crosses used to establish knock-in lines for each target gene using the direct injection approach. For genes on autosomes (Orco and Ir76b, third chromosome; Ir25a, second chromosome), a Cas9 line on the X chromosome was used, and mCherry+ male progeny selected to remove the Cas9 after successful HACKing. For Ir8a on the X chromosome, a Cas9 line on the third chromosome was used, which was marked with GFP to facilitate its removal in subsequent generations. In addition to mCherry marker screening, PCR genotyping, and sequencing, knock-in lines were confirmed by crossing to a QUAS reporter line and checking brain expression, either at the G2 stage, or by crossing G0 flies to balanced reporter lines to immediately check G1 brain expression. (C) Details of using the crossing method to generate HACK knock-ins. This involves the creation of donor lines, in which the HACK targeting construct is inserted into the genome, such as through P-element insertion or ΦC31 integration. Donor lines are crossed to a germline Cas9 line (Parental). The progeny, F1, have all the components necessary for a knock-in to occur (gRNAs, Cas9, HDR cassette). An additional cross is required to select germline HACKing events at the F2 generation. Because the donor lines also express mCherry, as do the knock-ins, the target chromosome must be marked with a different marker (such as w+) for knock-in selection (see chromosome schematics at the right, illustrating the locations of the genetic elements in the F1 and F2 generations). F2 knock-ins will have both markers (in this case, mCherry+w+), while donor flies will only have one (mCherry+w-). The cross approach was tested with two of the four genetic targets (Orco and Ir25a) and successfully produced knock-in lines for both. However, the cross approach can produce false positives (see Table 1 and Table 1—source data 1) and is more time consuming. The direct injection approach is recommended for the generation of targeted knock-ins. (D) Knock-in screening can be performed at the larval (left) and pupal (right) stages. (E) The 3XP3-mCherry marker is expressed throughout the adult fly brain and ventral nerve cord. Nc82 channel has been pseudo-colored blue for clarity. (F) The marker cassette is flanked by loxP sites and can be removed through Cre recombination (see Materials and methods for details) (Siegal and Hartl, 1996). The 3XP3-mCherry marker does not affect knock-in expression. Ir25a-T2A-QF2-driven GFP expression in the brain was similar before (top row) and after (bottom row) Cre-mediated removal of the mCherry marker. (G) No difference was found in the expression patterns of Ir25a-T2A-QF2 knock-in lines generated by the two HACK methods, genetic cross (top) and direct injection (bottom). For (F) and (G): N = 5 for direct injection; N = 4 for cross, mCherry+; N = 3 for cross, mCherry-. Expression patterns were comparable across all three groups. See also Table 1 and Table 1—source data 1. Scale bars: 100 µm in (E), 50 µm in (F, G).
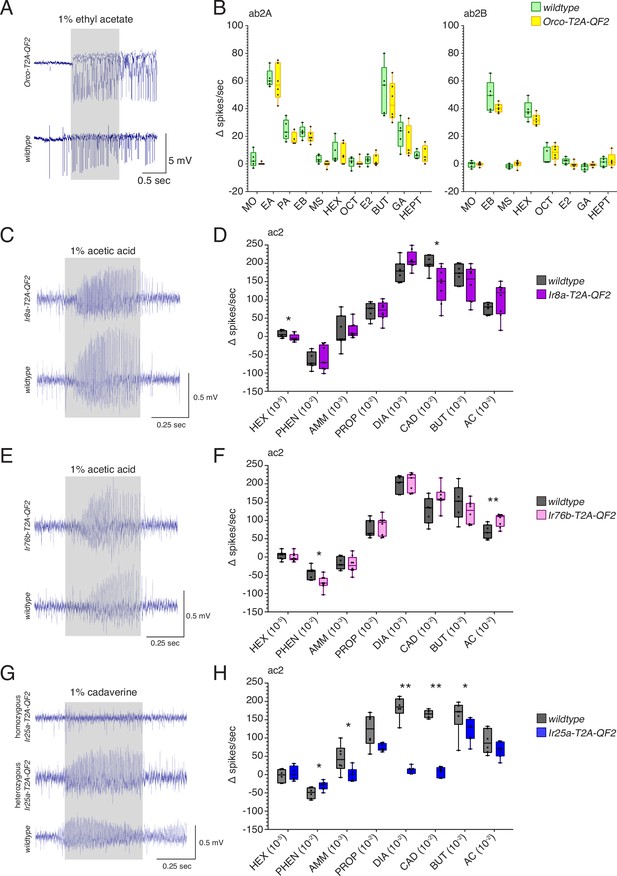
T2A-QF2 HACK knock-in effects on target gene function.
(A) Orco knock-in single sensillum recording (SSR) experiments were performed in ab2 sensilla. Responses to 10 odorants plus mineral oil control were compared in wildtype and homozygous Orco-T2A-QF2 sensilla. Shown are example responses to 1% ethyl acetate. (B) ab2 sensilla contain two neurons: A (left) and B (right). No differences were found in the responses of these neurons between the two genotypes across all odorants tested (Mann–Whitney U test, p>0.05). A small difference in baseline (pre-stimulus) activity was observed, which can be seen in (A). Fewer stimuli are shown for ab2B because odorants strongly activating the A neuron obscured the B neuron responses. N = 5 for wildtype, N = 6 for Orco-T2A-QF2. MO: mineral oil; EA: ethyl acetate; PA: pentyl acetate; EB: ethyl butyrate; MS: methyl salicylate; HEX: hexanol; OCT: 1-octen-3-ol; E2: e2-hexenal; BUT: 2,3-butanedione; GA: geranyl acetate; HEPT: 2-heptanone. (C–H) IrCo knock-in SSR experiments were performed in ac2 sensilla. Responses to eight odorants were compared in wildtype and homozygous knock-in flies. (C) Example responses to 1% acetic acid in Ir8a-T2A-QF2 and wildtype sensilla. (D) There was a small but significant difference in responses between the two genotypes to hexanol (Mann–Whitney U test, p=0.0286) and cadaverine (Mann–Whitney U test, p=0.0106), which are not typically considered to be Ir8a-dependent odorants (Silbering et al., 2011). Responses to all other stimuli were not significantly different between genotypes. N = 7 for wildtype, N = 8 for Ir8a-T2A-QF2. (E) Example responses to 1% acetic acid in Ir76b-T2A-QF2 and wildtype flies. (F) There were small but significant differences in responses to phenethylamine (Mann–Whitney U test, p=0.012) and acetic acid (Mann–Whitney U test, p=0.0087) between Ir76b-T2A-QF2 and wildtype flies. Responses to the other stimuli were not significantly different between genotypes. N = 7 for wildtype, N = 8 for Ir76b-T2A-QF2. (G) Homozygous Ir25a-T2A-QF2 flies lost responses to most amines, recapitulating an Ir25a mutant phenotype (Abuin et al., 2011; Silbering et al., 2011). Heterozygous Ir25a-T2A-QF2 flies with one wildtype copy of Ir25a had normal responses. Shown are example responses to 1% cadaverine in homozygous Ir25a-T2A-QF2, heterozygous Ir25a-T2A-QF2, and wildtype ac2 sensilla. (H) Homozygous Ir25a-T2A-QF2 flies had strongly reduced or abolished responses to phenethylamine (Mann–Whitney U test, p=0.039), ammonia (Mann–Whitney U test, p=0.0338), 1,4-diaminobutane (Mann–Whitney U test, p=0.0012), cadaverine (Mann–Whitney U test, p=0.0025), and 2,3-butanedione (Mann–Whitney U test, p=0.0472). Responses to the other stimuli were not significantly different between genotypes. N = 6 for wildtype, N = 7 for homozygous Ir25a-T2A-QF2. PO: paraffin oil; HEX: hexanol; PHEN: phenethylamine; AMM: ammonia; PROP: propanal; DIA: 1,4-diaminobutane; CAD: cadaverine; BUT: 2,3-butanedione; AC: acetic acid. See Figure 2—source data 1 for all U and p-values.
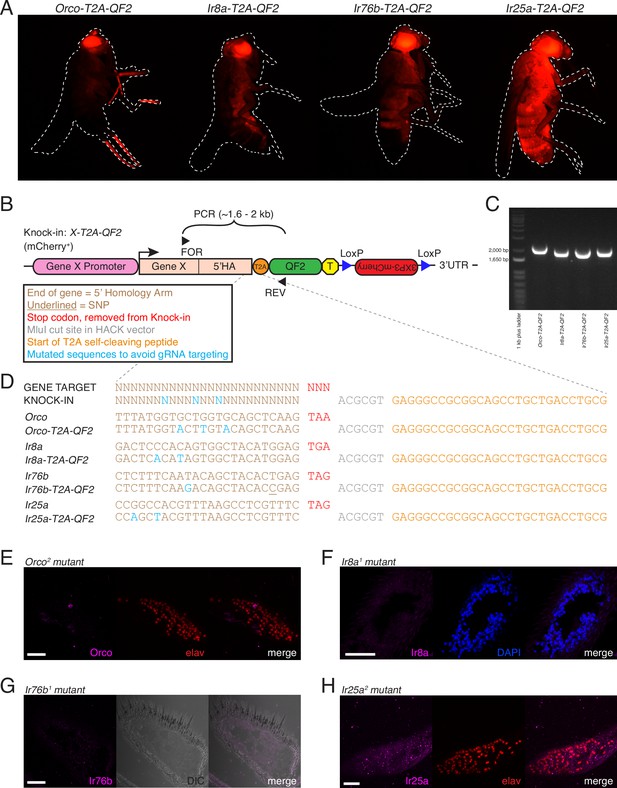
Additional validation of co-receptor knock-in lines.
(A) The HACK 3XP3-mCherry marker is expressed in the eyes and broadly throughout the fly nervous system and is subject to positional effects in expression. (B) Each knock-in line is generated by CRISPR-Cas9-mediated homology-directed insertion of a T2A-QF2 cassette marked by mCherry in front of the translational stop codon of the target gene. The 5′ homology arm (5′HA) is ~1 kb of the gene just before the stop codon. Successful knock-in lines were confirmed using forward primers upstream of the 5′HA (FOR), and a reverse primer within the QF2 sequence (REV). Amplification of an ~1.6–2 kb sequence occurs only if a knock-in is present. See Figure 2A for target and donor construct schematics. (C) PCR genotyping using the primers shown in (B) confirms successful knock-in of the four targeted genes. (D) Knock-ins were confirmed with sequencing of the targeted genomic site. For each co-receptor, the first line shows the target sequence (based on the FlyBase reference genome) (Thurmond et al., 2019), and the second line shows the verified sequence of the knock-in. The stop codon (red) has been replaced with the HACK T2A-QF2 cassette. The portion shown here includes the MluI restriction site used for cloning the 5′HA (gray), as well as the start of the T2A sequence (orange). In each knock-in construct, synonymous substitutions (blue) have been made to prevent the gRNA/Cas9 from cutting the donor construct. Successful knock-in ensures substitution of the gRNA recognition sequences and protects the genomic DNA from further gRNA/Cas9 targeting. Each knock-in construct was designed with three substitutions. The underlined base pair in the Ir76b knock-in is an SNP discovered in the lab wildtype stock and considered when designing the knock-in construct. (E.–H) Antibody and in situ probe validation. (E) No anti-Orco expression in Orco2 mutant palps. elav is used as a pan-neuronal counterstain. (F) No anti-Ir8a expression in Ir8a1 mutant antennae. DAPI is used as a cellular counterstain. (G) No Ir76b probe signal in Ir76b1 mutant antennae. DIC used to visualize tissue. (H) No anti-Ir25a expression in Ir25a2 mutant palps (elav counterstain). Scale bars = 25 µm. See also Table 2.
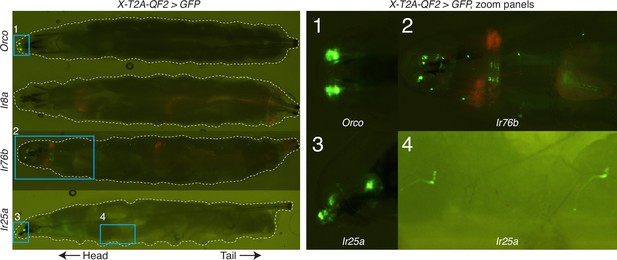
Knock-in expression in the larva.
In the larval stage, Orco-T2A-QF2 (first row) appears to drive expression only in the dorsal organs (zoom panel 1), as previously described (Larsson et al., 2004); Ir8a-T2A-QF2 (second row) does not appear to drive GFP expression in the larva (although very weak expression may not be visible at this magnification); and both Ir76b-T2A-QF2 (third row) and Ir25a-T2A-QF2 (fourth row) drive GFP expression in the larval head as well as throughout the body wall (zoom panel 2 for Ir76b; zoom panels 3 and 4 for Ir25a), reflecting the role of these genes in additional, non-olfactory modalities. Weak red fluorescence from the 3XP3-mCherry knock-in marker can be detected in the Ir8a-T2A-QF2 and Ir76b-T2A-QF2 larvae. This is due to channel bleed-through and is not seen in the other knock-in lines (Orco-T2A-QF2 and Ir25a-T2A-QF2) that have had the marker removed (see Materials and methods for details).
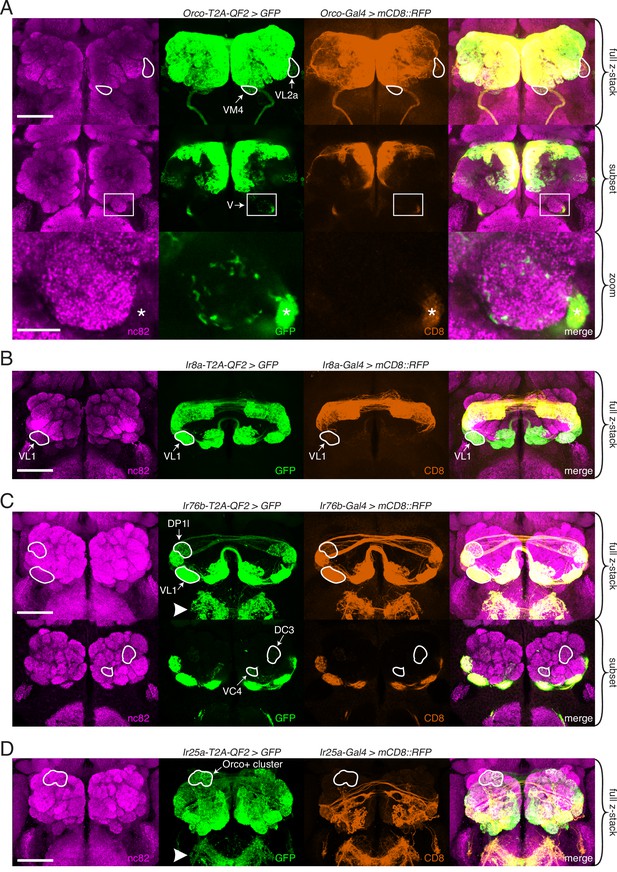
Expanded expression of olfactory co-receptors.
(A–D) Comparing knock-in innervation patterns of the antennal lobe (AL) with what has previously been reported for each co-receptor. Co-labeling experiments with each co-receptor knock-in line driving QUAS-GFP (green) and the corresponding transgenic co-receptor Gal4 line driving UAS-mCD8::RFP (anti-CD8, orange). The nc82 antibody labels synapses (magenta) and is used as a brain counterstain in these and all subsequent brain images. (A) The Orco-T2A-QF2 knock-in labels more glomeruli than the Orco-Gal4 line. Top: maximum intensity projection of full z-stack showing two additional glomeruli labeled by the knock-in, VM4 (Ir8a+/Ir76b+/Ir25a+) and VL2a (Ir8a+). Middle: subset of z-stack with a box around the V glomerulus. Bottom: zoom of boxed region showing sparse innervation of the V glomerulus (Gr21a+/Gr63a+) by the knock-in but not the Gal4 line. Asterisk indicates antennal nerve that is outside the V glomerulus. In the sub z-stack and zoom panel, gain has been increased in the GFP channel to visualize weak labeling more clearly. (B) The Ir8a-T2A-QF2 knock-in also drives GFP expression in more glomeruli than previously reported, including the outlined VL1 glomerulus (Ir25a+). (C) In the brain, Ir76b-T2A-QF2>GFP olfactory neurons innervate the ALs, while gustatory neurons from the labella innervate the subesophageal zone (SEZ, arrowhead). Top: both the Ir76b knock-in and transgenic Gal4 line label more glomeruli than previously reported, including VL1 (Ir25a+) and DP1l (Ir8a+). Bottom: the Ir76b-T2A-QF2 knock-in labels several Orco+ glomeruli, such as DC3 and VC4 (outlined). In the subset, gain has been increased in the GFP channel to visualize weakly labeled glomeruli more clearly. (D) The Ir25a-T2A-QF2 knock-in drives GFP expression broadly in the antennal lobes and SEZ (arrowhead). Ir25a+ neurons innervate many Orco+ glomeruli, such as those outlined. The transgenic Ir25a-Gal4 line labels a subset of the knock-in expression pattern. N = 3–10 for co-labeling experiments, N = 5–15 for additional analyses of the knock-in lines alone. Scale bars = 25 µm, except zoom panel scale bar = 10 µm. See also Figure 3—figure supplements 1 and 2, Table 3, and Figure 3—source data 1 and Figure 3—source data 2.
-
Figure 3—source data 1
Knock-in antennal lobe analyses.
- https://cdn.elifesciences.org/articles/72599/elife-72599-fig3-data1-v2.xlsx
-
Figure 3—source data 2
Examples of new glomerular expression in knock-in lines.
- https://cdn.elifesciences.org/articles/72599/elife-72599-fig3-data2-v2.pdf
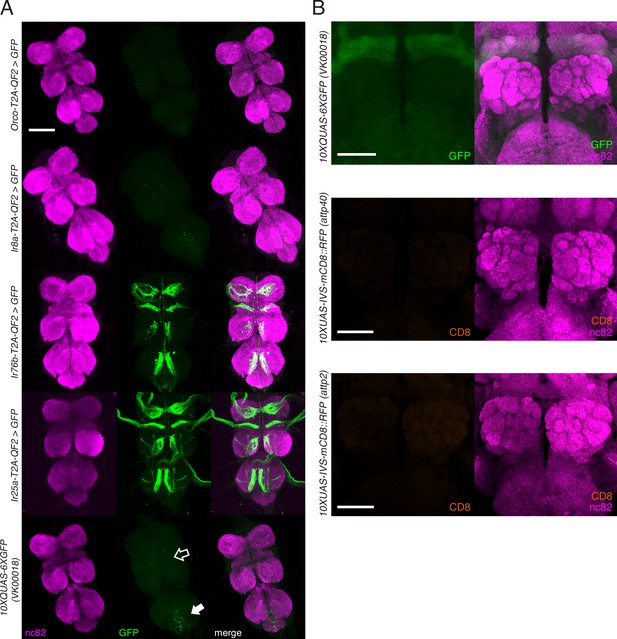
Knock-in expression in the adult ventral nerve cord (VNC) and reporter expression in the brain.
(A) In adult flies, two of the four knock-ins (Ir76b-T2A-QF2, third row, and Ir25a-T2A-QF2, fourth row) drive GFP expression in neurons innervating the VNC. This likely reflects the role of these co-receptors in gustation. There is weak GFP expression in the reporter control (bottom row) in the abdominal neuromere (filled arrow), as well as the accessory mesothoracic neuropil (empty arrow), which can be seen in the two other knock-ins (Orco-T2A-QF2, first row, and Ir8a-T2A-QF2, second row). This nonspecific expression is likely from the reporter itself rather than driven by the two knock-ins. (B) Control brains showing the QUAS-GFP reporter alone (top) and the UAS-mCD8::RFP reporters alone (middle and bottom). These reporters were used for all brain images in Figures 3—5. The QUAS reporter weakly labels the mushroom bodies. The VNC from the fly in (A, fourth row) is also used in Figure 2—figure supplement 1E. Scale bars: 100 µm in (A), 50 µm in (B).
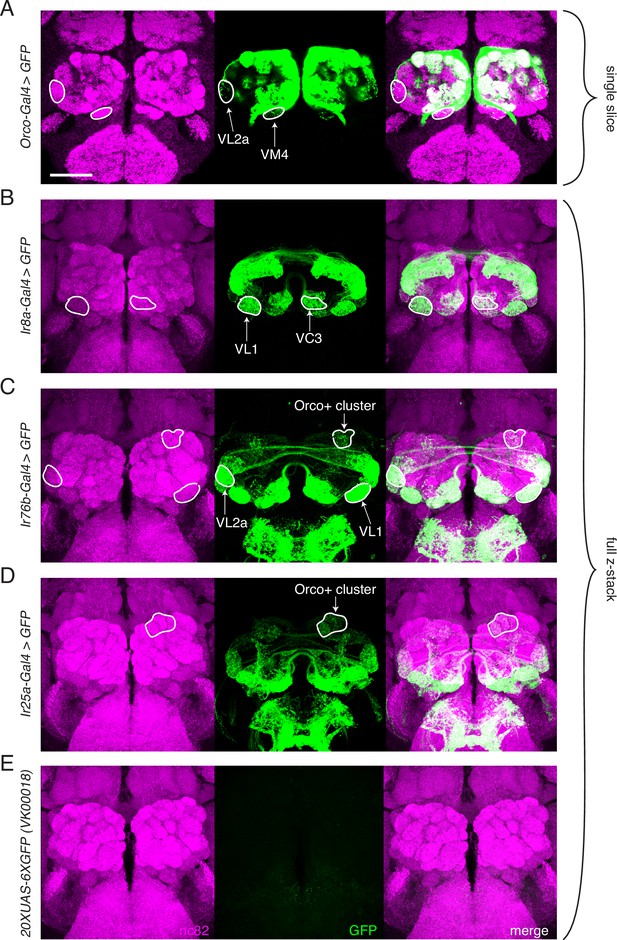
Transgenic co-receptor Gal4 lines do not fully recapitulate knock-in expression.
(A–E) Transgenic co-receptor Gal4 lines crossed to a strong UAS reporter reveal expression in some, but not all, of the additional glomeruli labeled by the respective knock-ins. Some discrepancies between the Gal4 and knock-in lines are enumerated here, but do not represent a comprehensive list. (A) Orco-Gal4 drives weak GFP expression in VL2a and VM4 glomeruli (outlined), but lacks expression in DL2, V, and VL1 glomeruli, which are labeled by the knock-in. (B) Ir8a-Gal4>GFP labels VC3 and VL1 (outlined), but lacks expression in the VM4 and VA3 glomeruli, which are labeled in the knock-in. (C) Ir76b-Gal4 labels the VL1 and VL2a glomeruli (outlined), but lacks expression in the VA5 and DC3 glomeruli that are consistently labeled in the knock-in. Additionally, it drives GFP expression in Orco+ glomeruli not seen in the knock-in (outlined). (D) Ir25a-Gal4 labels some Orco+ glomeruli (outline includes DA2, DA3, DA4m, and DA4l), but is lacking expression in many glomeruli labeled by the knock-in. (E) The UAS-GFP control line has weak leaky expression in the subesophageal zone (SEZ) but no expression in the antennal lobe (AL). (A) shows single slice to visualize weakly labeled glomeruli; (B–E) are maximum intensity projections of z-stacks. Scale bar = 50 µm. See also Figure 3—source data 1.
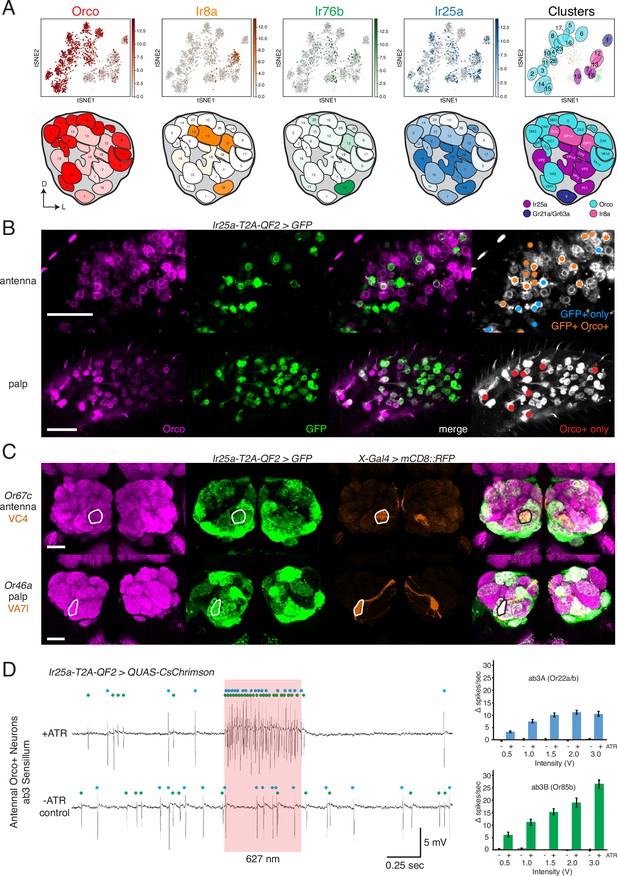
Confirmation of co-receptor co-expression.
(A) snRNAseq of adult fly antennae (McLaughlin et al., 2021) confirms expanded expression of olfactory co-receptors. Top: tSNE plots show expression of each co-receptor in 20 decoded olfactory sensory neuron (OSN) clusters. Bottom: clusters were mapped to 24 glomeruli. Opacity of fill in each glomerulus indicates the proportion of cells in that cluster expressing the given co-receptor, normalized to total expression for that co-receptor gene (see Figure 4—source data 1). Right column: clusters color-coded according to original chemoreceptor gene family. Compass: D = dorsal; L = lateral. (B) Anti-Orco antibody staining in antennal cryosections (top) and whole-mount palps (bottom) confirms co-expression of Orco and Ir25a in the periphery (genotype: Ir25a-T2A-QF2>GFP). Right panels show cells pseudo-colored gray with specific single- or double-labeled cells indicated by colored cell markers (GFP+ only in blue, GFP+Orco+ in orange, Orco+ only in red). (C) Co-labeling experiments with various transgenic Gal4 lines driving mCD8::RFP (orange) and the Ir25a-T2A-QF2 knock-in driving GFP (green). Ir25a-T2A-QF2 labels glomeruli innervated by both antennal (top) and palpal (bottom) OSNs. (D) Verification of Ir25a expression in antennal ab3 sensilla using optogenetics. Single sensillum recordings (SSR) from ab3 Orco+ neurons in Ir25a-T2A-QF2>QUAS-CsChrimson flies. Representative traces from ab3 using 1.5 V of 627 nm LED light (red box) to activate CsChrimson. Bottom trace is control animal, which has the same genotype as the experimental animal but was not fed the required all-trans retinal cofactor (-ATR). Spikes from the ab3A and ab3B neurons are indicated by blue and green dots, respectively. Right: quantification of neuronal activity in response to light at various LED intensities (N = 7–12). These optogenetic experiments support Ir25a expression in both ab3A neurons (Or22a/b, top; corresponding to DM2 glomerulus) and ab3B neurons (Or85b, bottom; corresponding to VM5d glomerulus). Scale bars = 25 µm. See also Figure 4—figure supplement 1, Table 3, Figure 4—source data 1, Figure 4—source data 2, and Figure 4—source data 3.
-
Figure 4—source data 1
snRNAseq co-receptor expression in adult olfactory sensory neurons (OSNs).
- https://cdn.elifesciences.org/articles/72599/elife-72599-fig4-data1-v2.xlsx
-
Figure 4—source data 2
Individual glomerular analyses.
- https://cdn.elifesciences.org/articles/72599/elife-72599-fig4-data2-v2.xlsx
-
Figure 4—source data 3
Optogenetic validation of Ir25a expression.
- https://cdn.elifesciences.org/articles/72599/elife-72599-fig4-data3-v2.xlsx
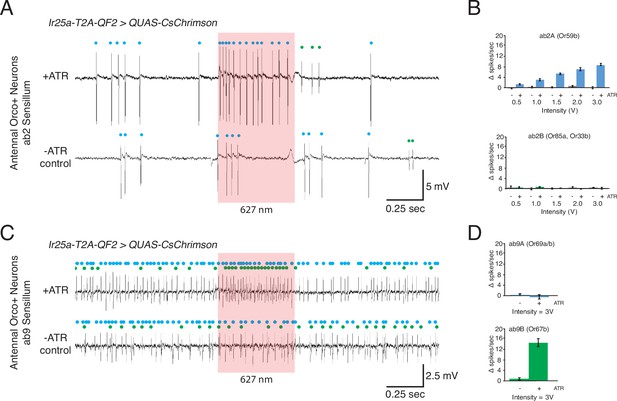
Optogenetic experiments to examine Ir25a expression in Orco+ neurons.
(A–D) Confirmation of Ir25a expression in ab2 and ab9 sensilla using optogenetic stimulation. CsChrimson expression is driven by Ir25a-T2A-QF2, and recordings are performed in neurons previously determined to express only Orco. In all optogenetic experiments, control animals have the same genotypes as the corresponding experimental animals but have not been fed all-trans retinal. (A) Representative single sensillum recording (SSR) traces from ab2 using 1.5 V of a 627 nm LED light (red box) to activate CsChrimson. Bottom trace is control animal, which was not fed the necessary cofactor all-trans retinal (-ATR). ab2 has two neurons: blue dots indicate A neuron spikes, while green dots indicate B neuron spikes (genotype: Ir25a-T2A-QF2; QUAS-CsChrimson). (B) Quantification of neuronal activity in response to light stimulation at various intensities (N = 7–12). Optogenetic experiments confirm Ir25a expression in ab2A (expressing Or59b, top; DM4 glomerulus) but not ab2B (expressing Or85a and Or33b, bottom; DM5 glomerulus). (C) Combination of optogenetics and fluorescent-guided SSR (Lin and Potter, 2015) to examine Ir25a expression in ab9 sensilla (genotype: Ir25a-T2A-QF2; QUAS-CsChrimson/Or67b-Gal4, 15XUAS-IVS-mcd8::GFP). Representative traces from ab9 in response to 3 V of 627 nm LED light (red box). As in (A), -ATR indicates the inactive CsChrimson control; blue dots indicate A neuron spikes, green dots indicate B neuron. (D) Quantification of activity in response to light stimulation verified that Ir25a is expressed in ab9B (Or67b, bottom; VA3 glomerulus) but not in ab9A neurons (Or69aA/aB, top; D glomerulus). N = 5.
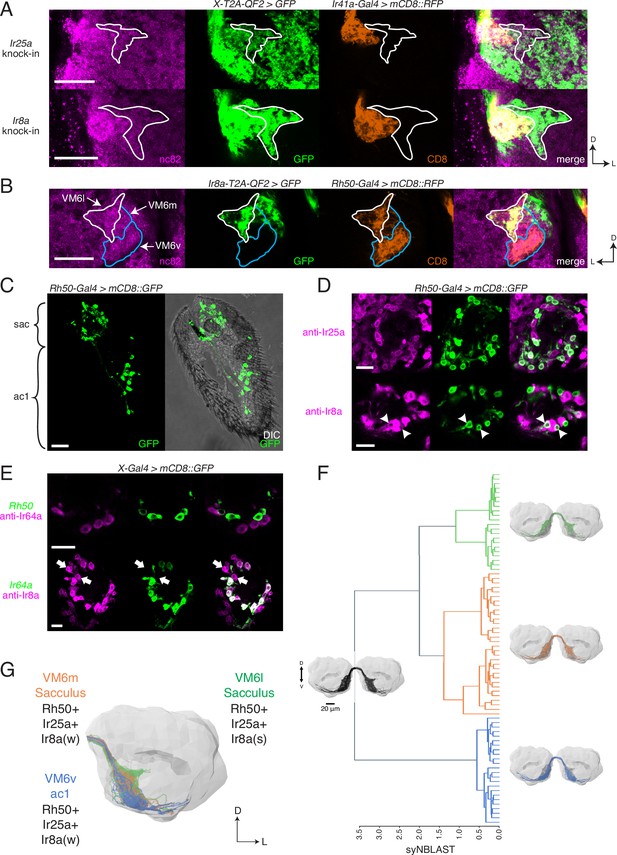
Identification of new olfactory sensory neuron (OSN) classes.
(A) Co-labeling experiments with Ir41a-Gal4 show that both Ir25a-T2A-QF2 and Ir8a-T2A-QF2 label the VC5 glomerulus (orange), and also a previously unidentified antennal lobe (AL) region (outline). (B) The new innervation pattern corresponds to the ‘horn’ (white outline) of the VM6 glomerulus labeled by Rh50+ neurons (orange). One portion of VM6 is strongly Ir8a+ (VM6l), while two other portions show little to no Ir8a expression (VM6m and VM6v, blue outlines). (C) Rh50-Gal4>GFP labels neurons in the sacculus (sac) and antennal coeloconic ac1 sensilla. (D) In the sacculus, all Rh50+ neurons appear to be Ir25a+ (top), and a subset are Ir8a+ (bottom, arrowheads). (E) Top: Rh50+ neurons in the sacculus do not overlap with Ir64a+ neurons. Bottom: there are two distinct populations of Ir8a+ neurons in the sacculus – those that are Ir64a+ and those that are Ir64a- (arrows). The latter likely correspond to Rh50+ neurons. (F) EM reconstructions of VM6 OSNs in a full brain volume (Dorkenwald et al., 2020) reveal three distinct subpopulations. (G) Model of OSN innervation of the VM6 region. VM6 can be subdivided into three OSN populations based on anatomical location in the periphery and chemoreceptor expression: VM6v (blue) OSNs originate in ac1, strongly (s) express Rh50 and Ir25a, and weakly (w) or infrequently express Ir8a; VM6m (orange) neurons originate in the sacculus and have a similar chemoreceptor expression profile to VM6v; VM6l (green) OSNs originate in the sacculus but strongly express Ir8a in addition to Rh50 and Ir25a. Compass: D = dorsal, L = lateral. Scale bars: 20 µm in (A–C) and (F), 10 µm in (D, E). N = 9–11 for (C–E). See also Figure 5—figure supplement 1 and Tables 3 and 4.
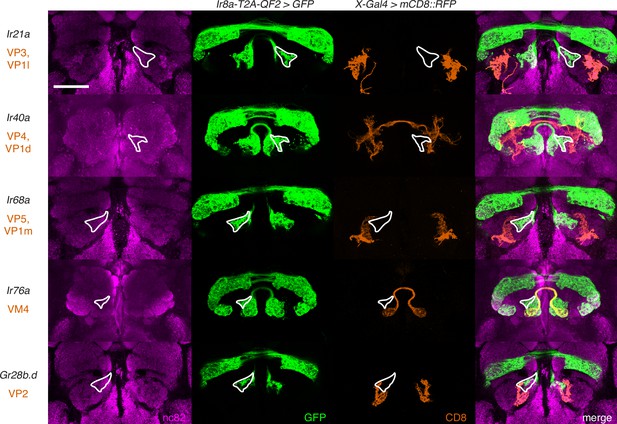
The new glomerular region labeled by the Ir8a knock-in does not correspond to previously identified posterior glomeruli.
Co-labeling experiments with Ir8a-T2A-QF2>GFP (green) and various Gal4 lines driving mCD8::RFP (orange) confirm that the outlined region does not correspond to any of the VP glomeruli nor to VM4. Scale bar: 50 µm.
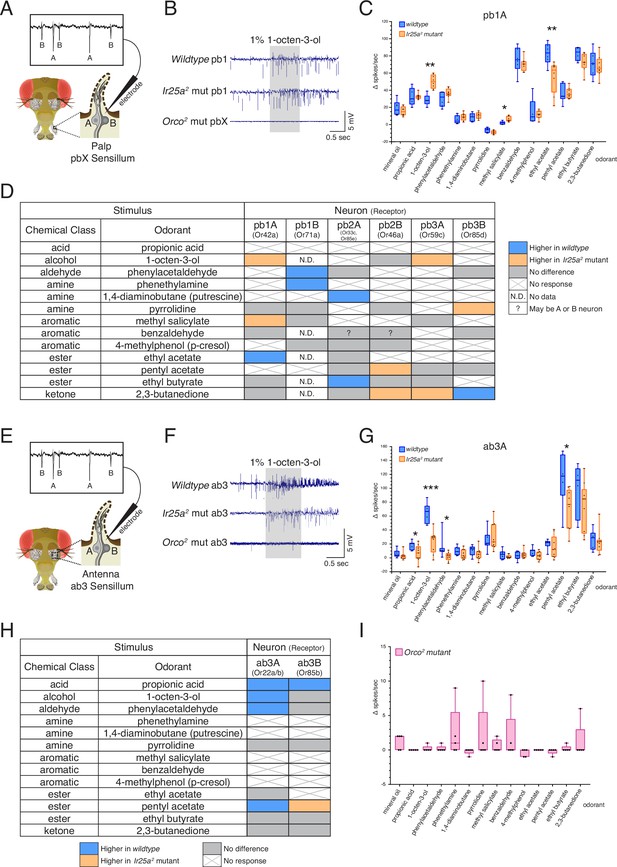
Co-receptor contributions to olfactory neuron physiology.
(A–I) Single sensillum recording (SSR) experiments were performed in three genetic backgrounds: wildtype, Ir25a2 mutant, and Orco2 mutant flies. A panel of 13 odorants was tested. In all box plots, *p<0.05, **p<0.01, and ***p<0.001. (A) Cartoon of a fly head, zooming in on a single sensillum in the palp. Each palpal sensillum (pbX) contains two neurons, A and B. An electrode is inserted into the sensillum, and neuronal activity is recorded in response to odorants. Activity of the A and B neurons can be distinguished based on their spike amplitudes (top). (B) Representative traces from recordings in palp basiconic pb1 sensilla in the three genotypes in response to 1% 1-octen-3-ol. Sensilla were identified based on responses to reference odorants (de Bruyne et al., 1999; see Materials and methods). The Orco2 mutant did not exhibit odor-evoked activity nor spontaneous activity, making it difficult to determine the identity of the recorded sensillum. Orco2 mutant sensilla are thus denoted pbX. (C) Quantification of responses to the panel of odorants in wildtype (blue; N = 5–9 flies) and Ir25a2 mutant (orange; N = 6–10 flies) pb1A neurons. Responses were higher in the Ir25a2 mutant than in the wildtype for 1-octen-3-ol and methyl salicylate, and lower in the Ir25a2 mutant for ethyl acetate. Mann–Whitney U tests indicated these differences were statistically significant: 1-octen-3-ol: MdnIr25amut = 50, Mdnwildtype = 28, U(NIr25amut = 8, Nwildtype = 5) = 0, p=0.0016; methyl salicylate: MdnIr25amut = 5, Mdnwildtype = 2, U(NIr25amut = 7, Nwildtype = 5) = 3, p=0.0177; ethyl acetate: MdnIr25amut = 63.5, Mdnwildtype = 83.5, U(NIr25amut = 8, Nwildtype = 6) = 4, p=0.008. (D) Summary of differences in responses across all six neuron classes in the palps between wildtype and Ir25a2 mutant flies. Comparisons were made using Mann–Whitney U tests. Orange indicates higher response in Ir25a2 mutant, blue indicates higher response in wildtype. Gray is no difference between genotypes, X indicates no response to the given stimulus, and N.D. is no data (strong A neuron response obscured B neuron spikes preventing quantification). In the wildtype, for one sensillum-odorant combination (pb2 and benzaldehyde), it could not be distinguished if responses arose from the A or B neuron or both (indicated by a question mark). (E) Fly head cartoon, zooming in on a single sensillum in the antenna. We recorded from antennal ab3 sensilla, each of which contains two neurons, A and B. As in the palps, responses from these neurons can be distinguished based upon their spike amplitude (top). (F) Representative traces from recordings in antennal basiconic ab3 sensilla in the three genotypes in response to 1% 1-octen-3-ol. In Orco2 mutant ab3 sensilla spontaneous activity was observed, but there was no significant odor-evoked activity. Wildtype N = 7 sensilla from five flies; Ir25a2 mutant N = 10 sensilla from five flies. (G) Quantification of responses in wildtype (blue; N = 7) and Ir25a2 mutant (orange; N = 9) ab3A neurons. Responses were significantly higher in wildtype compared to Ir25a2 mutant ab3A neurons for four odorants (Mann–Whitney U results in parentheses; all Nwildtype = 7 and NIr25amut = 9): propionic acid (Mdnwildtype = 21, MdnIr25amut = 7, U = 12.5, p=0.0441); 1-octen-3-ol (Mdnwildtype = 67, MdnIr25amut = 29, U = 1.5, p=0.0004); phenylacetaldehyde (Mdnwildtype = 10, MdnIr25amut = 3, U = 9, p=0.015); and pentyl acetate (Mdnwildtype = 118, MdnIr25amut = 77, U = 9, p=0.0164). Difference between wildtype and Ir25a2 mutant to phenylacetaldehyde is significant even with the large wildtype outlier removed (p=0.0336). (H) Summary of differences in responses in the two neuron classes in ab3 between wildtype and Ir25a2 mutant flies. Comparisons were made using Mann–Whitney U tests. Orange indicates higher response in Ir25a2 mutant, blue indicates higher response in wildtype, gray is no difference between genotypes, and X is no response to the given stimulus. One Ir25a2 mutant fly was excluded from analyses as it had high responses to the mineral oil control (40–53 Δ spikes/s), not seen in any other animal of any genotype. (I) Weak responses in Orco2 mutant flies to certain stimuli (≤10 Δ spikes/s) were occasionally detected. While there were some statistically significant differences from mineral oil control (pentyl acetate p=0.0109, propionic acid p=0.0434, ethyl acetate p=0.0434, 1,4-diaminobutane p=0.0109, p-cresol p=0.0021), these were not deemed biologically significant due to very small Δ spike values relative to zero. For more details, see Materials and methods. N = 5 flies. See also Figure 6—figure supplements 1–3 and Figure 6—source data 1.
-
Figure 6—source data 1
Single sensillum recording (SSR) of Ir25a mutant, Orco mutant, and wildtype flies.
- https://cdn.elifesciences.org/articles/72599/elife-72599-fig6-data1-v2.xlsx
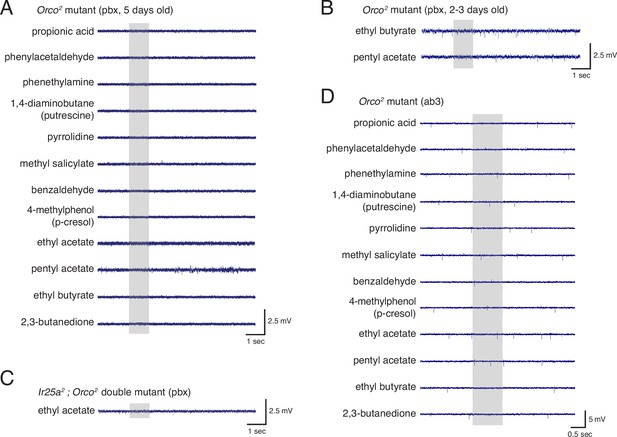
Electrophysiological experiments to examine Ir25a function in Orco+ neurons.
(A) Single sensillum recordings (SSR) in Orco2 mutant palpal sensilla show neither baseline nor odor-evoked activity in response to a panel of odorants in 5-day-old flies. N = 20 sensilla, four animals (three males, one female). (B) Young Orco2 mutant palpal neurons also do not have spontaneous or odor-evoked activity. (C) Ir25a2; Orco2 double mutant palpal sensilla show neither baseline nor odor-evoked activity. N = 42 sensilla from five animals (three males, two females). (D) Antennal ab3 sensilla do occasionally show baseline activity in the Orco2 mutant, but generally do not show odor-evoked activity (N = 5 flies; see also Figure 6). Gray box indicates the time of stimulus delivery. See also Figure 6—source data 1.
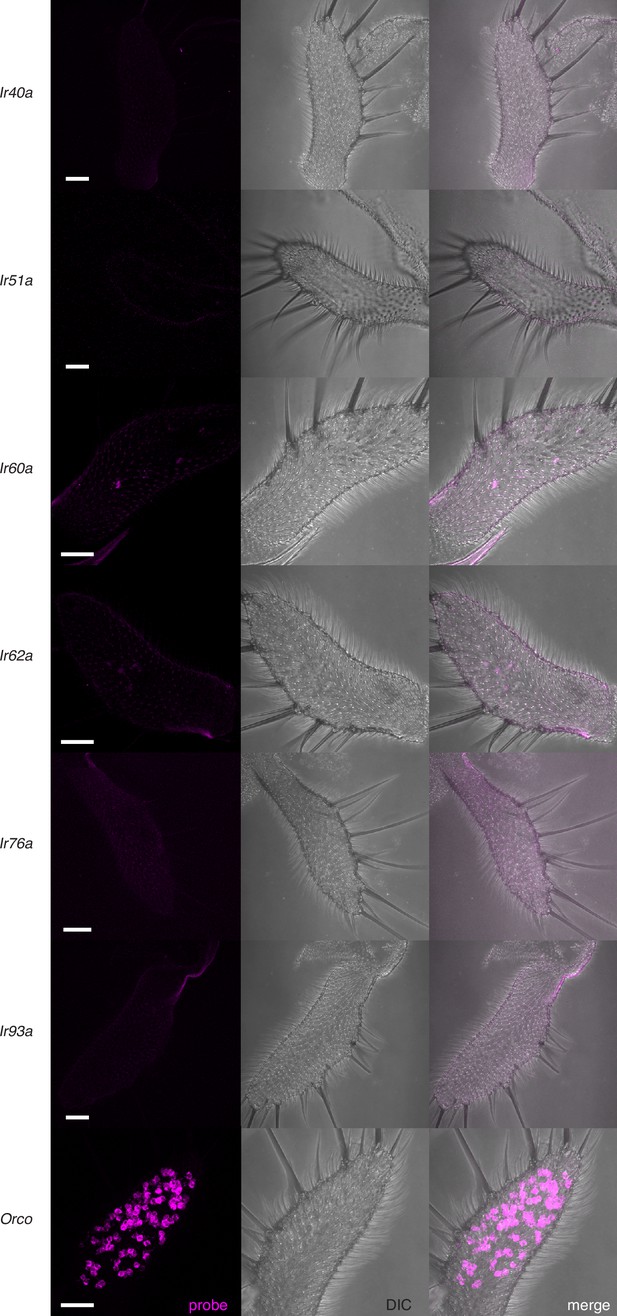
No IrX expression of the top candidates in the maxillary palps.
The recent fly cell atlas (Li et al., 2021) was used to identify potential IrX tuning receptors in the palps. In situ experiments did not reveal strong expression of any of these target genes. Orco (bottom) was used as a positive control. See also Appendix 1—key resources table. Scale bars = 25 µm.
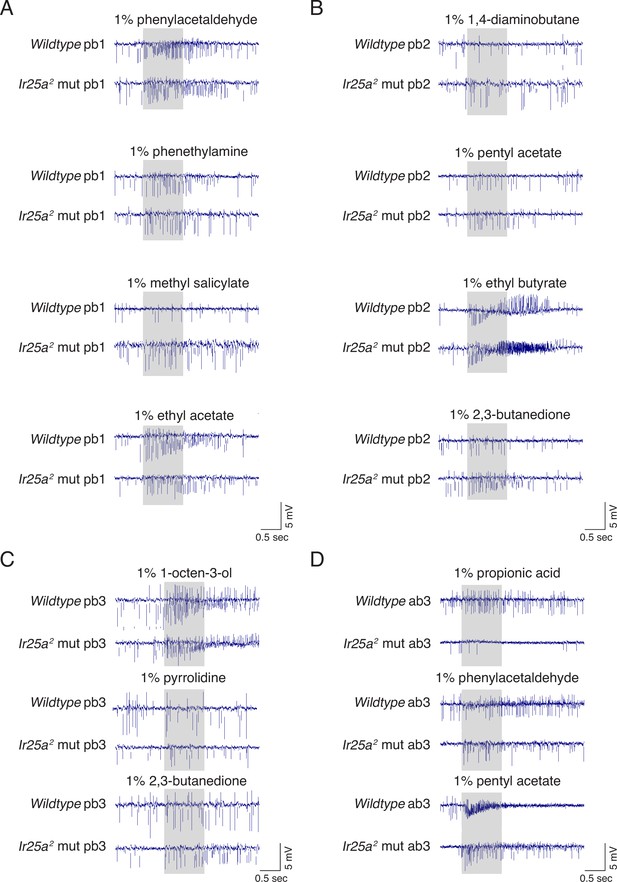
Example traces for odorants eliciting differences between wildtype and Ir25a mutant sensilla.
(A–C) Palpal single sensillum recordings (SSR) in wildtype and Ir25a2 mutant sensilla. (D) Ab3 SSR in wildtype and Ir25a2 mutant sensilla. The data from these and other recordings are represented by the blue (responses higher in wildtype) and orange (responses higher in Ir25a2 mutant) boxes in Figure 6D (pb sensilla) and Figure 6H (ab3). (A) pb1B neurons have higher responses to phenylacetaldehyde and phenethylamine in wildtype compared to Ir25a mutant sensilla (top two rows), while pb1A mutant neurons have higher responses to methyl salicylate (third row), and lower responses to ethyl acetate (fourth row). (B) pb2A wildtype neurons have higher responses to 1,4-diaminobutane (first row) and ethyl butyrate (third row) compared to Ir25a mutants. pb2B neurons have higher responses to pentyl acetate (second row) and 2,3-butanedione (fourth row) in the Ir25a mutant. (C) pb3A Ir25a mutant neurons show stronger responses to 1-octen-3-ol (top) and 2,3-butanedione (bottom), while pb3B mutant neurons show weaker responses to 2,3-butanedione (middle) compared to wildtype. (D) In ab3 sensilla, both the A and B neurons respond more strongly to propionic acid in the wildtype compared to the Ir25a mutant (top); the A neuron also has a higher response to phenylacetaldehyde in the wildtype compared to the Ir25a mutant (middle). The response to pentyl acetate (bottom) is mixed: the A neuron responds more strongly in the wildtype, but the B neuron responds more strongly in the Ir25a mutant.
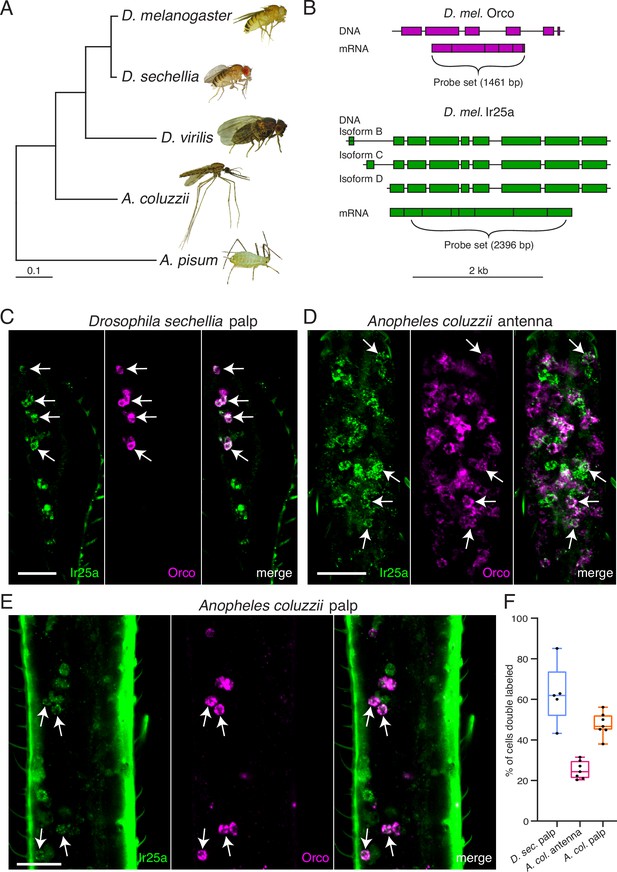
Orco and Ir25a are co-expressed in Drosophila sechellia and Anopheles coluzzii olfactory organs.
(A) Phylogenetic tree based on the Orco sequences from the five insects shown (D. = Drosophila, A. coluzzii = Anopheles coluzzii, A. pisum = Acyrthosiphon pisum). Evolutionary history was inferred using the Maximum Likelihood method and Tamura–Nei model (Tamura and Nei, 1993). Pea aphid image A was reproduced from PLoS Biology Issue Image (2010). (B) The Drosophila melanogaster Orco in situ probe set, which covers the entire Orco coding sequence (top, magenta), was used to examine Orco expression in the maxillary palps of other Drosophila fly species. We designed a new probe set covering the most conserved portion of D. mel. Ir25a (bottom) as determined by analyzing the Ir25a sequences from multiple fly species and comparing them to the various Drosophila melanogaster Ir25a isoforms (three of which are illustrated in green). (C) Many olfactory sensory neurons (OSNs) in the Drosophila sechellia maxillary palps co-express both Orco and Ir25a, as revealed by in situ experiments (four example cells indicated with arrows). N = 5. (D) In situs in Anopheles coluzzii antennae reveal a small proportion of cells expressing both co-receptors (arrows). N = 7. (E) In situs in Anopheles coluzzii maxillary palps show many cells express both Orco and Ir25a (four examples indicated with arrows). N = 7. (F) Summary of co-expression analyses in (C–E). For each olfactory organ examined, we divided the number of Orco+ Ir25a+ double-labeled cells by the total number of cells labeled by either probe. We found that 63% of D. sec. palpal OSNs express both Orco and Ir25a (blue), 25% of A. col. antennal OSNs express both co-receptors (pink), and 47% of A. col. palpal OSNs are double labeled (orange). (C–E) are maximum intensity projections of partial z-stacks. See also Table 5, and Appendix 1—key resources table.
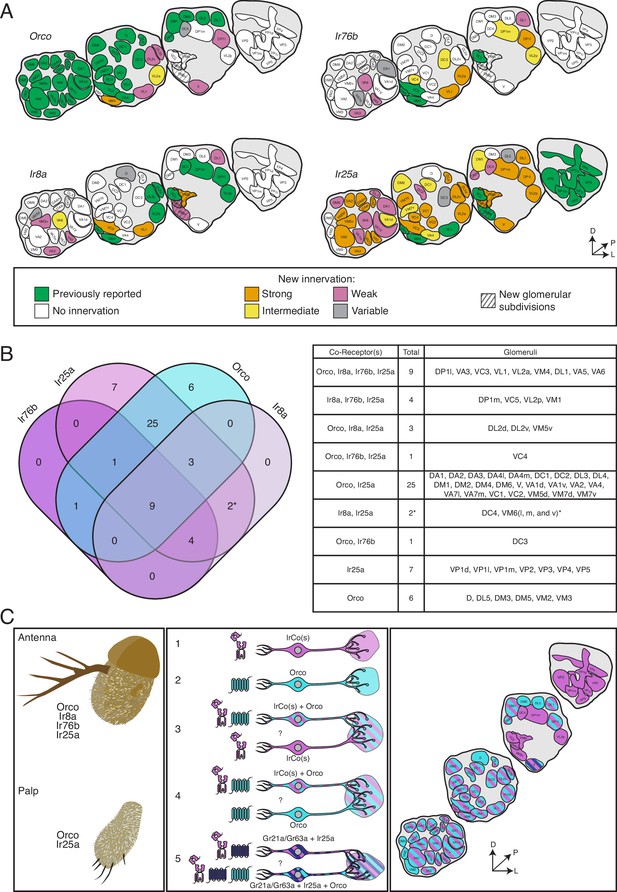
The co-receptor co-expression map of olfaction in Drosophila melanogaster.
(A) Summary of antennal lobe (AL) expression for all co-receptor knock-in lines (from all brains examined in Figures 3—5; Orco N = 8, Ir8a N = 15, Ir76b N = 11, Ir25a N = 15). The previously reported innervation pattern for each co-receptor is shown in green; new innervation reported here is color-coded according to strength of glomerular labeling, from strong (orange), to intermediate (yellow), to weak (pink). Glomeruli labeled in <50% of brains examined for a given knock-in line are designated variable (gray); glomeruli not labeled are white. The novel VM6 glomerular subdivisions reported here are indicated by gray stripes. (B) Overlap of chemosensory modalities in the AL. In the Venn diagram (left), IR co-receptors are color-coded in shades of purple, while Orco is in teal, as in Figure 1. Numbers indicate how many glomeruli are found in the given intersection of co-receptors out of 58 total glomeruli. Variably labeled glomeruli were excluded from these analyses. The table lists the names of the glomeruli in each section of the Venn diagram. The new glomerular subdivisions are indicated with an asterisk. (C) New view of olfaction in Drosophila. Left: in the periphery, all four co-receptors are expressed in the antenna (top), while palpal neurons express Orco and Ir25a (bottom). Middle: many different classes of olfactory sensory neurons (OSNs) express various combinations of chemosensory receptors and co-receptors. While some neurons express only IrCos (purple, #1) or Orco (teal, #2), many neurons co-express these chemoreceptors (indicated with striped fill, #3 and 4). Within the latter group, there may be OSN populations in which IRs are the dominant receptors, and OR expression is sparse (#3), and other populations where ORs are the primary receptors and IR expression is infrequent (#4). GR+ neurons (dark blue) also express Ir25a (#5, dark blue and purple striped fill), and some of these neurons additionally express Orco (#5, dark blue, purple, and teal striped fill). Question marks indicate potential instances of co-convergence of different subtypes of OSNs onto the same glomeruli. Right: a comprehensive map of the antennal lobe shows that most glomeruli are innervated by OSNs that co-express multiple chemoreceptors. Compass in (A) and (C): D = dorsal, L = lateral, P = posterior. See also Table 3 and Figure 3—source data 1 and Figure 3—source data 2.
Tables
Summary of HACK knock-in efficiency (related to Figure 2).
Gene | Approach | mCherry+ | mCherry- | Total | Efficiency (%) | Founders producing knock-in (#/total) | Knock-ins sampled | False positives | Confirmed | Correct (%) |
---|---|---|---|---|---|---|---|---|---|---|
Orco | Direct injection | 180 | 365 | 545 | 33 | 43% (3/7) | 30 | 0 | 30 | 100 |
Ir8a | Direct injection | 53 | 609 | 662 | 8 | 20% (4/20) | 5 | 0 | 5 | 100 |
Ir76b | Direct injection | 79 | 184 | 263 | 30 | 100% (2/2) | 10 | 0 | 10 | 100 |
Ir25a | Direct injection | 82 | 268 | 350 | 23 | 40% (2/5) | 6 | 0 | 6 | 100 |
Orco | Cross | 37 | 96 | 133 | 28 | 100% (3/3) | 2 | 1 | 1 | 50 |
Ir25a | Cross | 30 | 95 | 125 | 24 | 100% (2/2) | 30 | 5 | 25 | 83 |
-
Table 1—source data 1
HACK knock-in screen.
- https://cdn.elifesciences.org/articles/72599/elife-72599-table1-data1-v2.xlsx
Validation of T2A-QF2 knock-in expression in the antennae and maxillary palps (related to Figure 2).
To verify that the knock-in lines recapitulate the endogenous expression patterns of the target genes, antennae or maxillary palps of flies containing the knock-ins driving GFP expression were co-stained with the corresponding antibody (Ab) (anti-Orco, anti-Ir8a, or anti-Ir25a). The overlap of Ab+ and GFP+ cells was examined, and a high correspondence between antibody staining and knock-in driven GFP was found. WM: whole-mount; cryo: cryosection. See also Figure 2—figure supplement 3.
Knock-in | Sample | Antibody (Ab) | Ab+ cells | GFP+ cells | Double-labeled cells | Total cells |
---|---|---|---|---|---|---|
Orco | Palp 1 (WM) | Anti-Orco | 125 | 127 | 125 | 127 |
Orco | Palp 2 (WM) | Anti-Orco | 112 | 111 | 108 | 115 |
Orco | Palp 6 (WM) | Anti-Orco | 125 | 126 | 123 | 128 |
Total across samples: | 362 | 364 | 356 | 370 | ||
Proportion of Ab+ cells that are GFP+: | Proportion of GFP+ cells that are Ab+: | Proportion of all cells that are double labeled: | ||||
0.98 | 0.98 | 0.96 | ||||
Ir8a | Antenna 1 (cryo) | Anti-Ir8a | 20 | 21 | 20 | 21 |
Ir8a | Antenna 2 (cryo) | Anti-Ir8a | 24 | 24 | 24 | 24 |
Ir8a | Antenna 6 (cryo) | Anti-Ir8a | 40 | 43 | 40 | 43 |
Ir8a | Antenna 7 (cryo) | Anti-Ir8a | 12 | 13 | 12 | 13 |
Ir8a | Antenna 8 (cryo) | Anti-Ir8a | 16 | 16 | 16 | 16 |
Ir8a | Antenna 9 (cryo) | Anti-Ir8a | 42 | 42 | 41 | 43 |
Ir8a | Antenna 10 (cryo) | Anti-Ir8a | 41 | 40 | 40 | 41 |
Total across samples: | 195 | 199 | 193 | 201 | ||
Proportion of Ab+ cells that are GFP+: | Proportion of GFP+ cells that are Ab+: | Proportion of all cells that are double labeled: | ||||
0.99 | 0.97 | 0.96 | ||||
Ir25a | Palp 1 (WM) | Anti-Ir25a | 107 | 105 | 104 | 108 |
Ir25a | Palp 2 (WM) | Anti-Ir25a | 86 | 85 | 85 | 86 |
Ir25a | Palp 3 (WM) | Anti-Ir25a | 111 | 111 | 110 | 112 |
Ir25a | Palp 4 (WM) | Anti-Ir25a | 94 | 94 | 94 | 94 |
Ir25a | Palp 5 (WM) | Anti-Ir25a | 83 | 83 | 81 | 85 |
Total across samples: | 481 | 478 | 474 | 485 | ||
Proportion of Ab+ cells that are GFP+: | Proportion of GFP+ cells that are Ab+: | Proportion of all cells that are double labeled: | ||||
0.99 | 0.99 | 0.98 |
Summary of expression patterns for all knock-in lines (related to Figures 3—5).
Summarized here are all of the olfactory sensory neuron (OSN) classes innervating the 58 antennal lobe glomeruli†; their corresponding sensilla and tuning receptors; the previously reported (original) co-receptors they express; and whether or not each of the co-receptor knock-in lines labels those glomeruli. Variable indicates that the glomerulus was labeled in <50% of brains examined in the given knock-in line. Sensilla or glomeruli that have been renamed or reclassified have their former nomenclature listed in parentheses. Question marks indicate expression that has been reported but not functionally validated. * See also Figure 3—source data 1 and Figure 3—source data 2.
-
*VM6l was initially named VC6 in version 1 of our pre-print (Task et al., 2020) but was reclassified using additional data from EM reconstructions in the antennal lobe (AL) and immunohistochemical experiments in the periphery (see Figure 5).
-
†The VM6 subdivisions (VM6v, VM6m, VM6l) are separated in this table for clarity but counted together as one glomerulus in accordance with Schlegel et al., 2021.
Co-expression of Rh50 and Ir8a in the sacculus (related to Figure 5).
Antennal cryosections of Rh50-Gal4>GFP flies were stained with an anti-Ir8a antibody, and the overlap of Ir8a+ and GFP+ cells was quantified in the sacculus. 22% of Ir8a+ cells expressed Rh50, 35% of Rh50+ cells expressed Ir8a, and 16% of all cells were double labeled. N = 11.
Genotype | Sample | Ir8a+ cells | GFP+ cells | Double-labeled cells | Total cells |
---|---|---|---|---|---|
Rh50-Gal4>GFP | 20210226 a1 | 18 | 9 | 2 | 25 |
Rh50-Gal4>GFP | 20210226 a2 | 22 | 15 | 4 | 33 |
Rh50-Gal4>GFP | 20210226 a3 | 41 | 22 | 7 | 56 |
Rh50-Gal4>GFP | 20210226 a4 | 41 | 14 | 5 | 50 |
Rh50-Gal4>GFP | 20210129 a1 | 26 | 20 | 9 | 37 |
Rh50-Gal4>GFP | 20210129 a2 | 32 | 24 | 7 | 49 |
Rh50-Gal4>GFP | 20210129 a3 | 29 | 19 | 7 | 41 |
Rh50-Gal4>GFP | 20210216 a1 | 26 | 21 | 8 | 39 |
Rh50-Gal4>GFP | 20210216 a2 | 30 | 18 | 7 | 41 |
Rh50-Gal4>GFP | 20210216 a3 | 34 | 23 | 8 | 49 |
Rh50-Gal4>GFP | 20210216 a4 | 34 | 23 | 9 | 48 |
Total across samples: | 333 | 208 | 73 | 468 | |
Proportion of Ir8a+ cells that are GFP+: | Proportion of GFP+ cells that are Ir8a+: | Proportion of all cells that are double labeled: | |||
0.22 | 0.35 | 0.16 |
Co-expression of Orco and Ir25a in non-melanogaster insect olfactory organs (related to Figure 7).
Whole-mount palps from Drosophila sechellia flies, and whole-mount antennae and palps from Anopheles coluzzii mosquitoes, were examined using fluorescence in situ hybridization with probe sets against Orco and Ir25a. Co-expression between Orco and Ir25a co-receptors was observed in both insects, with D. sec. palps having the highest degree of co-expression (63% of cells double labeled) and A. col. antennae having the lowest (25% of cells double labeled). N = 5 for D. sec. and 7 for A. col.
Species | Sample | Orco+ cells | Ir25a+ cells | Double-labeled cells | Total cells |
---|---|---|---|---|---|
Drosophila sechellia | Palp 1 | 70 | 44 | 44 | 70 |
Drosophila sechellia | Palp 2 | 64 | 48 | 42 | 70 |
Drosophila sechellia | Palp 3 | 63 | 39 | 39 | 63 |
Drosophila sechellia | Palp 4 | 78 | 38 | 35 | 81 |
Drosophila sechellia | Palp 5 | 86 | 75 | 74 | 87 |
Total across samples: | 361 | 244 | 234 | 371 | |
Proportion of Orco+ cells that are Ir25a+: | Proportion of Ir25a+ cells that are Orco+: | Proportion of all cells that are double labeled: | |||
0.65 | 0.96 | 0.63 | |||
Anopheles coluzzii | Antenna 1 | 52 | 17 | 12 | 57 |
Anopheles coluzzii | Antenna 2 | 47 | 24 | 17 | 54 |
Anopheles coluzzii | Antenna 3 | 57 | 20 | 13 | 64 |
Anopheles coluzzii | Antenna 4 | 50 | 27 | 14 | 63 |
Anopheles coluzzii | Antenna 5 | 62 | 30 | 18 | 74 |
Anopheles coluzzii | Antenna 6 | 53 | 21 | 17 | 57 |
Anopheles coluzzii | Antenna 7 | 49 | 26 | 16 | 59 |
Total across samples: | 370 | 165 | 107 | 428 | |
Proportion of Orco+ cells that are Ir25a+: | Proportion of Ir25a+ cells that are Orco+: | Proportion of all cells that are double labeled: | |||
0.29 | 0.65 | 0.25 | |||
Anopheles coluzzii | Palp 1 | 34 | 30 | 23 | 41 |
Anopheles coluzzii | Palp 2 | 30 | 36 | 22 | 44 |
Anopheles coluzzii | Palp 3 | 26 | 35 | 19 | 42 |
Anopheles coluzzii | Palp 4 | 35 | 34 | 19 | 50 |
Anopheles coluzzii | Palp 5 | 32 | 39 | 22 | 49 |
Anopheles coluzzii | Palp 6 | 31 | 35 | 21 | 45 |
Anopheles coluzzii | Palp 7 | 30 | 37 | 23 | 44 |
Total across samples: | 218 | 246 | 149 | 315 | |
Proportion of Orco+ cells that are Ir25a+: | Proportion of Ir25a+ cells that are Orco+: | Proportion of all cells that are double labeled: | |||
0.68 | 0.61 | 0.47 |
Reagent type (species) or resource | Designation | Source or reference | Identifiers | Additional information |
---|---|---|---|---|
Antibody | Anti-nc82 (mouse monoclonal) | DSHB | Cat# nc82; RRID:AB_2314866 | IHC (1:25) |
Antibody | Anti-cd8 (rat monoclonal) | Thermo Fisher Scientific | Cat# 14-0081-82; RRID:AB_467087 | IHC (1:100 or 1:200) |
Antibody | Anti-elav (rat monoclonal) | DSHB | Cat# Rat-Elav-7E8A10; RRID:AB_528218 | IHC (1:100) |
Antibody | Anti-Orco (rabbit polyclonal) | Gift from Leslie Vosshall Larsson et al., 2004 | IHC (1:100) | |
Antibody | Anti-Ir25a (rabbit polyclonal) | Gift from Benton et al., 2009 | RRID:AB_2567027 | IHC (1:100) |
Antibody | Anti-Ir8a (guinea pig polyclonal) | Gift from Richard Benton Abuin et al., 2011 | RRID:AB_2566833 | IHC (1:100 for whole-mount, 1:1000 for cryosections) |
Antibody | Anti-Ir64a (rabbit polyclonal) | Gift from Greg Suh Ai et al., 2010 | RRID:AB_2566854 | IHC (1:100) |
Antibody | Anti-guinea pig Alexa 568 (goat polyclonal) | Thermo Fisher Scientific | Cat# A11075; RRID:AB_141954 | IHC (1:500) |
Antibody | Anti-rabbit Cy3 conjugated AffiniPure 568 (goat polyclonal) | Jackson ImmunoResearch | Cat# 111-165-144; RRID:AB_2338006 | IHC (1:500) |
Antibody | Anti-mouse Cy3 (goat polyclonal) | Jackson ImmunoResearch | Cat# 115-165-166; RRID:AB_2338692 | IHC (1:200) |
Antibody | Anti-mouse Alexa 647 (goat polyclonal) | Jackson ImmunoResearch | Cat# 115-605-166; RRID:AB_2338914 | IHC (1:200) |
Antibody | Anti-guinea pig Cy3 (donkey polyclonal) | Jackson ImmunoResearch | Cat# 706-165-148; RRID:AB_2340460 | IHC (1:200) |
Antibody | Anti-rat Cy3 (goat polyclonal) | Jackson ImmunoResearch | Cat# 112-165-167; RRID:AB_2338251 | IHC (1:200) |
Antibody | Anti-rat Alexa 647 (goat polyclonal) | Jackson ImmunoResearch | Cat# 112-605-167; RRID:AB_2338404 | IHC (1:200) |
Antibody | Anti-rabbit Cy3 (goat polyclonal) | Jackson ImmunoResearch | Cat# 111-165-144; RRID:AB_2338006 | IHC (1:200) |
Antibody | Anti-rabbit Alexa 647 (goat polyclonal) | Jackson ImmunoResearch | Cat# 111-605-144; RRID:AB_2338078 | IHC (1:200) |
Recombinant DNA reagent | pHACK-QF2 (plasmid) | Addgene Lin and Potter, 2016a | Plasmid# 80274; RRID:Addgene_80274 | QF2 HACK backbone Contains QF2-hsp70, but no gRNAs |
Recombinant DNA reagent | p10XQUAS-CsChrimson-SV40 (plasmid) | This paper | Plasmid# 163629; RRID:Addgene_163629 | For red-shifted optogenetic activation of neurons under control of the Q-system; see ’Fly stocks’ |
Recombinant DNA reagent | pHACK-QF2Orco (plasmid) | This paper | HACK construct targeting the Orco gene; see ‘Plasmid construction’ | |
Recombinant DNA reagent | pHACK-QF2Ir8a (plasmid) | This paper | HACK construct targeting the Ir8a gene; see ‘Plasmid construction’ | |
Recombinant DNA reagent | pHACK-QF2Ir76b (plasmid) | This paper | HACK construct targeting the Ir76b gene; see ‘Plasmid construction’ | |
Recombinant DNA reagent | pHACK-QF2Ir25a (plasmid) | This paper | HACK construct targeting the Ir25a gene; see ‘Plasmid construction’ | |
Genetic reagent (Drosophila melanogaster) | Orco-T2A-QF2 knock-in | This paper | BDSC 92400, 92401, 92402 | See ‘Generation of HACK knock-in lines’ |
Genetic reagent (D. melanogaster) | Ir8a-T2A-QF2 knock-in | This paper | BDSC 92398, 92399 | See ‘Generation of HACK knock-in lines’ |
Genetic reagent (D. melanogaster) | Ir76b-T2A-QF2 knock-in | This paper | BDSC 92396, 92397 | See ‘Generation of HACK knock-in lines’ |
Genetic reagent (D. melanogaster) | Ir25a-T2A-QF2 knock-in | This paper | BDSC 92392, 92393, 92394, 92395 | See ‘Generation of HACK knock-in lines’ |
Genetic reagent (D. melanogaster) | 10XQUAS-CsChrimson (y[1] w[*]; Pin[1]/CyO; P(w[+mC] = 10XQUAS-CsChrimson.mVenus)11c) | This paper | BDSC 91996, FlyBase FBst0091996 | See ‘Fly stocks’ |
Genetic reagent (D. melanogaster) | Ir21a-T2A-Gal4 knock-in | Gift from Paul Garrity Marin et al., 2020 | ||
Genetic reagent (D. melanogaster) | Ir68a-T2A-Gal4 knock-in | Gift from Paul Garrity Marin et al., 2020 | ||
Genetic reagent (D. melanogaster) | Or7a-Gal4 knock-in (y[1] w[*] TI(GAL4)Or7a[KI-GAL4.w-]) | Potter lab | BDSC 91991, FlyBase FBti0214362 | |
Genetic reagent (D. melanogaster) | Ir64a-Gal4 | Gift from Greg Suh lab Ai et al., 2010 | ||
Genetic reagent (D. melanogaster) | Rh50-Gal4 | Menuz lab Vulpe et al., 2021 | ||
Genetic reagent (D. melanogaster) | Amt-Gal4 | Menuz lab Menuz et al., 2014 | ||
Genetic reagent (D. melanogaster) | Repo-Gal80 | Awasaki et al., 2011 | FlyBase FBtp0067904 | |
Genetic reagent (D. melanogaster) | wCS (Cantonized w1118) | Koh et al., 2014 | FlyBase FBrf0226011 | |
Genetic reagent (D. melanogaster) | Or35a-Gal4 | Yao et al., 2005 | ||
Genetic reagent (D. melanogaster) | Crey +1B (y[1] w[67c23] P(y[+mDint2] = Crey)1b; sna[Sco]/CyO) | Bloomington Drosophila Stock Center | BDSC 766, FlyBase FBti0012692 | |
Genetic reagent (D. melanogaster) | Gr21a-GAL4 (w[*]; P(w[ + mC] = Gr21a-GAL4.9.323)2/CyO; Dr[1]/TM3, Sb[+]) | Bloomington Drosophila Stock Center | BDSC 57600, FlyBase FBti0162643 | |
Genetic reagent (D. melanogaster) | Gr28b.d-GAL4 (w[*]; P(w[+mC] = Gr28b.d-GAL4)B27; Dr[1]/TM3, Sb[1]) | Bloomington Drosophila Stock Center | BDSC 57620, FlyBase FBst0057620 | |
Genetic reagent (D. melanogaster) | Ir25a-GAL4 (w[*]; P(w[ + mC] = Ir25a-GAL4.A)236.1; TM2/TM6B, Tb[1]) | Bloomington Drosophila Stock Center | BDSC 41728, FlyBase FBti0148895 | |
Genetic reagent (D. melanogaster) | Ir40a-GAL4 (w[*]; P(w[ + mC] = Ir40a-GAL4.3011)214.1; TM2/TM6B, Tb[1]) | Bloomington Drosophila Stock Center | BDSC 41727, FlyBase FBst0041727 | |
Genetic reagent (D. melanogaster) | Ir41a-GAL4 (w[*]; P(y[+ t7.7] w[+mC] = Ir41a-GAL4.2474)attP40; TM2/TM6B, Tb[1]) | Bloomington Drosophila Stock Center | BDSC 41749, FlyBase FBst0041749 | |
Genetic reagent (D. melanogaster) | Ir64a-GAL4 (w[*]; P(w[+mC] = Ir64a-GAL4.A)183.8; TM2/TM6B, Tb[1]) | Bloomington Drosophila Stock Center | BDSC 41732, FlyBase FBti0148898 | |
Genetic reagent (D. melanogaster) | Ir76a-GAL4 (w[*]; P(w[+ mC] = Ir76a-GAL4.PB)292.3B; TM2/TM6B, Tb[1]) | Bloomington Drosophila Stock Center | BDSC 41735, FlyBase FBst0041735 | |
Genetic reagent (D. melanogaster) | Ir76b-GAL4 (w[*]; P(w[ + mC] = Ir76b-GAL4.916)226.8; TM2/TM6B, Tb[+]) | Bloomington Drosophila Stock Center | BDSC 41730, FlyBase FBti0153291 | |
Genetic reagent (D. melanogaster) | Ir8a-GAL4 (w[*]; P(w[ + mC] = Ir8a-GAL4.A)204.8; TM2/TM6B, Tb[1]) | Bloomington Drosophila Stock Center | BDSC 41731, FlyBase FBti0148897 | |
Genetic reagent (D. melanogaster) | Or10a-Gal4 (w[*]; l(2)*[*]/CyO; P(w[ + mC] = Or10a-GAL4.C)134t1.3) | Bloomington Drosophila Stock Center | BDSC 23885, FlyBase FBti0102042 | |
Genetic reagent (D. melanogaster) | Or13a-Gal4 (w[*]; P(w[ + mC] = Or13a-GAL4.C)229t56.2/TM3, Sb[1]) | Bloomington Drosophila Stock Center | BDSC 23886, FlyBase FBti0102056 | |
Genetic reagent (D. melanogaster) | Or22a-Gal4 (w[*]; P(w[ + mC] = Or22a-GAL4.7.717)14.2) | Bloomington Drosophila Stock Center | BDSC 9951, FlyBase FBti0101805 | |
Genetic reagent (D. melanogaster) | Or22a-Gal4 (w[*]; P(w[ + mC] = Or22a-GAL4.7.717)14.21) | Bloomington Drosophila Stock Center | BDSC 9952, FlyBase FBti0101805 | |
Genetic reagent (D. melanogaster) | Or33c-Gal4 (w[*]; P(w[ + mC] = Or33c-GAL4.F)78.3) | Bloomington Drosophila Stock Center | BDSC 9966, FlyBase FBti0101843 | |
Genetic reagent (D. melanogaster) | Or35a-cd8GFP (w[*]; P(w[ + mC] = Or35a-Mmus\Cd8a.GFP)3/TM3, Sb[1]) | Bloomington Drosophila Stock Center | BDSC 52624, FlyBase FBti0156834 | |
Genetic reagent (D. melanogaster) | Or35a-Gal4 (w[*]; P(w[ + mC] = Or35a-GAL4.F)109.2A) | Bloomington Drosophila Stock Center | BDSC 9967, FlyBase FBti0101810 | |
Genetic reagent (D. melanogaster) | Or35a-Gal4 (w[*]; P(w[ + mC] = Or35a-GAL4.F)109.3) | Bloomington Drosophila Stock Center | BDSC 9968, FlyBase FBti0101844 | |
Genetic reagent (D. melanogaster) | Or42a-Gal4 (w[*]; P(w[ + mC] = Or42a-GAL4.F)48.3B) | Bloomington Drosophila Stock Center | BDSC 9970, FlyBase FBti0101811 | |
Genetic reagent (D. melanogaster) | Or42b-Gal4 (w[*]; P(w[ + mC] = Or42b-GAL4.F)64.3) | Bloomington Drosophila Stock Center | BDSC 9971, FlyBase FBti0101812 | |
Genetic reagent (D. melanogaster) | Or43b-Gal4 (w[*]; P(w[ + mC] = Or43b-GAL4.C)110t8.1) | Bloomington Drosophila Stock Center | BDSC 23894, FlyBase FBti0102047 | |
Genetic reagent (D. melanogaster) | Or46a-Gal4 (w[1,118]; P(w[ + mC] = Or46a-GAL4.G)32.1.y) | Bloomington Drosophila Stock Center | BDSC 23291, FlyBase FBti0076800 | |
Genetic reagent (D. melanogaster) | Or47a-Gal4 (w[*]; P(w[ + mC] = Or47a-GAL4.8.239)15.4A) | Bloomington Drosophila Stock Center | BDSC 9982, FlyBase FBti0101851 | |
Genetic reagent (D. melanogaster) | Or49b-Gal4 (w[*]; P(w[ + mC] = Or49b-GAL4.F)80.1) | Bloomington Drosophila Stock Center | BDSC 9986, FlyBase FBti0101853 | |
Genetic reagent (D. melanogaster) | Or59b-Gal4 (w[*]; P(w[ + mC] = Or59b-GAL4.C)114t2.2) | Bloomington Drosophila Stock Center | BDSC 23897, FlyBase FBti0102060 | |
Genetic reagent (D. melanogaster) | Or59c-Gal4 (w[*]; P(w[ + mC] = Or59c-GAL4.C)129t1.1) | Bloomington Drosophila Stock Center | BDSC 23899, FlyBase FBti0102061 | |
Genetic reagent (D. melanogaster) | Or65a-Gal4 (w[*]; Bl[1]/SM1; P(w[ + mC] = Or65a-GAL4.F)72.1) | Bloomington Drosophila Stock Center | BDSC 9994, FlyBase FBti0101857 | |
Genetic reagent (D. melanogaster) | Or67a-Gal4 (w[*]; P(w[ + mC] = Or67a-GAL4.C)137t3.3) | Bloomington Drosophila Stock Center | BDSC 23904, FlyBase FBti0102049 | |
Genetic reagent (D. melanogaster) | Or67b-Gal4 (w[*]; P(w[ + mC] = Or67b-GAL4.F)68.3/TM6B, Tb[1]) | Bloomington Drosophila Stock Center | BDSC 9995, FlyBase FBti0101858 | |
Genetic reagent (D. melanogaster) | Or67c-Gal4 (w[*]; P(w[ + mC] = Or67c-GAL4.C)116t3.2/CyO) | Bloomington Drosophila Stock Center | BDSC 23905, FlyBase FBti0102050 | |
Genetic reagent (D. melanogaster) | Or71a-Gal4 (w[*]; P(w[ + mC] = Or71a-GAL4.F)30.4) | Bloomington Drosophila Stock Center | BDSC 23122, FlyBase FBti0101860 | |
Genetic reagent (D. melanogaster) | Or83c-Gal4 (w[*]; P(w[ + mC] = Or83c-GAL4.F)73.3B) | Bloomington Drosophila Stock Center | BDSC 23131, FlyBase FBti0101829 | |
Genetic reagent (D. melanogaster) | Or85a-Gal4 (w[*]; P(w[ + mC] = Or85a-GAL4.F)67.2) | Bloomington Drosophila Stock Center | BDSC 23133, FlyBase FBti0101830 | |
Genetic reagent (D. melanogaster) | Or85b-Gal4 (w[*]; P(w[ + mC] = Or85b-GAL4.C)179t5.1) | Bloomington Drosophila Stock Center | BDSC 23911, FlyBase FBti0102053 | |
Genetic reagent (D. melanogaster) | Or85d-Gal4 (w[*]; P(w[ + mC] = Or85d-GAL4.C)143t2.1) | Bloomington Drosophila Stock Center | BDSC 24148, FlyBase FBti0102066 | |
Genetic reagent (D. melanogaster) | Or92a-Gal4 (w[*]; P(w[ + mC] = Or92a-GAL4.F)62.1) | Bloomington Drosophila Stock Center | BDSC 23139, FlyBase FBti0101867 | |
Genetic reagent (D. melanogaster) | Or98a-Gal4 (w[*]; P(w[ + mC] = Or98a-GAL4.F)115.1) | Bloomington Drosophila Stock Center | BDSC 23141, FlyBase FBti0101868 | |
Genetic reagent (D. melanogaster) | Orco-Gal4 (w[*]; P(w[ + mC] = Orco-GAL4.W)11.17; TM2/TM6B, Tb[1]) | Bloomington Drosophila Stock Center | BDSC 26818, FlyBase FBti0101150 | |
Genetic reagent (D. melanogaster) | QUAS reporter (y[1] w[1,118]; P(w[ + mC] = QUAS-mCD8::GFP.P)5B/TM6B, Tb[1]) | Bloomington Drosophila Stock Center | BDSC 30003, FlyBase FBti0129937 | |
Genetic reagent (D. melanogaster) | QUAS reporter (y[1] w[*]; PBac(y[ + mDint2] w[ + mC] = 10XQUAS-6XGFP)VK00018/CyO, P(Wee-P.ph0)Bacc[Wee-P20]) | Bloomington Drosophila Stock Center | BDSC 52264, FlyBase FBti0162759 | |
Genetic reagent (D. melanogaster) | UAS reporter (w[*]; P(y[ + t7.7] w[ + mC] = 10XUAS-IVS-mCD8::GFP)attP40) | Bloomington Drosophila Stock Center | BDSC 32186, FlyBase FBti0131963 | |
Genetic reagent (D. melanogaster) | UAS reporter (w[*]; P(y[ + t7.7] w[ + mC] = 15XUAS-IVS-mCD8::GFP)attP2) | Bloomington Drosophila Stock Center | BDSC 32193, FlyBase FBti0131935 | |
Genetic reagent (D. melanogaster) | UAS reporter (w[*]; P(y[ + t7.7] w[ + mC] = 10XUAS-IVS-mCD8::RFP)attP2) | Bloomington Drosophila Stock Center | BDSC 32218, FlyBase FBti0131950 | |
Genetic reagent (D. melanogaster) | UAS reporter (w[*]; P(y[ + t7.7] w[ + mC] = 10XUAS-IVS-mCD8::RFP)attP40) | Bloomington Drosophila Stock Center | BDSC 32219, FlyBase FBti0131967 | |
Genetic reagent (D. melanogaster) | UAS reporter (y[1] w[*]; PBac(y[ + mDint2] w[ + mC] = 20XUAS-6XGFP)VK00018/CyO, P(Wee-P.ph0)Bacc[Wee-P20]) | Bloomington Drosophila Stock Center | BDSC 52261, FlyBase FBti0162758 | |
Genetic reagent (D. melanogaster) | UAS reporter, sacculus experiments (UAS-mCD8::GFP (2nd)) | Lee and Luo, 1999 | FlyBase FBti0012685 | |
Genetic reagent (D. melanogaster) | UAS reporter, sacculus experiments (UAS-mCD8::GFP (3rd)) | Lee and Luo, 1999 | FlyBase FBti0012686 | |
Genetic reagent (D. melanogaster) | Orco2 mutant (w[*]; TI(w[ + m*] = TI)Orco[2]) | Bloomington Drosophila Stock Center | BDSC 23130, FlyBase FBti0168777 | |
Genetic reagent (D. melanogaster) | Ir25a2 mutant (w[*]; TI(w[ + m*] = TI)Ir25a[2]/CyO) | Bloomington Drosophila Stock Center | BDSC 41737, FlyBase FBti0168524 | |
Genetic reagent (D. melanogaster) | Ir8a1 mutant (w[*] TI(w[ + mW.hs] = TI)Ir8a[1]; Bl[1] L[2]/CyO) | Bloomington Drosophila Stock Center | BDSC 41744, FlyBase FBst0041744 | |
Genetic reagent (D. melanogaster) | Ir76b1 mutant (w[*]; Ir76b[1]) | Bloomington Drosophila Stock Center | BDSC 51309, FlyBase FBst0051309 | |
Genetic reagent (D. melanogaster) | Germline Cas9 (y[1] M(RFP[3xP3.PB] GFP[E.3xP3] = vas-Cas9)ZH-2A w[1,118]/FM7c) | Bloomington Drosophila Stock Center | BDSC 51323, FlyBase FBti0154823 | |
Genetic reagent (D. melanogaster) | Germline Cas9 (w[1,118]; PBac(y[ + mDint2] = vas-Cas9)VK00027) | Bloomington Drosophila Stock Center | BDSC 51324, FlyBase FBti0154822 | |
Genetic reagent (D. melanogaster) | Germline Cas9 (y[1] M(w[ + mC] = Act5C-Cas9.P)ZH-2A w[*]) | Bloomington Drosophila Stock Center | BDSC 54590, FlyBase FBti0159182 | |
Genetic reagent (D. melanogaster) | Double balancer (19ADrok/FM7c; Pin/CyO) | Potter lab stock | Derived from BDSC 6666, FBba0000009, FBal0013831, FBba0000025 | |
Genetic reagent (D. melanogaster) | Double balancer (y,w; Pin/CyO; Dh/TM6B) | Potter lab stock | Derived from FBal0013831, FBba0000025, FBti0004009, FBba0000057, FBal0016730 | |
Genetic reagent (D. melanogaster) | Double balancer (y,w; S/CyO; Pr/TM6B) | Potter lab stock | Derived from FBal0015108, FBba0000025, FBal0013944, FBba0000057, FBal0016730 | |
Genetic reagent (D. melanogaster) | Single balancer (y,w; +/+; Pr/TM6B) | Potter lab stock | Derived from FBal0013944, FBba0000057, FBal0016730 | |
Genetic reagent (D. melanogaster) | Single balancer (y,w; S/CyO; +/+) | Potter lab stock | Derived from FBal0015108, FBba0000025 | |
Genetic reagent (D. melanogaster) | Wildtype (w1118 IsoD1) | Gift from Thomas R. Clandinin | Derived from FBal0018186 | |
Genetic reagent (Drosophila sechellia) | Wildtype, genome Cousin Island, Seychelles | National Drosophila Species Stock Center | SKU:14021-0248.25 | |
Genetic reagent (Anopheles coluzzii) | Wildtype, N’Gousso strain | Gift from Insect Transformation Facility (Rockville, MD) Riabinina et al., 2016 | ||
Chemical compound, drug | Mineral oil | Sigma-Aldrich | CAS# 8042-47-5, Cat# 330779-1L | |
Chemical compound, drug | Ethyl acetate | Sigma-Aldrich | CAS# 141-78-6, Cat# 650528-1L, Cat# 270989-100ML | |
Chemical compound, drug | Pentyl acetate | Sigma-Aldrich | CAS# 628-63-7, Cat# 109584-250ML | |
Chemical compound, drug | Benzaldehyde | Sigma-Aldrich | CAS# 100-52-7, Cat# 418099-100ML, Cat# B1334-100G | |
Chemical compound, drug | Ethyl butyrate | Sigma-Aldrich | CAS# 105-54-4, Cat# E15701-500M, Cat# E15701-25ML | |
Chemical compound, drug | Hexanol | Sigma-Aldrich | CAS# 111-27-3, Cat# H13303-100ML | |
Chemical compound, drug | E2-hexenal | Sigma-Aldrich | CAS# 6728-26-3, Cat# W256005-1KG-K | |
Chemical compound, drug | Geranyl acetate | Sigma-Aldrich | CAS# 105-87-3, Cat# 173495-25G, Cat# 45896-1ML-F | |
Chemical compound, drug | 2-Heptanone | Sigma-Aldrich | CAS# 110-43-0, Cat# 537683-100ML | |
Chemical compound, drug | 1-Octen-3-ol | Sigma-Aldrich | CAS# 3391-86-4, Cat# O5284-25G, Cat# W280518-SAMPLE-K | |
Chemical compound, drug | 2,3-Butanedione | Sigma-Aldrich | CAS# 431-03-8, Cat# 11038-1ML-F | |
Chemical compound, drug | Phenylacetaldehyde | Sigma-Aldrich | CAS# 122-78-1, Cat# 107395-100ML | |
Chemical compound, drug | Phenethylamine | Sigma-Aldrich | CAS# 64-04-0, Cat# 241008-50ML | |
Chemical compound, drug | Propionic acid | Sigma-Aldrich | CAS# 79-09-4, Cat# 402907-100ML | |
Chemical compound, drug | 1,4-Diaminobutane | Sigma-Aldrich | CAS# 110-60-1, Cat# D13208-100G | |
Chemical compound, drug | Pyrrolidine | Sigma-Aldrich | CAS# 123-75-1, Cat# P73803-100ML, Cat# P73803-5ML | |
Chemical compound, drug | P-cresol | Sigma-Aldrich | CAS# 106-44-5, Cat# 42429-5G-F, Cat# C85751-5G | |
Chemical compound, drug | Methyl salicylate | Sigma-Aldrich | CAS# 119-36-8, Cat# M6752-250ML | |
Chemical compound, drug | Paraffin Oil | ACROS Organics | CAS# 8012-95-1, Cat# 171400010 | |
Chemical compound, drug | Water | SIGMA Life Science | CAS# 7732-18-5, Cat# W3500 | |
Chemical compound, drug | Acetic acid | Fisher Scientific | CAS# 64-19-7, Cat# A38S | |
Chemical compound, drug | Ammonium hydroxide | Fisher Scientific | CAS# 7664-41-7, Cat# A669S | |
Chemical compound, drug | Cadaverine | Sigma-Aldrich | CAS# 462-94-2, Cat# 33211-10ML-F | |
Chemical compound, drug | Hexanol | ACROS Organics | CAS# 111-27-3, Cat# AC43386 | |
Chemical compound, drug | 2,3-Butanedione | ACROS Organics | CAS# 431-03-8, Cat# AC10765 | |
Chemical compound, drug | Phenethylamine | ACROS Organics | CAS# 64-04-0, Cat# AC156491000 | |
Chemical compound, drug | Propanal | ACROS Organics | CAS# 123-38-6, Cat# AC220511000 | |
Chemical compound, drug | 1,4-Diaminobutane | ACROS Organics | CAS# 110-60-1, Cat# AC11212-250 | |
Chemical compound, drug | All trans-retinal | Sigma-Aldrich | CAS# 116-31-4, Cat# R2500 | |
Commercial assay or kit | HCR v3.0 | Molecular Instruments | ||
Commercial assay or kit | In-Fusion Cloning | Clontech Labs | Cat# 639645 | |
Commercial assay or kit | DNeasy Blood and Tissue Kit | QIAGEN | Cat# 69506 | |
Software, algorithm | Fiji (ImageJ) | Schindelin et al., 2012 | https://imagej.net/Fiji | |
Software, algorithm | GraphPad Prism 8.0 | GraphPad Software | http://www.graphpad.com/ | |
Software, algorithm | AutoSpike | Syntech | http://www.ockenfels-syntech.com/products/signal-acquisition-systems-2/ | |
Software, algorithm | LabChart Pro v8 | ADInstruments | https://www.adinstruments.com/support/downloads/windows/labchart | |
Software, algorithm | Adobe Illustrator CS6 | Adobe, Inc | https://www.adobe.com/products/illustrator.html | |
Software, algorithm | Adobe Photoshop CS6 | Adobe, Inc | https://www.adobe.com/products/photoshop.html | |
Software, algorithm | MacVector 16.0 | MacVector, Inc | https://macvector.com/ | |
Software, algorithm | MEGA11 | Tamura et al., 2021 | https://www.megasoftware.net/ | |
Software, algorithm | Zen Black | Carl Zeiss Microscopy | https://www.zeiss.com/microscopy/us/products/microscope-software/zen.html | |
Software, algorithm | Venn Diagram web tool | VIB/UGent Bioinformatics & Evolutionary Genomics | http://bioinformatics.psb.ugent.be/webtools/Venn/ | |
Software, algorithm | Amira | Thermo Fisher Scientific | https://www.thermofisher.com/us/en/home/industrial/electron-microscopy/electron-microscopy-instruments-workflow-solutions/3d-visualization-analysis-software/amira-life-sciences-biomedical.html | |
Software, algorithm | Python packages | Schlegel et al., 2021 | https://github.com/schlegelp/navis, https://github.com/flyconnectome/fafbseg-py | |
Software, algorithm | Q-Capture Pro 7 | Teledyne QImaging | https://www.qimaging.com/home | |
Other | DAPI stain | Invitrogen | D1306 | IHC (1:10,000) |
Sequence-based reagent | Orco_gRNA_FOR | This paper, Integrated DNA Technologies | PCR primers | TCCGGGTGAACT TCGCACAGTGCG GAGGGGGCAAG TTTTAGAGCT AGAAATAGCAAGTTA |
Sequence-based reagent | Orco_gRNA_REV | This paper, Integrated DNA Technologies | PCR primers | TTCTAGCTCTAAAAC ACTTTATGGTGCT GGTGCAGCGACGTTA AATTGAAAATAGGTC |
Sequence-based reagent | Orco_5HA_FOR | This paper, Integrated DNA Technologies | PCR primers | CCCTTACGTAACGCGTCAGCTT GTTTGACTTACTTGATTAC |
Sequence-based reagent | Orco_5HA_REV | This paper, Integrated DNA Technologies | PCR primers | CGCGGCCCTCACGCGTCTTGA GCTGTACAAGTACCATAAAGT |
Sequence-based reagent | Orco_3HA_FOR | This paper, Integrated DNA Technologies | PCR primers | GTTATAGATCACTAGTCTCAG TACTATGCAACCAGCAATA |
Sequence-based reagent | Orco_3HA_REV | This paper, Integrated DNA Technologies | PCR primers | AATTCAGATCACTAGTGTTTT ATGAAAGCTGCAAGAAATAA |
Sequence-based reagent | Ir8a_gRNA_FOR | This paper, Integrated DNA Technologies | PCR primers | TCCGGGTGAACTTCGTTTGT TTGTTCGGCCATGTGTTTTAG AGCTAGAAATAGCAAGTTA |
Sequence-based reagent | Ir8a_gRNA_REV | This paper, Integrated DNA Technologies | PCR primers | TTCTAGCTCTAAAACCTGTGG GAGTCAGAGGCACCGACGT TAAATTGAAAATAGGTC |
Sequence-based reagent | Ir8a_5HA_FOR | This paper, Integrated DNA Technologies | PCR primers | CCCTTACGTAACGCGtCTATT GGCTATTCGTCGTACTCATGC |
Sequence-based reagent | Ir8a_5HA_REV | This paper, Integrated DNA Technologies | PCR primers | CGCGGCCCTCACGCGtCTCCA TGTAGCCACTATGTGAGTCAGAT |
Sequence-based reagent | Ir8a_3HA_FOR | This paper, Integrated DNA Technologies | PCR primers | GTTATAGATCACTAGtGTTTCTT GTCGCACCTAATTAACAAGTG |
Sequence-based reagent | Ir8a_3HA_REV | This paper, Integrated DNA Technologies | PCR primers | AATTCAGATCACTAGtCATAC TTAAGCTCCTTGAGGTCCAGC |
Sequence-based reagent | Ir76b_gRNA_FOR | This paper, Integrated DNA Technologies | PCR primers | TCCGGGTGAACTTCGCAGT GATGCGAACTTCATAGTTTT AGAGCTAGAAATAGCAAGTTA |
Sequence-based reagent | Ir76b_gRNA_REV | This paper, Integrated DNA Technologies | PCR primers | TTCTAGCTCTAAAACCGGCG GCCCTCTTTCAATACGACG TTAAATTGAAAATAGGTC |
Sequence-based reagent | Ir76b_5HA_FOR | This paper, Integrated DNA Technologies | PCR primers | CCCTTACGTAACGCGtACCAATG AATCCTTGTCCATGCTAAA |
Sequence-based reagent | Ir76b_5HA_REV | This paper, Integrated DNA Technologies | PCR primers | CGCGGCCCTCACGCGtCTC GGTGTAGCTGTCTTGAAGGAA |
Sequence-based reagent | Ir76b_3HA_FOR | This paper, Integrated DNA Technologies | PCR primers | GTTATAGATC ACTAGtGCCTA ATTGGAATACC TTCTACATAATGGA |
Sequence-based reagent | Ir76b_3HA_REV | This paper, Integrated DNA Technologies | PCR primers | AATTCAGATCACTAGtGGCAA GGCACAAAATAAAACGAAG |
Sequence-based reagent | Ir25a_gRNA_FOR | This paper, Integrated DNA Technologies | PCR primers | TCCGGGTGAACTTCGCCGGA TACTGATTAAAGCGGTTTTA GAGCTAGAAATAGCAAGTTA |
Sequence-based reagent | Ir25a_gRNA_REV | This paper, Integrated DNA Technologies | PCR primers | TTCTAGCTCTAAAACAGTG CTCATTTTACCATAATCGA CGTTAAATTGAAAATAGGTC |
Sequence-based reagent | Ir25a_5HA_FOR | This paper, Integrated DNA Technologies | PCR primers | CCCTTACGTAACGCGtTGC ATGACTTCATTGACACCTCAAG |
Sequence-based reagent | Ir25a_5HA_REV | This paper, Integrated DNA Technologies | PCR primers | CGCGGCCCTCACGCGtGAA ACGAGGCTTAAACGTAGCTGGATATT |
Sequence-based reagent | Ir25a_3HA_FOR | This paper, Integrated DNA Technologies | PCR primers | GTTATAGATCACTAGtAATAT TATGGTTAAGTGAGCTCTCGG |
Sequence-based reagent | Ir25a_3HA_REV | This paper, Integrated DNA Technologies | PCR primers | AATTCAGATCACTAGtCAAA GCTAAGTTCATCGTCATAGAGAC |
Sequence-based reagent | Orco_Seq_FOR | This paper, Integrated DNA Technologies | PCR primers | GATGTTCTGCTCTTGGCTGATATTC |
Sequence-based reagent | Ir8a_Seq_FOR | This paper, Integrated DNA Technologies | PCR primers | CATCGACTTCATCATCAGGCTTTCG |
Sequence-based reagent | Ir76b_Seq_FOR | This paper, Integrated DNA Technologies | PCR primers | CAACGATATCCTCACGAAGAACAAGC |
Sequence-based reagent | Ir25a_Seq_FOR | This paper, Integrated DNA Technologies | PCR primers | CGAAAGGATACAAAGGATACTGCAT |
Sequence-based reagent | HACK_Seq_REV | This paper, Integrated DNA Technologies | PCR primers | TGTATTCCGTCGCATTTCTCTC |
Sequence-based reagent | IVS-FOR | This paper, Integrated DNA Technologies | PCR primers | TGGGTTGGACTCAGGGAATA GATCTAAAAGGTAGGTTCAACCACT |
Sequence-based reagent | EcoRI-SV40-REV | This paper, Integrated DNA Technologies | PCR primers | GCTTACGTCAGAATTCAGA TCGATCCAGACATGATAAGA |
Sequence-based reagent | Tsp42Ej_FOR | This paper, Integrated DNA Technologies | PCR primers | GAGAAGTCGTTTCCCATAACACCCT |
Sequence-based reagent | Tsp42Ej_REV | This paper, Integrated DNA Technologies | PCR primers | GAGGAGCAGTTTTCGGAGTCGCCTTC |
Sequence-based reagent | D. melanogaster Orco | This paper, Molecular Instruments | RNA in situ HCR probe set | See Appendix 2 ‘In situ probe sequences’ |
Sequence-based reagent | D. melanogaster Ir25a | This paper, Molecular Instruments | RNA in situ HCR probe set | See Appendix 2 ‘In situ probe sequences’ |
Sequence-based reagent | D. melanogaster Ir40a | This paper, Molecular Instruments | RNA in situ HCR probe set | See Appendix 2 ‘In situ probe sequences’ |
Sequence-based reagent | D. melanogaster Ir51a | This paper, Molecular Instruments | RNA in situ HCR probe set | See Appendix 2 ‘In situ probe sequences’ |
Sequence-based reagent | D. melanogaster Ir60a | This paper, Molecular Instruments | RNA in situ HCR probe set | See Appendix 2 ‘In situ probe sequences’ |
Sequence-based reagent | D. melanogaster Ir62a | This paper, Molecular Instruments | RNA in situ HCR probe set | See Appendix 2 ‘In situ probe sequences’ |
Sequence-based reagent | D. melanogaster Ir76a | This paper, Molecular Instruments | RNA in situ HCR probe set | See Appendix 2 ‘In situ probe sequences’ |
Sequence-based reagent | D. melanogaster Ir76b | This paper, Molecular Instruments | RNA in situ HCR probe set | See Appendix 2 ‘In situ probe sequences’ |
Sequence-based reagent | D. melanogaster Ir93a | This paper, Molecular Instruments | RNA in situ HCR probe set | See Appendix 2 ‘In situ probe sequences’ |
Sequence-based reagent | Anopheles coluzzii Orco | This paper, Molecular Instruments | RNA in situ HCR probe set | See Appendix 2 ‘In situ probe sequences’ |
Sequence-based reagent | A. coluzzii Ir25a | This paper, Molecular Instruments | RNA in situ HCR probe set | See Appendix 2 ‘In situ probe sequences’ |