Myelination synchronizes cortical oscillations by consolidating parvalbumin-mediated phasic inhibition
Figures
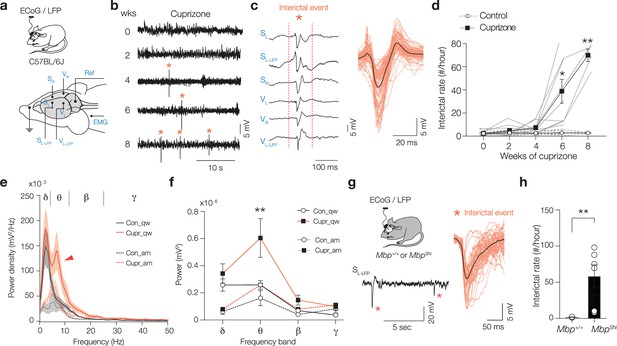
Loss of compact myelin causes interictal spikes and behavioral state-dependent amplification of theta rhythms.
(a) Schematic of the electrocorticogram (ECoG) and local field potential (LFP) recordings in freely moving mice. Electrodes were placed right (SR) and left (SL) in the primary somatosensory cortex, and a left LFP electrode (SL-LFP) into layer 5 (L5). A similar array of electrodes was positioned in the primary visual cortex (VR, VL, and VL-LFP). One electrode was placed around neck muscle recording electromyography (EMG) and one used as reference (Ref). (b) Interictal spikes (*) appear from 4-week cuprizone and onward. Example raw LFP traces (SL-LFP). (c) Representative interictal spike example showing spatiotemporal synchronization of the spike across cortical areas and hemispheres. Higher magnification of interictal spikes (red, ~50–300 ms duration) overlaid with the average (black). (d) Cuprizone treatment caused a progressively increasing frequency of interictal spikes (mixed-effects two-way ANOVA). Time × treatment interaction p<0.0001, Šidák’s multiple comparisons tests, cupri vs. con; 4 weeks p=0.121, 6 weeks *p=0.0406 and 8 weeks **p=0.0054. (e) Power spectral content during two different brain states, awake and moving (am, dotted lines) and quiet wakefulness (qw, solid lines) in control (black) and cuprizone (red). Red arrow marks amplified theta band power (θ) during quite wakefulness in cuprizone mice (Cupr_qw). (f) Cuprizone amplifies selectively θ power during quiet wakefulness (two-way ANOVA treatment p<0.0001, Šidák’s multiple comparisons cupr vs. con; for δ, p=0.8118; θ, p=0.0013; β, p=0.8568 and for γ, p=0.9292) but not during moving (two-way ANOVA treatment p=0.0575). (g) Left: schematic of ECoG and LFP recordings from Mbp+/+ and MbpShi mice with example trace showing interictal spikes (*). Right: overlaid individual interictal spikes in MbpShi mice (red) combined with the population average (black). (h) Bar plot of interictal rate in MbpShi mice. Two-tailed Mann–Whitney test **p=0.0095. Data shown as mean ± SEM with gray lines (d) or open circles (h) individual mice.
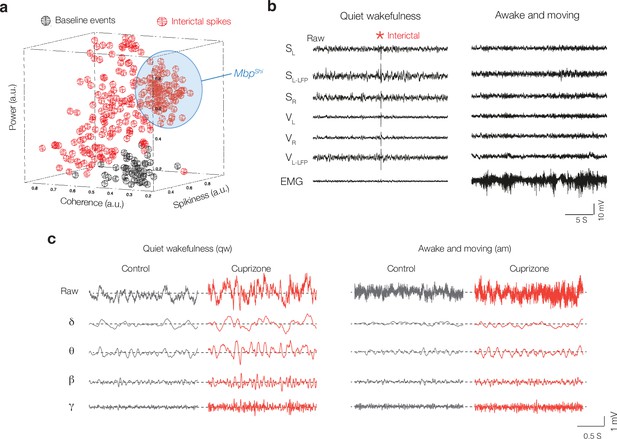
State-dependent interictal activity in cuprizone mice and automated interictal event detection library.
(a) Automated event detection library used for interictal classification. Three-dimensional projection metric space, showing coherence, spikiness, and signal power (a.u. = arbitrary units), with colors corresponding to interictal (red) and baseline/normal (black) events. The event library was constructed by an operator who classified events as ‘normal’ or interictal events. The blue domain represents the population of interictal events from MbpShi mice. (b) Example of a 30 s recording from multiple electrodes from 6-week cuprizone-treated mouse. Left: traces during the awake state, note the high-voltage electromyography (EMG) activity. Right: same mouse during quiet wakefulness with low EMG activity. Interictal discharge indicated with red asterisk (*). (c) Example traces showing raw local field potential (LFP) signals from S1 (top) and bandpass-filtered traces (bottom) at different brain states in control (black) and cuprizone (red) for delta (δ, 0.5–3.5 Hz), theta (θ, 4–12 Hz), beta (β, 12.5–25 Hz), and gamma (γ, 30–80 Hz).
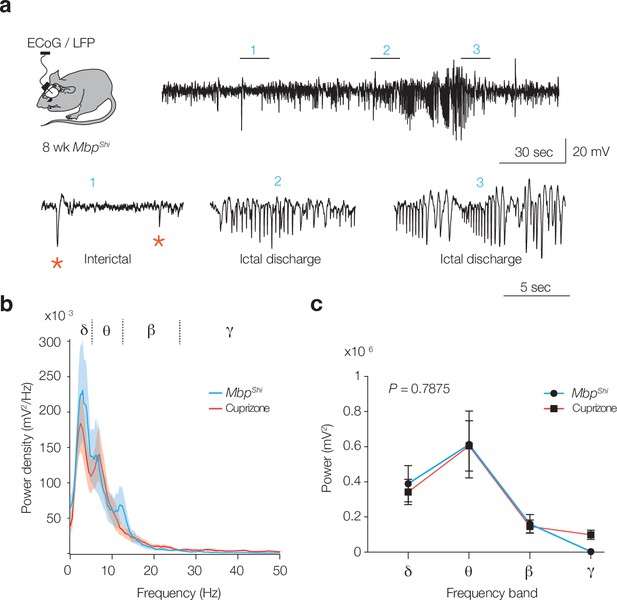
Ictal, interictal activity, and power spectrum in MbpShi mice.
(a) Schematic drawing showing electrocorticogram (ECoG) and local field potential (LFP) recordings from S1 of Mbp+/+ and MbpShi mice. Example LFP trace showing pre-ictal and ictal discharge from 8-week-old MbpShi mouse (top). Bottom: higher temporal resolution of the top LFP trace showing ECoG activity with interictals (1) and ictal discharge (2 and 3). (b, c) Comparable power spectral content during interictal activity in cuprizone (red) and MbpShi (blue) (two-way ANOVA myelin models p<0.0001, Šidák’s multiple comparisons cuprizone vs. MbpShi; for δ, p=0.9952; θ, p>0.9999; β, p>0.9999 and for γ, p=0.9346). Data shown as mean ± SEM.
Brain state-dependent interictal spikes.
Example video of electrocorticogram (ECoG), local field potential (LFP), and behavioral recordings in a 6-weeks cuprizone-treated mouse. Raw signals show ECoG from right and left primary somatosensory cortex (SR, SL, respectively) and LFP from the left layer 5 (L5) region (SL-LFP). Similar configuration for primary visual cortex (VR, VL, and VL-LFP). An additional electrode was connected to the neck muscle recording electromyography (EMG). Note the high EMG activity during awake and moving states. Interictal epileptiform discharges were automatically detected (red asterisks) and only occur during quiet wakefulness.
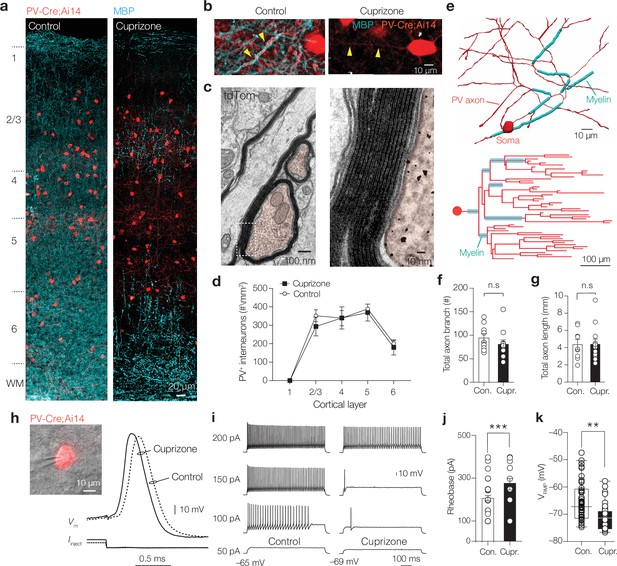
Demyelination preserves parvalbumin-positive (PV+) interneuron number and morphology but reduces excitability.
(a) Left: confocal z-projected overview image of S1 in a PV-Cre; Ai14 mouse (tdTomato+, red) overlaid with myelin basic protein (MBP, cyan). Right: overview image showing loss of MBP after + weeks cuprizone. (b) Myelinated PV+ axons in control (left) and PV+ axons demyelination with cuprizone treatment (right). (c) Electron microscopy (EM) of transverse cut tdTomato+ immunogold-labeled axons (false-colored red). Right: higher magnification of immunogold particles and ultrastructure of the PV interneuron myelin sheath. (d) PV+ interneuron number across the cortical lamina was not affected by cuprizone treatment (two-way ANOVA, treatment effect p=0.6240). (e) Top: example of a high-resolution 3D reconstruction of a biocytin-labeled PV axon (red) labeled with MBP (cyan) of a control mouse, showing the first approximately six axonal branch orders. Bottom: control axonogram showing axon branch order and myelinated segments (cyan). (f, g) Total axon branch number and length are not changed by demyelination (Mann–Whitney tests p=0.3032 and p=0.8822, respectively). n.s., not significant. (h) Left: brightfield/fluorescence overlay showing patch-clamp recording from a tdTomato+ interneuron. Right: example PV+ interneuron APs from control (dotted line) and cuprizone-treated mice (continuous lines). (i) Steady-state sub- and suprathreshold voltage responses during 700 ms current injections. Firing rates near threshold reduced and the (j) rheobase current significantly increased in cuprizone (***p=0.0003). (k) PV+ basket cells (BCs) showed an ~4 mV hyperpolarized resting membrane potential (**p=0.0036). Data shown as mean ± SEM and open circles individual cells.
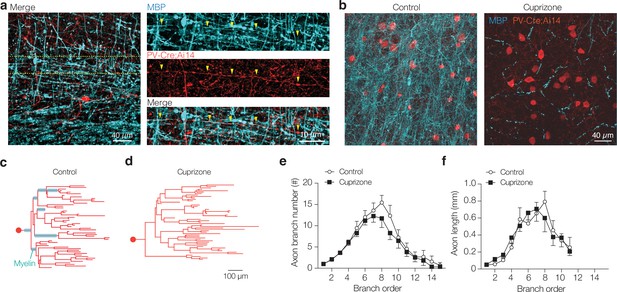
Cuprizone treatment causes loss of parvalbumin-positive (PV+) axon myelination but preserves axon length and complexity.
(a) Left: confocal image of control staining in layer 5 (L5) of S1 showing a PV+ interneuron. Right: higher magnification of the image in right, illustrating the trajectory of a myelinated PV+ interneuron axon (yellow arrows). Note that also PV+ axon swellings are frequently myelinated. (b) A confocal z-stack image examples of the L5 region in control (left) and cuprizone (right)-treated mice. (c) Axonograms of a control and (d) a cuprizone axon. Myelinated segments indicated with cyan. (e, f) Segment number and length per branch order. Both number and length of axon segments were not significantly affected by cuprizone-induced demyelination (two-way ANOVA treatment × branch order interaction p=0.8028 and p=0.6236, respectively). Data shown as mean ± SEM.
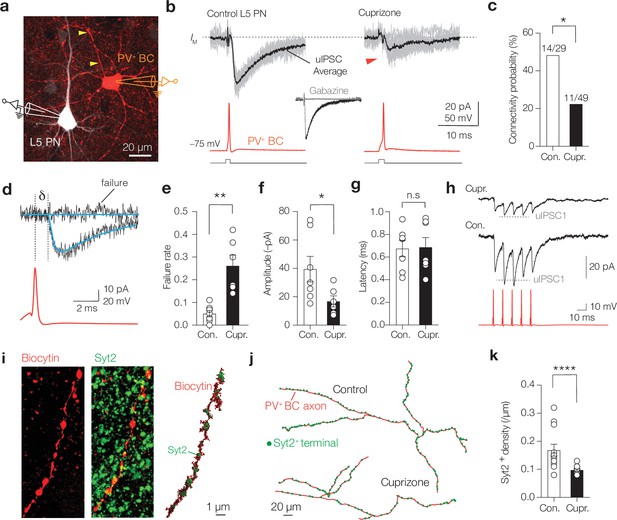
Demyelination decreases connectivity, reliability, and rapid facilitation of parvalbumin-positive (PV+) unitary inhibitory postsynaptic currents (uIPSCs).
(a) Immunofluorescence image of a connected control PV+ basket cell (BC) (red) and layer 5 (L5) pyramidal neuron (PN) (white). (b) Example traces of 10 single-trial uIPSC traces (gray) overlaid with mean average (>60 trials, black). Inset: uIPSCs abolished by gabazine (GABAA blocker, 4 µM). (c) Cuprizone-treated mice show significantly lower connection probability between PV+ BC and L5-PN (chi-square test *p=0.0182, n = 78 pairs). (d) Example fits (blue) of uIPSCs for rise- and decay time, amplitude, failure rate, amplitude, and latency analyses (δ, AP to 10% uIPSC peak amplitude). (e) Cuprizone increased failures by fivefold and the average peak amplitude by ~2.5-fold (Mann–Whitney test **p=0.012) and (f) reduces the mean amplitude (Mann–Whitney test *p=0.0256). (g) uIPSCs latency remained unchanged (Mann–Whitney test n.s., p>0.999). (h) Cuprizone impairs short-term facilitation. Dotted line indicates expected amplitude for uIPSC2 (scaled from uIPSC1). (i) Left: confocal z-projected image of a control PV+ axon (red) immunostained with Syt2 (green). Right: surface-rendered 3D image of the same axon. (j) Example sections of 3D reconstructions. (k) Cuprizone increased the Syt2+ puncta spacing by approximately twofold (Mann–Whitney test ****p<0.0001, n = 12). Data shown as mean ± SEM and open circles individual axons or pairs. n.s., not significant.
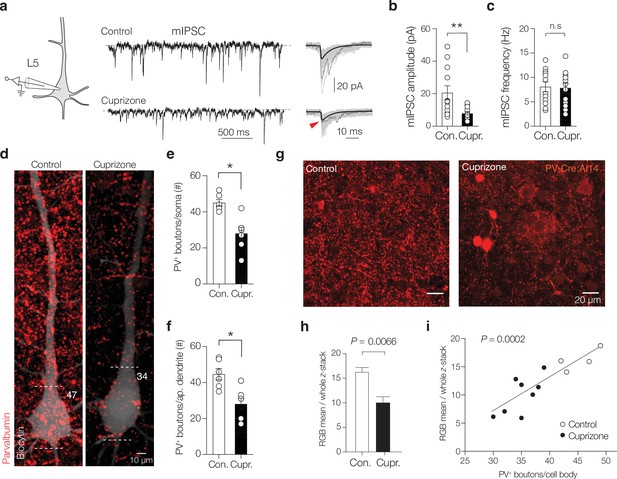
Cuprizone decreases miniature inhibitory postsynaptic currents (mIPSCs) and somatodendritic parvalbumin (PV) puncta.
(a) Left: example traces of miniature inhibitory postsynaptic currents (mIPSCs) at the soma of layer 5 (L5) pyramidal neurons in the presence of CNQX, d-AP5, and TTX in control (top) and demyelinated conditions (bottom). (b, c) Population data showing an approximately threefold mIPSC peak amplitude but not frequency reduction (Mann–Whitney test **p=0.0025; n.s., p=0.728). (d) Example images of maximum z-projection of a biocytin-filled L5 pyramidal neurons (PNs) (white) overlaid with PV immunofluorescence (red). Dotted lines indicate the soma borders with number indicating counted PV+ puncta. (e, f) Population analysis reveals a significant loss in the number of PV+ puncta at the L5 soma (Mann–Whitney test *p=0.0035) and the primary proximal apical dendrite (<200 µm, *p=0.0044). (g, h) Example confocal z-stack images of L5 in control (left) and cuprizone-treated mice (right) reveal a global significant reduction in PV immunofluorescence intensity. (i) Regression plot reveals the mean PV immunofluorescence intensity correlates with the number of PV+ boutons on large NeuN+ pyramidal neurons cell bodies (r2 = 0.755). NeuN+ immunofluorescent signals are not shown. The soma diameters of pyramidal neurons were unchanged (cuprizone 18.23 ± 0.99 µm, n = 6 vs. 18.37 ± 0.68 µm, Mann–Whitney U-test p=0.954, n = 9).
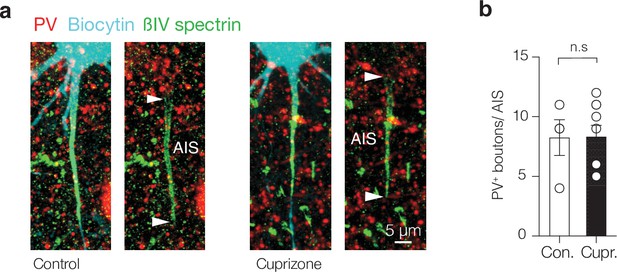
Putative parvalbumin-positive (PV+) chandelier inputs at the axon initial segment (AIS) are unaffected by cuprizone-induced demyelination.
(a) A confocal z-projection of a biocytin-filled (cyan) layer 5 (L5) pyramidal neuron overlaid with βIV spectrin (green) and parvalbumin (+). (b) The number of putative chandelier PV+ boutons was preserved in cuprizone-treated mice (Mann–Whitney test p=0.96). Data are shown as mean ± SEM and open circles individual neurons. n.s., not significant.
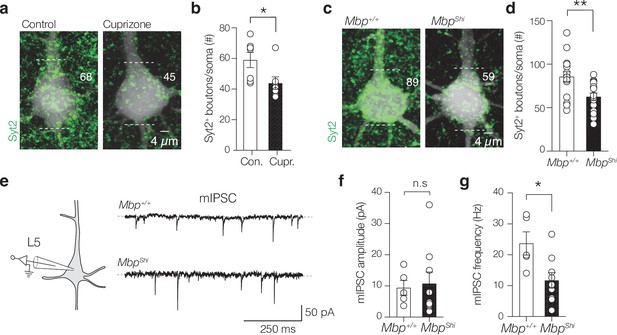
Demyelination and dysmyelination reduces miniature inhibitory postsynaptic currents (IPSCs) and perisomatic Syt2+ puncta.
(a) Maximum z-projection of biocytin-filled layer 5 (L5) pyramidal neuron (PN) (white) overlaid with Syt2+ immunofluorescence (green). Numbers indicate the Syt2+ puncta counted at the soma. (b) Population analysis reveals a significant Syt2+ puncta loss (two-tailed Mann–Whitney U-test *p=0.0216). (c) Maximum z-projection of a biocytin-filled L5 soma (white) overlaid with Syt2+ immunofluorescence (green) from Mbp+/+ and MbpShi mice. (d) Population analysis shows a significant loss of Syt2+ puncta in the MbpShi mice (**p=0.0013). (e) Example traces of miniature inhibitory postsynaptic currents (mIPSCs) of L5 PNs in the presence of CNQX, d-AP5, and TTX in Mbp+/+ (top) and MbpShi mice (bottom). (f, g) mIPSCs’ peak amplitude was unaffected but frequency is reduced in MbpShi (n.s., p=0.797 and *p=0.019, respectively). Data are shown as mean ± SEM and open circles individual neurons. n.s., not significant.
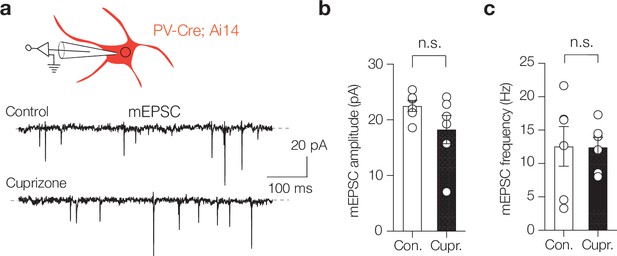
Demyelination does not affect excitatory drive of parvalbumin-positive (PV+) basket cells (BCs).
(a) Left: schematic of whole-cell voltage-clamp recording for mEPSCs in identified parvalbumin interneurons in the PV-Cre; Ai14 mouse line. mEPSCs were recorded in control mice and mice with 6-week cuprizone feeding. (b, c) Population data of mEPSC recordings revealed no difference in amplitude (Mann–Whitney test, n.s., p=0.240) nor mEPSC frequency (Mann–Whitney test, n.s., p=0.937). Data are shown as mean ± SEM and open circles individual neurons. n.s., not significant.
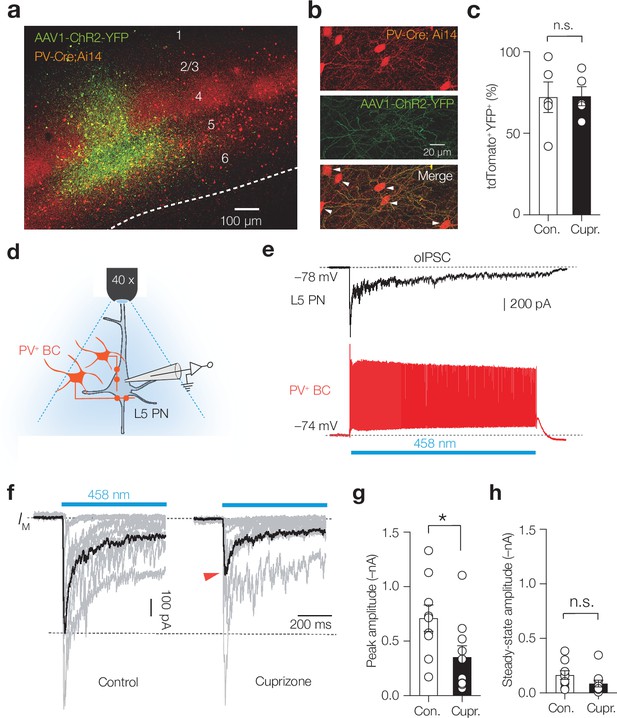
Demyelination impairs phasic parvalbumin-positive (PV+) basket cell (BC) inhibition of layer 5 (L5) pyramidal neurons (PNs).
(a) Immunofluorescent image of AAV1-hChR2-YFP expression (green) injected into L5 of S1. (b) Confocal images of separate fluorescent channels showing td-Tomato+ cell bodies and neurites (red, top), the localization of AAV1-hChR2-YFP (green, middle), and the merge image (bottom). The majority of tdTomato+ cells were YFP+ (white arrows). (c) Average transfection rate of AAV1-hChR2-YFP in the L5 (>70%) is comparable in control and cuprizone conditions (Mann–Whitney test p=0.889, n = 5). (d) Schematic showing full-field blue light optogenetically evoked postsynaptic inhibitory currents (oIPSCs) in L5 PNs. (e) Example trace of a whole-cell current-clamp recording from a PV+ interneurons (bottom red) compared to a separate whole-cell voltage-clamp recordings from an L5 PN. A 1 s blue light field illumination (blue bar) produces sustained firing in PV-Cre AAV1-ChR2 interneurons. (f) Single trial oIPSCs (gray) from different experiments (1 s duration pulses) overlaid with the average oIPSC (black) revealing a lower peak amplitude in cuprizone (red arrow). (g) Population data revealed an approximately twofold reduction in oIPSCs’ peak amplitude (Mann–Whitney test p=0.0172). (h) Steady-state oIPSCs’ amplitude did not reach significance, n.s., Mann–Whitney test p=0.0789.
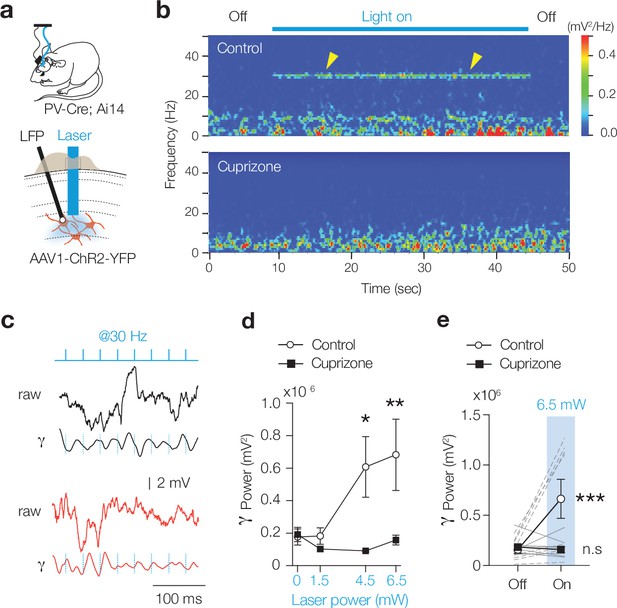
Demyelination impairs optogenetically evoked γ entrainment.
(a) Schematic for chronic local field potential (LFP) recordings and in vivo optogenetic stimulation in freely moving PV-Cre; Ai14 mice. (b) Time frequency plot showing low gamma frequency (γ) entrainment (40 blue light pulses of 1 ms duration at 30 Hz) in control but not in cuprizone mice. (c) Raw LFP (top) and bandpass-filtered trace (25–40 Hz, bottom) from control and cuprizone during low-γ entrainment. (i) Population data of γ power with increasing laser power reveals impaired γ in cuprizone-treated mice. (d) Myelin-deficient mice low-γ band entrainment to optogenetic stimuli (two-way ANOVA followed by Šidák’s multiple comparisons cuprizone vs. control, 0 mW, p>0.999; 1.5 mW, p=0.979; 4.5 mW, *p=0.0221, 6.5 mW, **p=0.0046). (e) Data are shown as mean ± SEM with gray lines individual cells, gray lines individual mice. n.s., not significant.
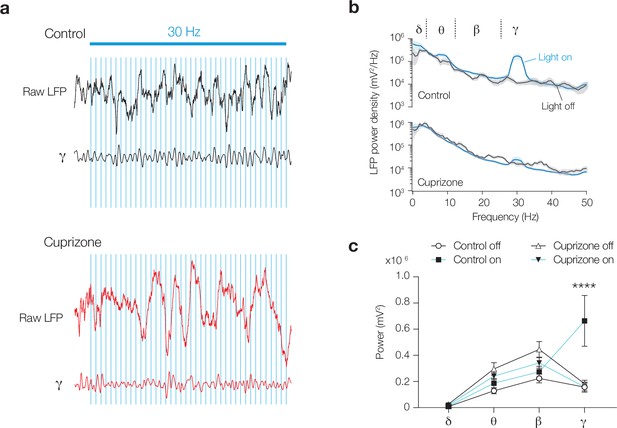
Myelin loss abolishes optogenetically evoked entrainment of γ rhythm.
(a) Raw local field potential (LFP) and low gamma (γ) 25–40 Hz bandpass-filtered trace during 30 Hz blue light stimulation in control (black) and cuprizone (red) mice. Blue light shifted the phase or extended the γ cycle in control mice. (b) Averaged power spectral density content of low-γ entrainment during light on (blue lines) or off (black lines). (c) 30 Hz blue light stimulation (blue lines) showed a lack of γ band entrainment in cortex of demyelinated mice (closed triangles) in comparison to the control mice (closed squares). Two-way ANOVA frequency × treatment p=0.0002, Šidák’s multiple comparisons tests; γ, ****p<0.0001; all other bands p>0.910. Data shown as mean ± SEM.
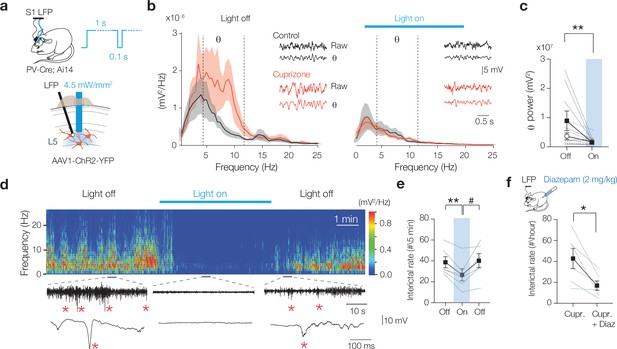
Optogenetic activation of myelin-deficient parvalbumin-positive (PV+) interneurons rescues theta rhythm and interictal epileptiform discharges.
(a) Schematic drawing for chronic local field potential (LFP) and optogenetic stimulation in freely moving mice. A 1 s blue light pulse with 100 ms off periods activated PV+ interneurons. Blue light was switched on during high interictal activity (>10 spikes/min). (b) Power spectral content collected from 2 s epoch windows in control (black) and cuprizone (red) before (left) and during 3 min (right) optogenetic activation of PV+ interneurons. Insets: raw LFP signals (top) and θ content (bottom) in control (black) and cuprizone (red) condition. (c) Population data showing optogenetic activation of PV+ cells attenuated the amplified θ frequency in cuprizone mice to control levels (Šidák’s multiple comparisons test in cuprizone, light on vs. light off for θ, **p=0.0076). (d) Example time frequency plot (top) and raw LFP traces (below) showing suppression of interictal spikes during light on conditions. See also Figure 6—video 1. (e) Population data of transient optogenetic suppression of the interictal activity in 8-week cuprizone-treated mice (one-way ANOVA p=0.0165, Tukey’s multiple comparisons tests; off vs. on, **p=0.0089; on vs. off, p=0.0539; off vs. off, p=0.928). (f) 2 mg/kg i.p. injection of diazepam in cuprizone-treated mice reduces interictal rate for at least 10 hr (two-tailed Student’s t-test *p=0.024). Data shown as mean ± SEM and gray lines individual mice.
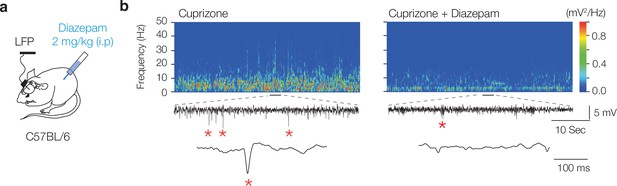
GABAA receptor agonism suppresses interictal epileptiform discharge frequency.
(a) The GABAA receptor agonist, diazepam, was injected i.p. at 7 weeks of cuprizone treatment, and local field potential (LFP) recordings performed 10 hr post diazepam injection. (b) Example time frequency plot before (left) and after diazepam injection (right) showing suppression of interictal epileptiform discharges in cuprizone-treated mice.
Optogenetic activation of myelin-deficient parvalbumin-positive (PV+) interneurons attenuates interictal spikes.
Example video of electrocorticogram (ECoG), local field potential (LFP), and behavioral recordings in a 7-week cuprizone-treated mouse. Raw signals show ECoG from right primary somatosensory cortex (SR) and LFP from the layer 5 region (SR-LFP). Interictal epileptiform discharges (red asterisks) were suppressed during laser-induced optogenetic activation of PV+ interneurons in layer 5.
Tables
Reagent type (species) or resource | Designation | Source or reference | Identifiers | Additional information |
---|---|---|---|---|
Strain, strain background (Mus musculus, male/female) | C57BL6 | Janvier Labs | Cat# 2670020; RRID:MGI:2670020 | |
Strain, strain background (M. musculus, male/female) | B6;129P2-Pvalbtm1(cre)Arbr/J | Jackson Laboratory | Stock no: 008069;RRID:IMSR_JAX:008069 | |
Strain, strain background (M. musculus, male/female) | B6;129S6-Gt(ROSA)26Sortm14(CAG-tdTomato)Hze/J | Jackson Laboratory | Stock no: 007908;RRID:IMSR_JAX:007908 | |
Strain, strain background (M. musculus, male/female) | C3Fe.SWV-Mbpshi/J | Jackson Laboratory | Stock no: 001428;RRID:IMSR_JAX:001428 | |
Transfected construct(M. musculus) | pAAV-EF1a-double- floxed-hChR2(H134)-EYFP-WPRE-HGHpA | Addgene.org | #20298;RRID:Addgene_20298 | |
Antibody | Rabbit monoclonal, anti-MBP | Santa Cruz | Cat# sc-13564; RRID:AB_675707 | (1:300) |
Antibody | Mouse monoclonal, anti-PV | Swant | Cat# 235;RRID:AB_10000343 | (1:1000) |
Antibody | Rabbit polyclonal, anti-syt2 | Synaptic Systems | Cat# 105223; RRID:AB_10894084 | (1:500) |
Antibody | Rabbit polyclonal, anti-ßIV-spectrin | M.Ransband (BCM) | N/A | (1:200) |
Peptide, recombinant protein | Alexa 488-streptavidin | Thermo Fisher | Cat# S32354;RRID:AB_2315383 | (1:500) |
Peptide, recombinant protein | Alexa 633-streptavidin | Thermo Fisher | Cat# S21375;RRID:AB_2313500 | (1:500) |
Chemical compound, drug | Bis(cyclohexanone) oxaldihydrazone | Merck | Cat# C9012 | |
Chemical compound, drug | Diazepam | CentrafarmNederland B.V | Cat# RVG56691 | |
Chemical compound, drug | Biocytin | Sigma | Cat# B4261 | |
Chemical compound, drug | D-AP5 | HelloBio | Cat# 0225 | |
Chemical compound, drug | Gabazine | Sigma | Cat# S106 | |
Chemical compound, drug | CNQX | HelloBio | Cat# HB0205 | |
Chemical compound, drug | Tetrodotoxin (TTX) citrate | Tocris | Cat# 1069 | |
Software, algorithm | Neurolucida 360Neurolucida Explorer | MBF Bioscience | V2018.02;RRID:SCR_001775 | |
Software, algorithm | Synaptic puncta quantification | FIJI (ImageJ) | V2.0, V.2.0.0-rc- 65/1.5w;RRID:SCR_002285 | |
Software, algorithm | Neuroarchiver tool | Open Source Instruments; Dubey et al., 2018 | LWDAQ_8.5.29 | |
Software, algorithm | Igor pro 8 | WaveMetrics | V8.04RRID:SCR_000325 | |
Software, algorithm | AxoGraph | AxoGraph | RRID:SCR_014284 | |
Software, algorithm | GraphPad | Prism 8 and 9 | RRID:SCR_002798 | |
Software, algorithm | SP8 X (DM6000 CFS) | Leica application Suite | AF v3.2.1.9702;RRID:SCR_013673 | |
Other | Electrode (90% Pt, 10% Ir) | Science Products | Cat# 101R-5T | |
Other | Optical fiber | Thorlabs | Cat# FP200URT | |
Other | Ceramic ferrule | Thorlabs | Cat# CFLC230-10 | |
Other | Fiber coupled blue laser | Shanghai Laser & Optics Co. | Cat# 473 nm, DPSS Laser with fiber coupled (T3) | |
Other | Cyclops LED driver | Open-ephys.org | Cat# Cyclops LED driver 3.7 | |
Other | Patch-clamp amplifier | Dagan Corporates | BVC-700A | |
Other | Patch-clamp amplifier | Molecular device | Axon Axopatch 200B | |
Other | In vivo Multi Channel Systems (Portable-ME- systems) amplifier | Multi Channel Systems | ME16-FAO-uPA |
Additional files
-
Transparent reporting form
- https://cdn.elifesciences.org/articles/73827/elife-73827-transrepform1-v2.docx
-
Source data 1
Data and statistical analyses of all figures.
- https://cdn.elifesciences.org/articles/73827/elife-73827-data1-v2.xlsx