Frequency- and spike-timing-dependent mitochondrial Ca2+ signaling regulates the metabolic rate and synaptic efficacy in cortical neurons
Figures
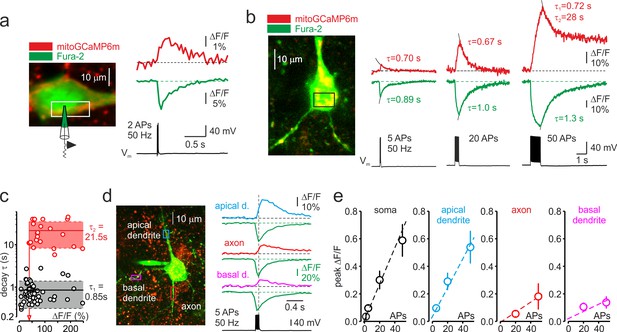
Trains of action potentials elicit mitochondrial Ca2+ elevations in soma and processes of L5 pyramidal neurons.
(a) Changes in mitoGCaMP6m and Fura-2 fluorescence elicited by a train of two APs in a representative L5 pyramidal cell. Left, The image obtained by merging two optical sections through part of a L5 neuron at excitation wavelengths of 760 and 960 nm, eliciting the Fura-2 and mitoGCaMP6m fluorescence, respectively. Right, Somatic mitoGCaMP6m (red) and Fura-2 (green) ΔF/F transients elicited by a train of two APs. MitoGCaMP6m trace (red) is an ensemble average of 16 consecutive sweeps. (b) Small-amplitude mitoGCaMP6m transients decay rapidly. Left, Localization of the mitoGCaMP6m labeled mitochondria in a representative L5 neuron. The image was obtained by merging the fourteen, 1 µm thick optical sections at excitation wavelengths of 760 and 960 nm, eliciting the Fura-2 and mitoGCaMP6m fluorescence, respectively. The yellow color indicates colocalization of the Fura-2 (green) and mitoGCaMP6m (red) fluorescence, revealing the position of the mitochondria. The rectangle indicates the region of interest from which the optical sweeps were obtained. Right, somatic mitoGCaMP6m (red) and Fura-2 (green) transients elicited by 5, 20, and 50 APs in a cell shown on the left. Black lines are the best single or double exponential fits of the decay. Notice that after the relatively small [Ca2+]m elevations evoked by five or 20 APs, the Ca2+ clearance is rapid and follows the single exponential time course (τ ~ 0.7 s). The decay of larger transients evoked by 50 APs is biexponential (τ1 of ~0.7 s and τ2 of ~28 s). (c) Decay time course of mitochondrial Ca2+ transients depends on their amplitude. Each dot represents a decay time constant of the mitoGCaMP6m transient (τ1 for monoexponential, τ1 and τ2 for bi-exponential decays) obtained in recordings from 42 neurons and plotted against its peak amplitude. Continuous lines are mean τ1 (n = 55, black) and τ2 (n = 23, red); dashed lines represent standard deviation from the mean. Arrow represents the amplitude of the smallest mitoGCaMP6m transient (ΔF/F = 44 %), which decayed biexponentially. (d) The amplitude of spike-evoked mitoGCaMP6m fluorescence transients varies between different neuronal processes. Left, Localization of the mitoGCaMP6m labeled mitochondria in a representative L5 pyramidal neuron. The rectangles indicate the regions within the apical dendrite (cyan), basal dendrite (magenta), and axon initial segment (red) from which fluorescence measurements were obtained. Right, Mitochondrial and cytosolic (green) Ca2+ transients elicited by a train of 5 APs at 50 Hz in different neuronal compartments. (e) Mean peak ΔF/F of the mitoGCaMP6m transients as a function of the number of action potentials in soma, apical, and basal dendrites, and axon initial segment. Shown are mean values ± SE (n = 5–29).
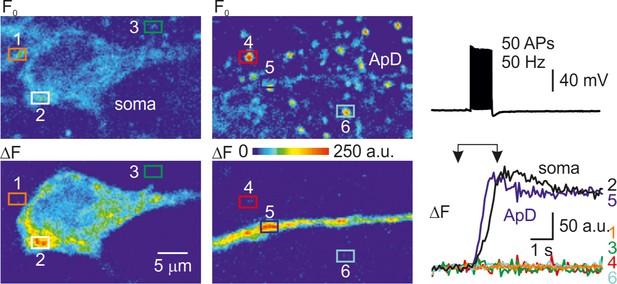
Ca2+ elevations in the mitochondria of the electrically active neurons.
Left, Pseudocolor maps of the mitoGCaMP6m resting fluorescence (Fo, top) and of the change in fluorescence elicited by a train of 50 APs at 50 Hz (ΔF, bottom) between the times marked by the arrowheads in the right panel. The rectangles 1–6 indicate the regions of interest from which fluorescence measurements were obtained. ROIs 2 and 5 contain a single mitochondrion within the soma and apical dendrite, respectively, of the electrically active, patched neuron. ROIs 1,3,4,6 contain mitochondria belonging to the nearby, electrically inactive cells. Right, Action potentials elicited by a train of current pulses injected via the somatic whole-cell pipette only trigger Ca2+ elevation in the mitochondria of the electrically active neuron (ROI 2, 5).
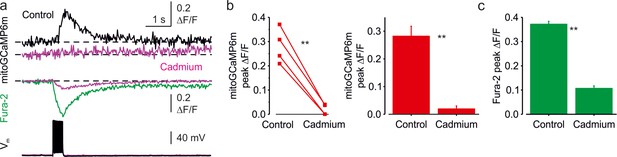
Blockade of voltage-gated Ca2+ channels abolishes spike-evoked mitochondrial Ca2+ transients.
(a) Somatic mitoGCaMP6m (top) and Fura-2 (bottom) transients elicited by a train of 20 APs before (black and green respectively) and, 20 minutes after (magenta) bath application of Cadmium (200 µM). (b) Cadmium application blocks mitochondrial Ca2+ transients. Left, Peak amplitude mitoGCaMP6m ΔF/F transients elicited by 20 AP before and after application of Cadmium. Notice that the mitochondrial Ca2+ transients were almost completely abolished when Cadmium is present. A line connects the paired values obtained from the same individual neuron. Right, Mean peak amplitude of mitoGCaMP6m ΔF/F transients in control, after the Cd2+ application (28% ± 4% vs 2 ± 1%, mean ± SE, n = 4, p < 0.01). (c) Cadmium application blocks cytosolic Ca2+ transients. Mean peak amplitude of Fura-2 ΔF/F transients in control, and after the Cd2+ application was significantly suppressed (37% ± 1% vs 11 ± 1%, mean ± SE, n = 3, p < 0.01).
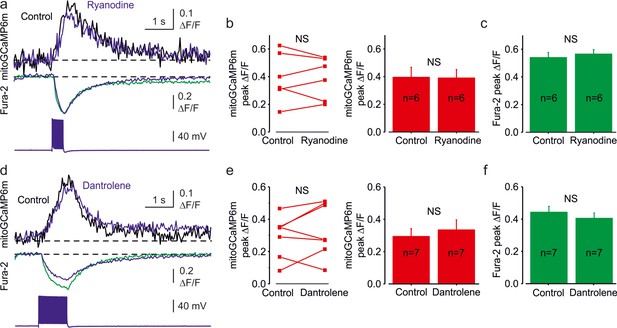
Blockade of the ER Ryanodine receptors has no significant effect on mitochondrial Ca2+ transients.
(a) Somatic mitoGCaMP6m transients elicited by a train of 20 APs before (black) and 20 min after (blue) bath application of high concentration of Ryanodine (100 µM). (b) Blockade of Ryanodine receptor-mediated calcium release from the endoplasmic reticulum did not affect mitochondrial Ca2+ transients. Left, The peak amplitude of mitoGCaMP6m ΔF/F transients before and after application of Ryanodine. A line connects the paired values obtained from the same individual neuron. Right, Mean peak amplitude of mitoGCaMP6m ΔF/F transients in control (39% ± 7%, n = 6) and after the Ryanodine application (39% ± 6%, n = 6, p = 0.88). (c) Mean peak amplitude of Fura-2 ΔF/F transients in control and after the Ryanodine application (53% ± 4% vs 57 ± 3%, respectively, n = 6, p = 0.37). Notice that Ryanodine produces no significant change in the amplitude of the cytosolic Ca2+ transients. (d) Somatic mitoGCaMP6m transients elicited by a train of 50APs before (black) and 20 min after (blue) bath application of Ryanodine receptor blocker, Dantrolene (100 µM). (e) Blockade of Ryanodine receptor-mediated calcium release from the endoplasmic reticulum by Dantrolene did not affect mitochondrial Ca2+ transients. Left, The peak amplitude of mitoGCaMP6m ΔF/F transients before and after application of Dantrolene. A line connects the paired values obtained from the same individual neuron. Right, Mean peak amplitude of mitoGCaMP6m ΔF/F transients in control (29% ± 5%, n = 7) and after the Dantrolene application (33% ± 6%, n = 7, p = 0.33). (f) Mean peak amplitude of Fura-2 ΔF/F transients in control and after the Dantrolene application (44% ± 4% vs 40 ± 3%, respectively, n = 7, p = 0.16). Notice that Dantrolene produces no significant change in the amplitude of the cytosolic Ca2+ transients.
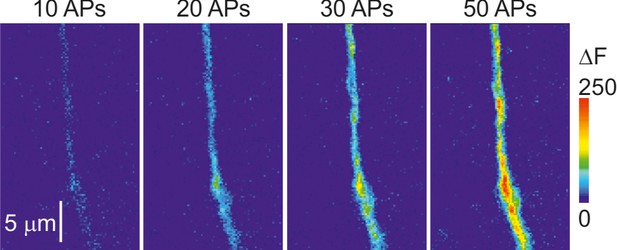
The amplitude of mitoGCaMP6m transients varies as a function of the number of APs.
Shown are pseudocolor maps of change in the mitoGCaMP6m fluorescence in response to trains of 10, 20, 30, and 50 APs at 50 Hz. Fluorescence was measured in the same apical dendrite as in Figure 1—figure supplement 1. Notice that the amplitude of the ΔF transients increases as a function of the number of spikes in the train.
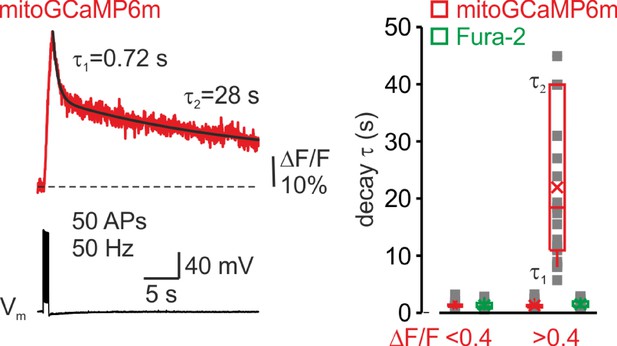
The decay time course of mitochondrial Ca2+ transients varies as a function of their amplitude.
Left, the double exponential time course of mitoGCaMP6m transient elicited by 50 APs during a prolonged (35 s) optical recording. Right, The decay time course of the mitoGCaMP6m and Fura-2 transients. Each dot (gray) represents a decay time constant (τ for monoexponential decays, τ 1 and τ 2 for bi-exponential decays) of transients elicited by 5–50 APs in 42 neurons. Note that the smaller mitoGCaMP6m transients (peak ΔF/F < 0.4, n = 25) decayed mono-exponentially, whereas the decay of the larger mitoGCaMP6m transients (peak ΔF/F > 0.4, n = 22) followed the bi-exponential time course. The decay of Fura-2 transients was rapid and followed the monoexponential time course, independently of their amplitude. Box plots represent the 25–75% interquartile range of the τ, τ1, and τ2 values for mitoGCaMP6m (red) and Fura-2 (green) transients, and the whiskers expand to the 5–95% range. A horizontal line inside the box represents the median of the distribution, and the mean is represented by a cross symbol (X).
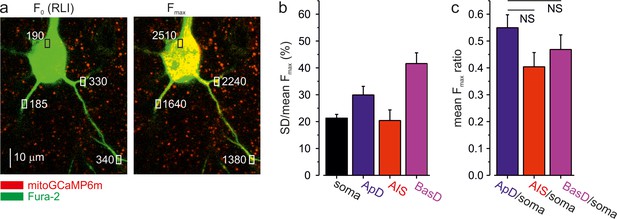
MitoGCaMP6m is homogenously expressed in soma and processes of L5 pyramidal neurons.
(a) Individual mitoGCaMP6m-labeled mitochondria in a representative voltage-clamped L5 pyramidal neuron at a holding potential of –70 mV and following a one-minute long voltage step +20 mV. The images were obtained by merging the single, 1-µm-thick optical sections at excitation wavelengths of 760 and 960 nm, eliciting the Fura-2 (green) and mitoGCaMP6m (red) fluorescence, respectively. An increase in yellow color intensity following the prolonged voltage step reflects an increased mitoGCaMP6m fluorescence. Rectangles indicate the regions within the thin basal dendrite and somata, from which fluorescence measurements (white numbers) were obtained. Notice that the prolonged depolarization elicited a 4–12 times increase in mitoGCaMP6m fluorescence in all neuronal compartments. (b) Variance analysis of the maximal mitoGCaMP6m fluorescence (Fmax) reveals little difference in mitoGCaMP6m expression in the individual mitochondria in the soma and neuronal processes. Fmax values were measured from 5 to 70 individual mitochondria in soma, apical and basal dendrites and the AIS of 14 neurons following prolonged depolarizing voltage step. The mean SD/mean of Fmax was 21% ± 1% for somatic mitochondria, 30% ± 3% in apical dendrites, 20% ± 4% in AIS, and 42% ± 4% in basal dendrites. The relatively narrow Fmax distributions reflect homogeneity in mitoGCaMP6m expression within the mitochondrial population. (c) Mean ratio of Fmax in the neuronal process vs. soma. The mean Fmax ratio was 0.55 ± 0.05 for mitochondria in apical dendrites vs. soma, 0.40 ± 0.05 for mitochondria in AIS vs. soma, 0.47 ± 0.05 for mitochondria in basal dendrites vs. soma (mean ± SE, n = 14 neurons).
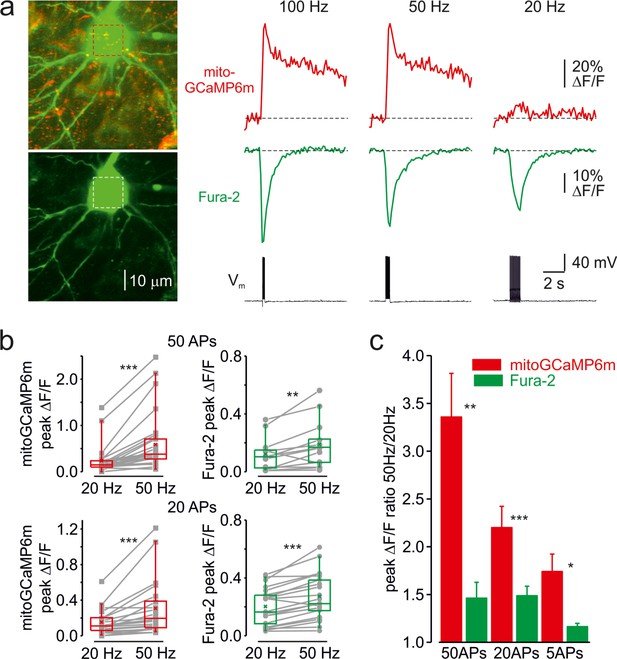
Frequency-dependent amplification of the mitochondrial Ca2+ elevations.
(a) Cytosolic and mitochondrial Ca2+ elevations elicited at the soma of a representative pyramidal neuron by trains of 20 APs at 100, 50, and 20 Hz. Left, The top image is mitoGCaMP6m labeled mitochondrial map of a representative L5 pyramidal neuron, obtained by merging the Fura-2 and mitoGCaMP6m fluorescence (for detail, see Figure 1). The bottom image is the maximum intensity Z-projection representing the Fura-2 fluorescence only. Right, The mitoGCaMP6m (red) and Fura-2 (green) ΔF/F transients elicited at the soma by the spike trains at indicated frequency. Notice that, at 20 Hz, the train of spikes produced almost no mitochondrial Ca2+ elevation, while the amplitude of the cytosolic Ca2+ transient changed little as a function of spike frequency. (b) Peak amplitude of mitoGCaMP6m (red) and Fura-2 (green) ΔF/F transients elicited by a train of 50 (top) or 20 (bottom) APs at a frequency of 20 and 50 Hz. The gray lines connect the paired values obtained from the same individual neuron at two firing frequencies. Box plots represent the 25–75% interquartile range, and the whiskers expand to the 5–95% range. A horizontal line inside the box represents the median of the distribution, and the mean is represented by a cross symbol (X). (c) Mean ratio of peak amplitudes of mitoGCaMP6m (red) and Fura-2 (green) ΔF/F transients elicited by trains of 50, 20, and 5 APs at 50 and 20 Hz frequency.
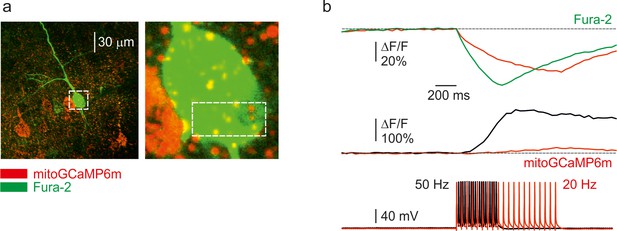
The amplitude of the mitochondrial Ca2+ elevation is proportional to the rate-of-rise of the cytosolic Ca2+ concentration.
(a)Left, an image of a representative L5 pyramidal neuron, obtained by merging the Fura-2 and mitoGCaMP6m fluorescence (for detail, see Figure 1). Right, inset: higher magnification image, corresponding to the rectangle in the left panel. The rectangle indicates the region from which the fluorescence measurements were obtained. (b) ΔF/F transients elicited by a train of 20AP at 20 Hz (red) and 50 Hz (black and green). Cytosolic (top) and mitochondrial (bottom) Ca2+ transients. Notice the slower time course of the cytosolic calcium transient in response to a 20 Hz spike train. The slower rise, and not the difference in [Ca2+]i level, correlates with the mitochondrial calcium transient amplitude.
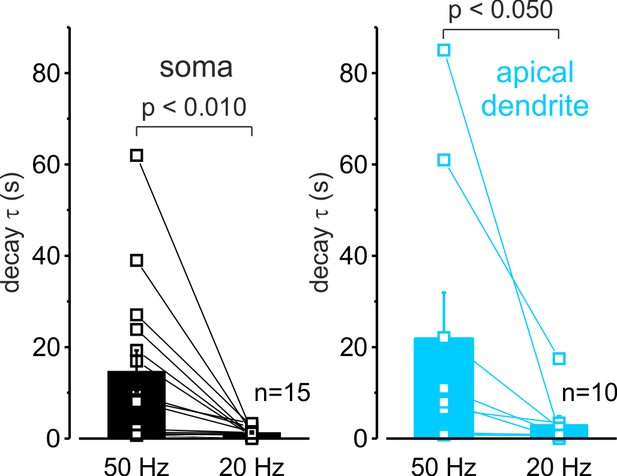
High-frequency firing elicits slowly decaying mitochondrial Ca2+ transients in soma and dendrites of L5 pyramidal neurons.
The decay time constant of somatic (left, black) and dendritic (right, cyan) mitoGCaMP6m transients elicited by trains of 50 APs at a frequency of 20 and 50 Hz. The lines connect paired values obtained from the same neuron at two firing frequencies. The bars represent the mean values ± SEM.
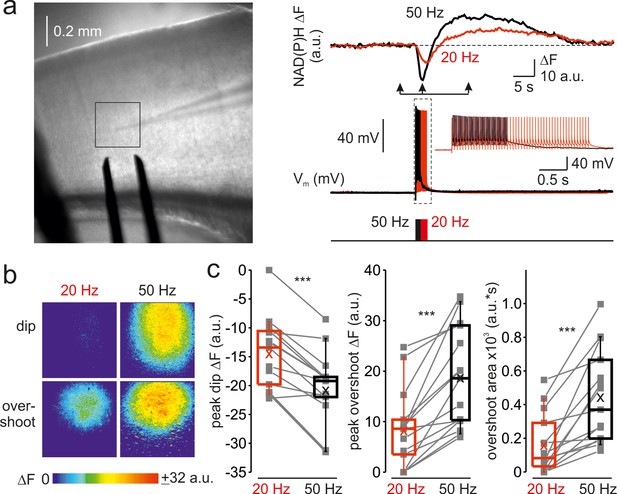
Frequency-dependent amplification of spike elicited changes in mitochondrial NAD(P)H auto-fluorescence.
(a) In a representative cortical slice, changes in NAD(P)H fluorescence in response to extracellular stimuli trains depend on stimulation frequency. Left, DIC image of a coronal slice during the electrical and optical recording. The rectangle indicates the region from which the auto-fluorescence measurements were obtained. The stimuli were delivered via the bipolar electrode placed on the white-gray matter border, and the whole-cell recording was obtained from an L5 neuron within the same cortical column. Right, The membrane potential and optical traces evoked by trains of 50 just suprathreshold stimuli at 50 Hz (black) and 20 Hz (red). Notice that both dip and overshoot of the NAD(P)H signals are more prominent at 50 Hz. Inset: Stimuli intensity was carefully adjusted to elicit only a single AP per stimulus. (b) The amplitude of NAD(P)H signals depends on stimulation frequency, whereas their spatial extent does not. Shown are pseudocolor maps of change in the NAD(P)H fluorescence between the times marked by the arrowheads in a. (c) Higher frequency stimulation causes an increase in the magnitude of the dip and of the overshoot of the NAD(P)H signal. Box plots representing the peaks of the dip (left), the peaks of the overshoot (middle), and the area of the overshoot (right) of the NAD(P)H signals evoked by trains of 20 Hz (red) and 50 Hz (black) stimuli. The gray lines connect the paired values obtained from the same cortical regions at two firing frequencies (n = 15 ROIs, ten cortical slices, three mice). Box plots represent the 25–75% interquartile range, and the whiskers expand to the 5–95% range. A horizontal line inside the box represents the median of the distribution, and the mean is represented by a cross symbol (X).
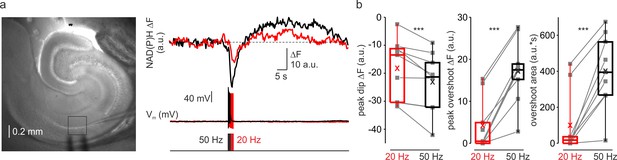
Firing frequency dependent amplification of NAD(P)H signal in the CA1 area of hippocampus.
(a) In a representative hippocampal slice, changes in NAD(P)H fluorescence in response to extracellular stimuli trains depend on stimulation frequency. Left, DIC image of a hippocampal slice during the electrical and optical recording. The rectangle indicates the region from which the auto-fluorescence measurements were obtained. The stimuli were delivered via the bipolar electrode placed in Stratum oriens, and the whole-cell recording was obtained from a nearby CA1 pyramidal neuron. Right, The membrane potential and optical traces evoked by trains of 50 just suprathreshold stimuli at 50 Hz (black) and 20 Hz (red). Notice that both dip and overshoot of the NAD(P)H signals are more prominent at 50 Hz. (b) Higher frequency stimulation causes an increase in the magnitude of the dip and of the overshoot of the NAD(P)H signal. Box plots representing the peaks of the dip (left, –18 ± 4 and –23 ± 4 a.u. for 20 and 50 Hz, respectively, n = 9, p < 0.005), the peaks of the overshoot (middle, 4 ± 2 and 17 ± 3 a.u. for 20 and 50 Hz, respectively, n = 9, p < 0.001), and the area of the overshoot (right, 100 ± 59 and 401 ± 69 a.u.*s for 20 and 50 Hz, respectively, n = 9, p < 0.001) of the NAD(P)H signals. The gray lines connect the paired values obtained from the same regions at two firing frequencies. Box plots represent the 25–75% interquartile range, and the whiskers expand to the 5–95% range. A horizontal line inside the box represents the median of the distribution, and the mean is represented by a cross symbol (X).
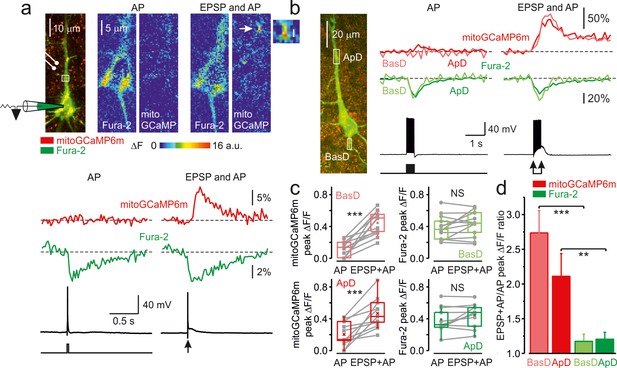
The coincidence of postsynaptic action potential and EPSP induces localized mitochondrial Ca2+ elevations in the dendrites.
(a) Action potential elicited by a single, just suprathreshold synaptic stimulus induces a large, spatially restricted increase in dendritic mitoGCaMP6m fluorescence, whereas action potential evoked by a 5 ms current pulse (600 pA, cell body injection) had no such effect. Top, Left: Mitochondrial map in a representative L5 pyramidal neuron, obtained by merging the Fura-2 and mitoGCaMP6m fluorescence (for detail, see Figure 1). The rectangle indicates the region within the apical dendrite from which fluorescence measurements were obtained. Right: Pseudocolor maps of change in the mitoGCaMP6m and Fura-2 fluorescence in response to a synaptically and current pulse evoked AP. Bottom, The mitoGCaMP6m (red) and Fura-2 (green) ΔF/F transients measured from regions of interest as indicated in the upper panel and the somatic membrane potential trace. Arrow indicates a hotspot at which the synaptic stimulus elicited a mitochondrial Ca2+ transient. The transients are ensemble averages of 50 sweeps. (b) Action potentials elicited by a train of synaptic stimuli produce larger mitoGCaMP6m signals in the dendrites than APs elicited by the injection of a train of current pulses. Left: Mitochondria map in a representative L5 pyramidal neuron, obtained by merging the Fura-2 and mitoGCaMP6m fluorescence. The rectangles indicate the regions within the apical and basal dendrites from which fluorescence measurements were obtained. Right, Comparison of ΔF/F mitoGCaMP6m transients elicited in the apical (red) and basal dendrite (rose) by twenty suprathreshold synaptic stimuli at 50 Hz and by twenty brief current pulses delivered to the soma. The green and light green traces are ΔF/F Fura-2 transients in apical and basal dendrites, respectively. The black traces represent somatic membrane potential. (c) Peak amplitude of mitoGCaMP6m and Fura-2 ΔF/F transients elicited in basal (top) and apical dendrites (bottom) by a train of twenty suprathreshold current pulses or synaptic stimuli at a frequency of 50 Hz. The line connects the paired values obtained from the same individual neuron at two stimulation modalities. Box plots represent the 25–75% interquartile range, and the whiskers expand to the 5–95% range. A horizontal line inside the box represents the median of the distribution, and the mean is represented by a cross symbol (X). (d) Mean ratio of peak amplitudes of mitoGCaMP6m (rose, red) and Fura-2 (light green, green) ΔF/F transients elicited in dendrites by suprathreshold synaptic stimuli (EPSP + AP) and current pulses (AP). Data obtained from 8 to 13 individual neurons.
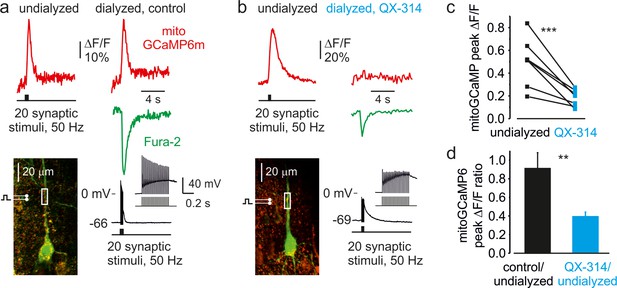
EPSPs alone cause no significant Ca2+ elevation in dendritic mitochondria.
(a) MitoGCaMP6m ΔF/F transients (red) in the apical dendrite elicited by a train of 20 synaptic stimuli before (left) and 10 min after (right) break-in to the whole-cell configuration with a pipette filled with control solution. Note that the mitochondrial Ca2+ transients are almost unaffected by intracellular dialysis. The green trace represents the Fura-2 ΔF/F transient obtained after the establishment of whole-cell recording. Inset: Whole-cell voltage recording of a sequence of EPSPs and orthodromic APs elicited by the train of stimuli. (b) MitoGCaMP6m ΔF/F transients (red) in the apical dendrite elicited by a train of 20 synaptic stimuli before (left) and 10 min after (right) break-in to the whole-cell configuration with a pipette filled with QX-314 (100 µM) containing solution. QX-314, a Na+ channel blocker, was added to block postsynaptic AP generation. Note that, in the presence of QX-314, a train of large-amplitude EPSPs (see inset) was sufficient to produce a cytosolic Ca2+ elevation (green trace). However, the amplitude of mitochondrial Ca2+ transients was significantly reduced. (c) The peak amplitude of the dendritic mitoGCaMP6m ΔF/F transients elicited by 20 synaptic stimuli before (black) and after (blue) intracellular application of QX-314. Notice a significant decrease in amplitude of mitochondrial Ca2+ signals when AP generation is blocked (from 50 ± 8%–18 ± 3%, n = 7, p < 0.005). A line connects the paired values obtained from the same individual neuron. (d) Mean ratio of peak amplitudes of synaptically elicited, dendritic mitoGCaMP6m ΔF/F transients after the dialysis with either control (black, n = 8) or QX-314-containing intracellular solution (blue, n = 7) to those before the establishment of whole-cell recording. Notice that while the dialysis with control solution elicits little change in the amplitude of mitochondrial Ca2+ signals, blockade of postsynaptic AP causes a significant decrease in the ratio (p < 0.005).
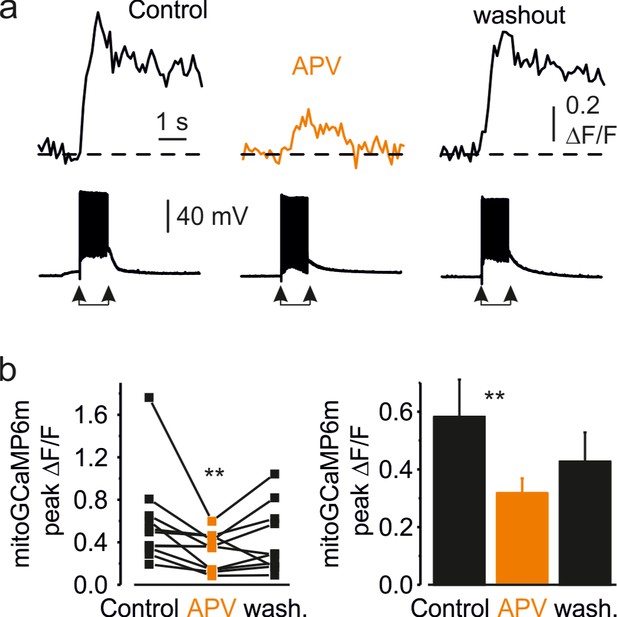
Availability of synaptic NMDA receptors is required for induction of dendritic mitochondrial Ca2+ elevation.
(a) MitoGCaMP6m ΔF/F transients in the apical dendrite of a representative L5 neuron before, after bath application of APV (50 µM), and following the washout. The bottom traces represent somatic membrane potential. Arrows mark the beginning and end of the synaptic stimuli train. (b) Blockade of NMDA receptors causes a reduction in amplitude of the synaptically evoked mitochondrial Ca2+ transients in dendrites. Left, The peak amplitude of the dendritic mitoGCaMP6m ΔF/F transients elicited by 20 synaptic stimuli before, during APV application, and upon the washout. Notice a significant decrease in the transients' amplitude (n = 10, p < 0.01) when APV is present. A line connects the paired values obtained from the same individual neuron. Right, Mean peak amplitude of mitoGCaMP6m ΔF/F transients in control, after the APV application and after the washout (58% ± 13%, 32% ± 5% and 43% ± 10%, respectively, n = 10).