Control of parallel hippocampal output pathways by amygdalar long-range inhibition
Figures
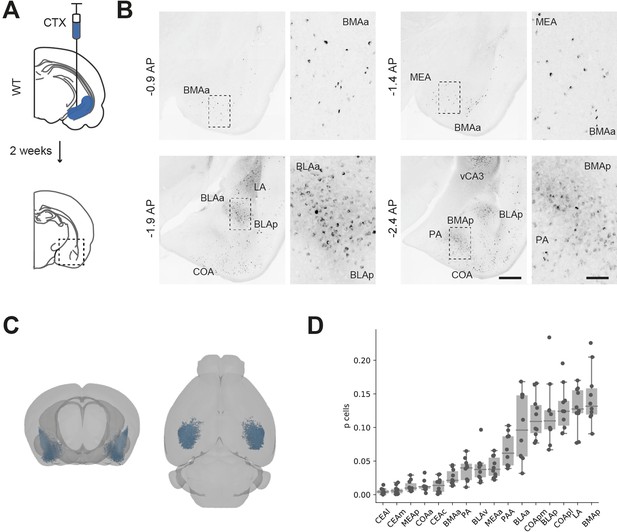
Distribution of basal amygdala (BA) input to ventral hippocampus (vH).
(A) Schematic of experiment. CTXβ was injected into vH, 2 weeks later coronal slices of BA were examined for retrogradely labelled neurons. (B) Example slices showing widespread labelling throughout numerous BA nuclei. Scale bar = 500 μm, 100 μm (zoom). Images are stitched from tiled scans. (C) Whole-brain distribution of labelled BA neurons. (D) Summary showing proportion of labelled BA cells in each nuclei. CEA, central amygdala; MEA, medial amygdala; COA, cortical amygdala; BMA, basomedial amygdala; PA, posterior amygdala; BLA, basolateral amygdala; LA, lateral amygdala; l, lateral; m, medial; a, anterior; p, posterior.
-
Figure 1—source data 1
Source data for Figure 1.
- https://cdn.elifesciences.org/articles/74758/elife-74758-fig1-data1-v2.xlsx
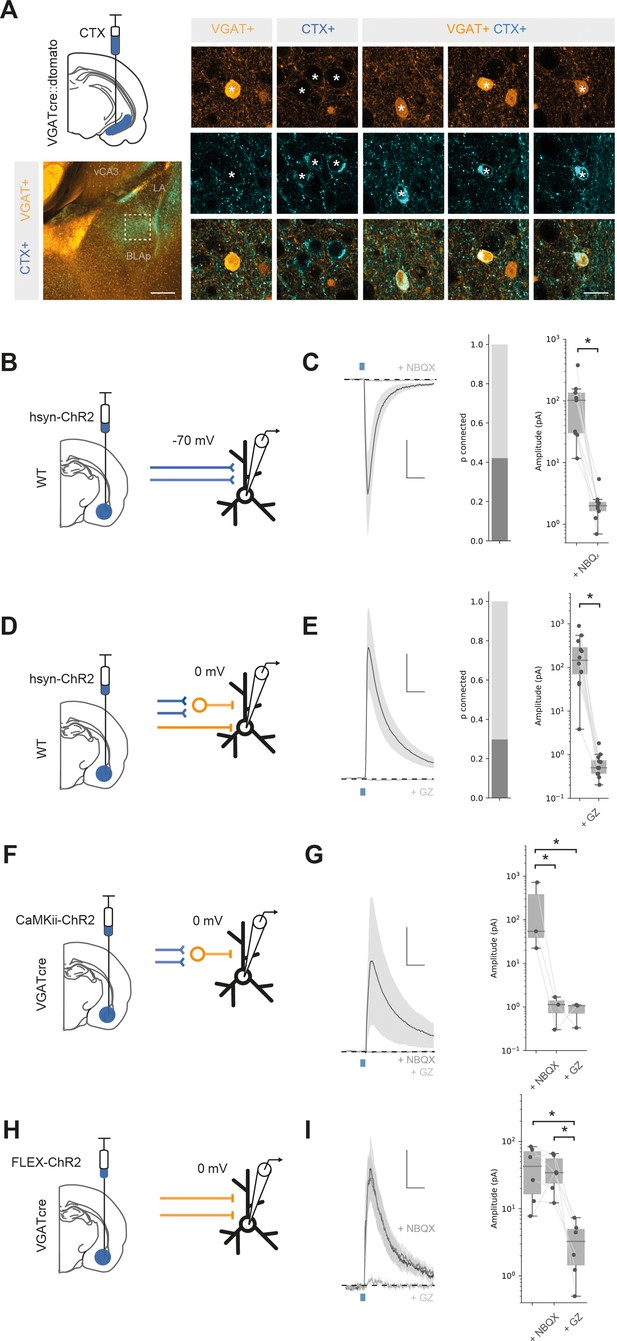
Basal amygdala (BA) input to ventral hippocampus (vH) is both excitatory and inhibitory.
(A) CTXβ injection in vH in a vGAT::cre::dtomato mouse line reveals inhibitory neurons (vGAT+), putative excitatory neurons that project to vH (CTX+) and inhibitory neurons that project to vH (vGAT+ CTX+). Example neurons from boxed region on left. Scale bar = 300 μm (left), 20 μm (right). (B) Schematic showing experimental setup. ChR2 was expressed using the pan-neuronal synapsin promoter using an adeno-associated virus (AAV) injection in BA. After allowing for expression, whole-cell recordings were performed in voltage clamp at – 70 mV in vH. (C) Brief pulses of blue light evoke excitatory currents that are blocked by the AMPA receptor antagonist NBQX. Left: average current trace pre- and post-NBQX. Middle: proportion of recorded cells connected (with time-locked response to light). Right: amplitude before and after NBQX. Note log scale. NBQX blocks excitatory currents evoked by BA input. Scale bar = 50 pA, 10 ms. (D, E) As (B, C) but for voltage clamp at 0 mV before and after the GABA receptor antagonist gabazine. Gabazine blocks inhibitory currents evoked by BA input. Scale bar = 50 pA, 10 ms. (F) Feedforward inhibition isolated using ChR2 expression under the CaMKii promoter. (G) Brief pulses of blue light evoked inhibitory currents at 0 mV that are blocked by the AMPA receptor antagonist NBQX. Left: average current trace pre- and post-NBQX and GZ. Right: amplitude before and after NBQX and GZ. Note log scale. NBQX blocks inhibitory currents evoked by CaMKii BA input, indicating it is solely feedforward. Scale bar = 50 pA, 10 ms. (H, I) As for (F, G) but direct inhibitory input isolated using ChR2 expression only in vGAT + BA neurons. NBQX has no effect on direct inhibitory connection, while it is blocked by GZ, indicating that it is a direct, long-range inhibitory connection. Scale bar = 15 pA, 10 ms.
-
Figure 2—source data 1
Source data for Figure 2.
- https://cdn.elifesciences.org/articles/74758/elife-74758-fig2-data1-v2.xlsx
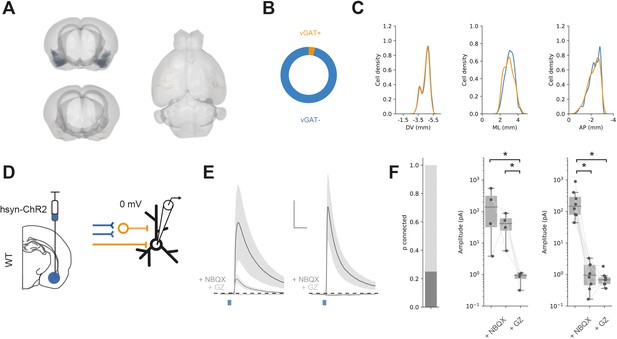
Feedforward and direct inhibitory input from basal amygdala (BA) to ventral hippocampus (vH).
(A) Whole-brain distribution of labelled excitatory (blue) and inhibitory (orange) BA neurons after injection of CTXβ into vH. (B) Proportion of CTXβ-labelled neurons that are vGAT+ (orange) and vGAT- (blue). (C) Distribution of CTXβ neurons that were vGAT+ (orange) and vGAT- (blue) across three anatomical axes. Note similar overall distribution. (D) Schematic of experiment. Pan-neuronal synapsin promoter expresses ChR2 in both inhibitory and excitatory neurons, allowing assessment of feedforward and direct BA input to vH. (E) Brief pulses of blue light evoked inhibitory currents at 0 mV. Average current traces pre- and post-NBQX and GZ. In a proportion of neurons (left) currents not completely blocked by the AMPA receptor antagonist NBQX, but removed by GABA receptor antagonist gabazine, indicating the presence of direct inhibition. In other recorded neurons, currents were completely blocked by NBQX (right), indicating only feedforward inhibition. Scale bar = 50 pA, 10 ms. (F) Left: proportion of neurons with inhibitory currents that received direct inhibition. Right: amplitude before and after NBQX and GZ in two groups of neurons. Note log scale.
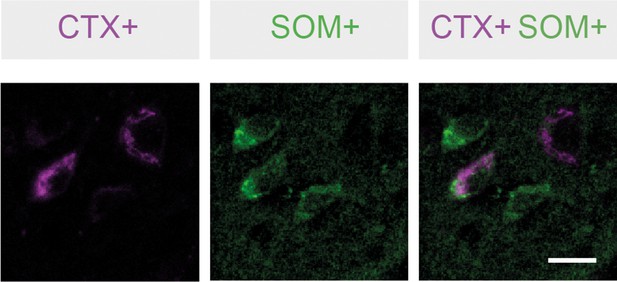
Somatostatin-positive neurons project from basal amygdala (BA) to ventral hippocampus (vH).
Retrogradely labelled CTX+ neurons (magenta) in BA after injection into vH are colocalised with immunofluorescence-detected somatostatin (green). Scale bar = 20 µm.
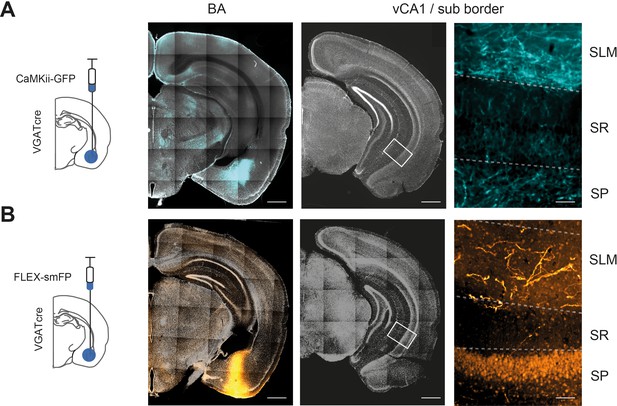
Both CaMKii+ and VGAT+ neurons project from basal amygdala (BA) to ventral hippocampus (vH).
(A) Injection of virus to express GFP under the CamKii promoter restricts expression to excitatory neurons in BA (cyan, left). Right shows zoom of distal CA1 in vH showing axon innervation from BA. Slices counterstained with DAPI (white). Scale bar = 500 µm, 50 µm (zoom). (B) As in (A) but using a virus expressing a cre-dependent tracer smFP in a VGAT mouse to restrict expression to inhibitory BA neurons (orange). Note that the dim cell body labelling in zoom on the right is background staining due to use of a FLAG antibody.
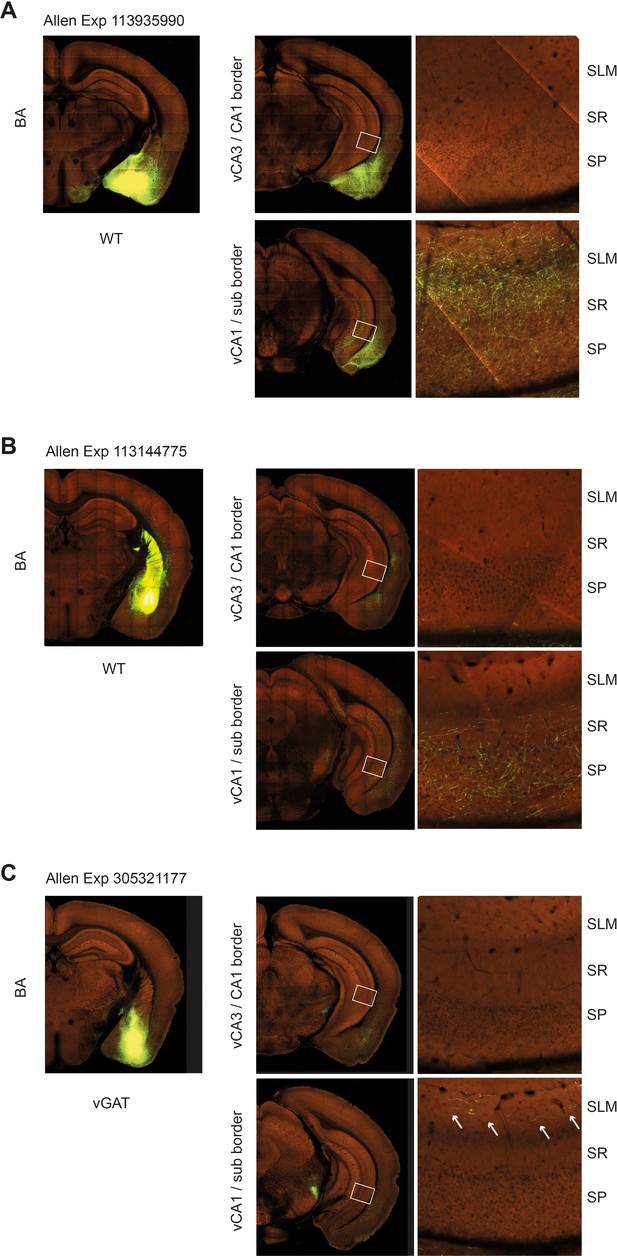
Confirmation of synapsin+ and VGAT+ projection from basal amygdala (BA) to ventral hippocampus (vH) from Allen Brain Connectivity Atlas.
(A) Images of Allen Exp 113935990 injection into BA with GFP expressed under the pan-neuronal synapsin promoter. Note lack of axons in proximal CA1/CA2 border, but dense innervation of distal CA1/subiculum border. (B) Images of Allen Exp 113144775 injection into anterior BA with GFP expressed under the pan-neuronal synapsin promoter. Note lack of axons in proximal CA1/CA2 border, but innervation of distal CA1/subiculum border. Also note lower density innervation due to anterior location of injection, consistent with retrograde tracing in Figure 1. (C) Images of Allen Exp 305321177 injection into BA with cre-dependent GFP expressed in a VGAT mouse to label only inhibitory neurons. Again note lack of inhibitory axons in proximal CA1/CA2 border, but innervation of distal CA1/subiculum border.
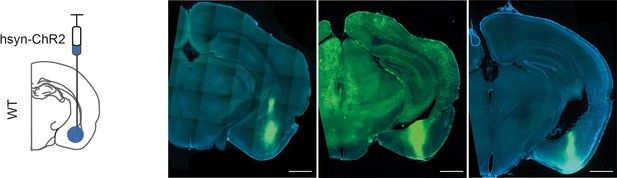
Example injection sites for physiology experiments.
Three example injections of ChR2 under the hSyn promoter into basal amygdala (BA). Scale bar 750 µm.
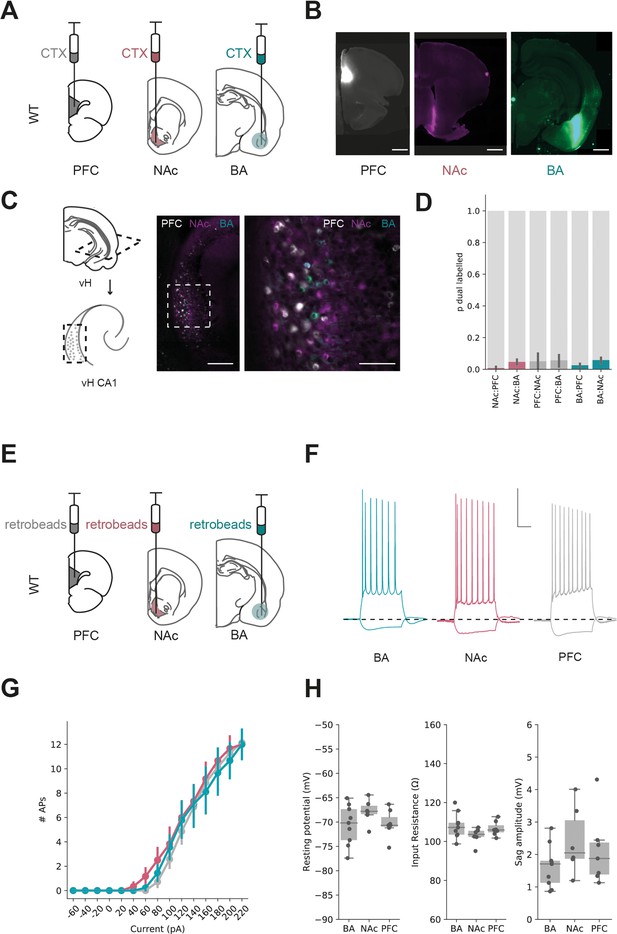
Parallel output populations in ventral CA1/subiculum.
(A) Schematic of experiment, three differently tagged CTXβ tracers were injected into prefrontal cortex (PFC), nucleus accumbens (NAc) and basal amygdala (BA). (B) Example injection sites in each region. Scale bar 1 mm. (C) Horizontal section of CA1/subiculum in ventral hippocampus (vH) showing interspersed but non-overlapping labelling. Scale bars 300 µm (left), 100 µm (right). (D) Proportion of neurons labelled with CTXβ injection in NAc (red), BA (green) or PFC (grey) co-labelled with CTXβ from a different region. Note that there is only a small proportion of dual labelled neurons. (E) Strategy for electrophysiology recordings – projection populations were fluorescently labelled with retrobead injections into downstream projection areas. (F) Examples of positive (+160 pA) and negative (–40 pA) current steps in fluorescently targeted neurons from each population. Scale bar = 30 mV, 100 ms. (G, H) No large differences in input/output curve, resting potential, input resistance or sag amplitude across the three populations.
-
Figure 3—source data 1
Source data for Figure 3.
- https://cdn.elifesciences.org/articles/74758/elife-74758-fig3-data1-v2.xlsx
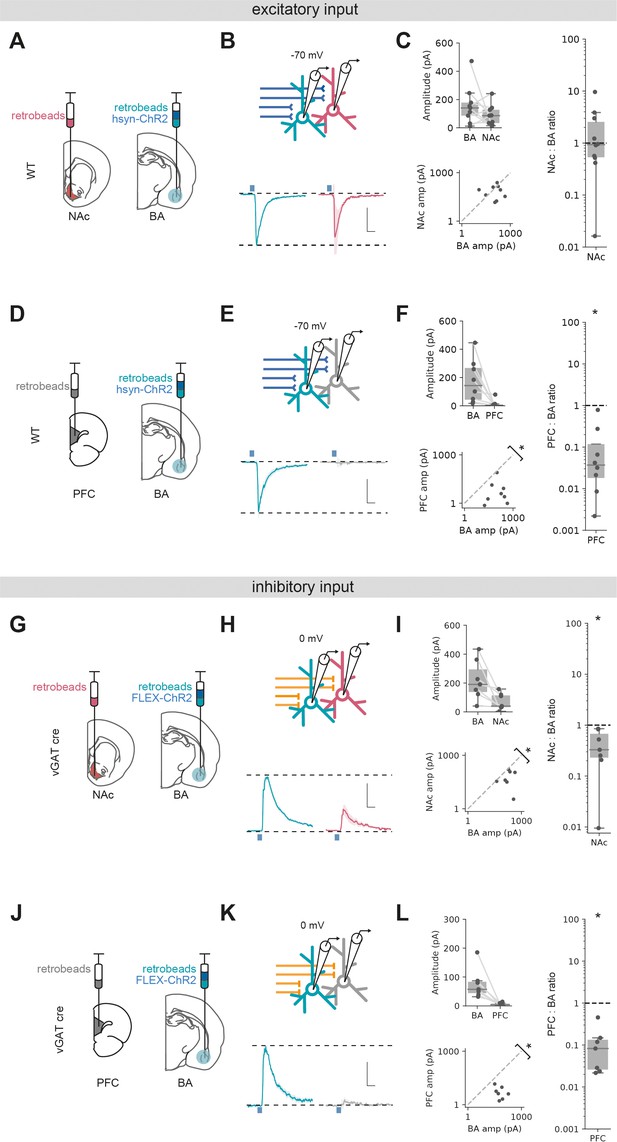
Excitatory and inhibitory basal amygdala (BA) input differentially targets ventral hippocampus (vH) output populations.
(A) Schematic of experiment vHNAc and vHBA neurons was labelled with retrobead injections, and ChR2 was expressed pan-neuronally in BA. (B) Paired, fluorescently targeted recordings from neurons in each pathway and recording of light-evoked currents. Top: recording setup. Bottom: average light-evoked currents in vHBA (green) and vHNAc (red) neurons. Scale bar = 0.5 vHBA response, 10 ms. (C) Summary of amplitude of light-evoked BA input in pairs of vHNAc and vHBA neurons (top). When displayed as a scatter plot (bottom), or as the ratio of vHNAc:vHBA (right), the amplitudes cluster on the line of unity, indicating that these populations share equal input. Note log axis. (D–F) As (A–C) but for pairs of vHBA and vHPFC neurons. Note that when displayed as a scatter and a ratio, both vHPFC:vHBA amplitudes are below the line of unity, indicating that input preferentially innervates vHBA neurons. (G–L) As (A–F) but for inhibitory input from BA isolated by expressing FLEX ChR2 in a vGAT::Cre line. Note that when displayed as a scatter and a ratio, both vHPFC and vHNAc amplitudes are below the line of unity, indicating that inhibitory input preferentially innervates vHBA neurons in both cases. Scale bar = 0.5 vHBA response, 10 ms.
-
Figure 4—source data 1
Source data for Figure 4.
- https://cdn.elifesciences.org/articles/74758/elife-74758-fig4-data1-v2.xlsx
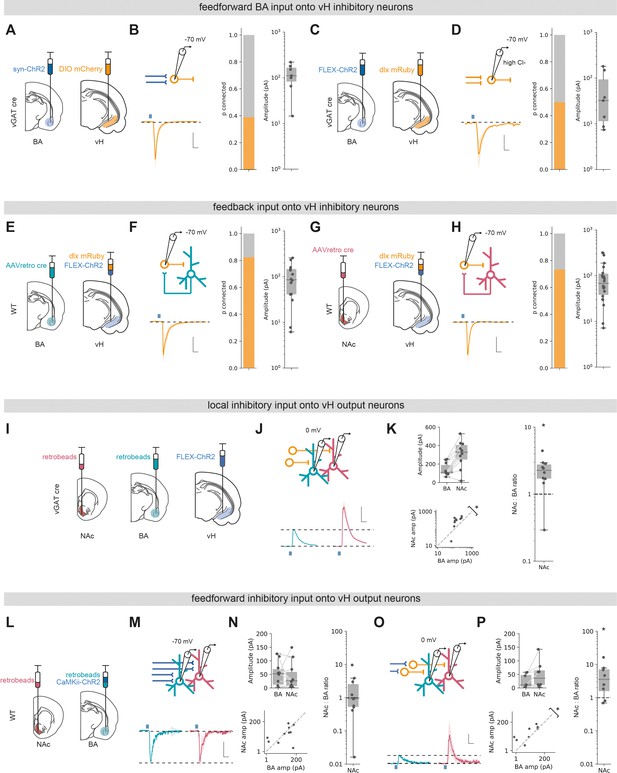
Basal amygdala (BA) input interacts with local inhibitory circuitry that is biased towards vHNAc neurons.
(A) Schematic of experiment. ChR2 was expressed in BA, and DIO mCherry was expressed in ventral hippocampus (vH) in vGAT:cre mice to label local interneurons. (B) Left: recording configuration to record excitatory connectivity at –70 mV (top). Average light-evoked current in interneurons in vH. Scale bar = 50 pA, 10 ms. Right: summary of probability of connection (left) and amplitude of connected currents (right). (C, D) As (A, B) but for inhibitory input isolated using FLEX ChR2 expression in vGAT:cre mice as before. Note that recordings were performed in high Cl-, so inward currents were measured at –70 mV. (E) Experimental setup for investigating feedback connectivity from vHBA neurons. AAVretro was injected into BA, and FLEX ChR2 and dlx-mRuby into vH to allow recordings from dlx+ interneurons, and measurement of light-evoked currents from vHBA activation. (F) Left: recording configuration to record excitatory connectivity at –70 mV (top). Average light-evoked current in dlx+ interneurons in vH. Right: summary of probability of connection (left) and amplitude of connected currents (right). (G, H) As (E, F) but for feedback input from vHNAc neurons. (I) Schematic of experiment, vHNAc and vHBA cells were labelled with injections of retrobeads, while ChR2 was expressed in vH interneurons using FLEX ChR2 in a vGAT::cre mouse. (J) Paired, fluorescently targeted recordings from neurons in each pathway at 0 mV and recording of light-evoked currents. Top: recording setup. Bottom: average light-evoked currents in vHBA (green) and vHNAc (red) neurons. Scale bar = 1 vH-BA response, 10 ms. (K) Summary of amplitude of light-evoked BA input in pairs of vHNAc and vHBA neurons (top). When displayed as a scatter plot (bottom), or as the ratio of vHNAc: vHBA (right), the amplitudes cluster above the line of unity, indicating that local inhibition preferentially innervates vHNAc neurons. Note log axis. (L–N) as (I, J) but for CaMKii input recorded at –70 mV. Note as in Figure 3 that there is equal input onto both populations. Scale bar = 0.5 vHBA response, 10 ms. (O, P) as in (M, N) but recording at 0 mV to isolate feedforward inhibition. Note that the amplitudes cluster above the line of unity, indicating that feedforward inhibition preferentially innervates vHNAc neurons. Scale bar = 1 vH-BA response, 10 ms.
-
Figure 5—source data 1
Source data for Figure 5.
- https://cdn.elifesciences.org/articles/74758/elife-74758-fig5-data1-v2.xlsx
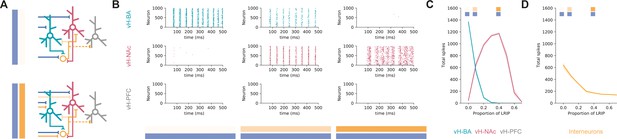
Co-activation of inhibitory and excitatory input switches ventral hippocampus (vH) activity from vHBA to vHNAc.
(A) Schematic of integrate-and-fire model. Three populations of projection neurons (vHNAc, red; vHBA, green; vHPFC, grey) and local interneurons (orange) are innervated by excitatory (blue, top) as well as inhibitory (orange, bottom) basal amygdala (BA) input. Connectivity is defined from results in previous figures. (B) Increasing the proportion of inhibitory relative to excitatory BA input has opposite effects on vHBA and vHNAc spiking. Each graph shows a raster of spiking for each neuron across a 500 ms period. Note high vHBA spiking with no inhibitory input, and high vHNAc spiking with high inhibitory input. vHPFC neurons never fire as they are not innervated by BA and only receive background input. (C) Summary of pyramidal neuron activity. With increasing inhibitory input, activity shifted from vHBA to vHNAc neurons. Markers indicate proportions plotted in (B). (D) Long-range inhibition reduces local interneuron firing, removing preferential feedback inhibition onto vHNAc neurons, allowing them to fire.
-
Figure 6—source data 1
Source data for Figure 6.
- https://cdn.elifesciences.org/articles/74758/elife-74758-fig6-data1-v2.xlsx
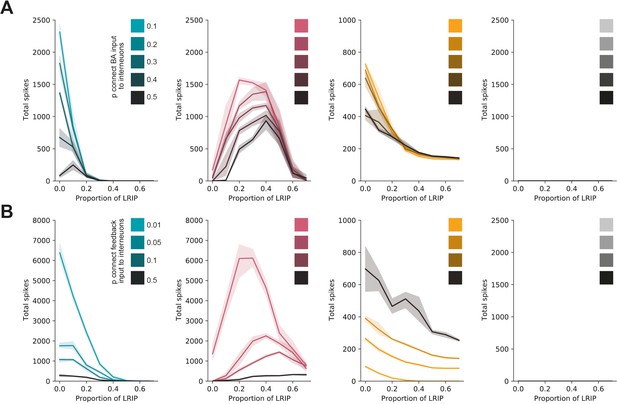
The switch in vHBA and vHNAc activity is robust over a wide range of feedforward and feedback connectivity.
(A) Total spiking of model in vHBA neurons (green), vHNAc neurons (red), vHPFC neurons (grey) and interneurons (orange) with increasing levels of basal amygdala (BA) inhibition, with different levels in feedforward inhibition (darker colours). Note that although absolute firing changes, the switch from vHBA to vHNAc activity with increasing inhibitory input is maintained. (B) As in (A) but for different levels of feedback connectivity. Note that again the switch from vHBA to vHNAc with increasing inhibitory input is maintained.
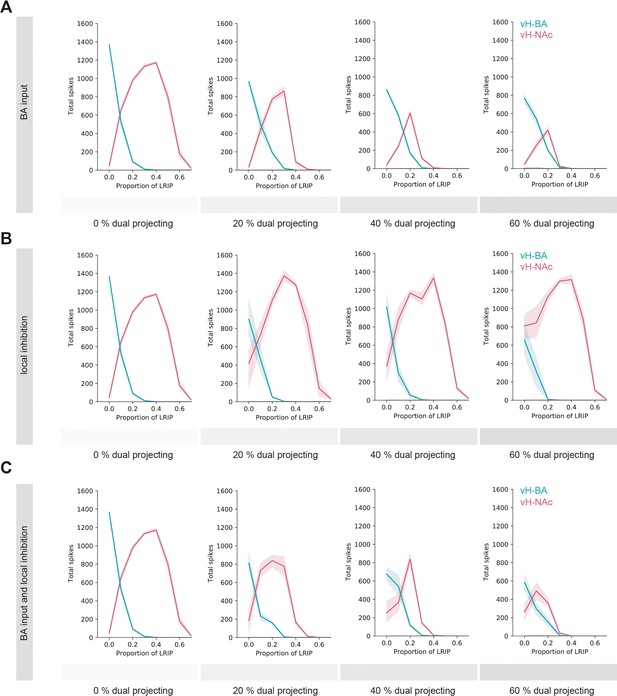
The switch in vHBA and vHNAc activity is robust to collateralisation of output projections.
(A) Multiple iterations of the circuit model were run where the effect of collateralisation was tested by adding increasing proportions of neurons where connectivity with basal amygdala (BA) input was defined by a different downstream projection. (B) As in (A), but local connectivity was altered dependent on downstream projection. (C) As in (A, B), but both BA input and local connectivity were altered dependent on downstream projection. Note that in all three simulations the overall behaviour of the model is maintained, even with large ~40–60% collateralisation.
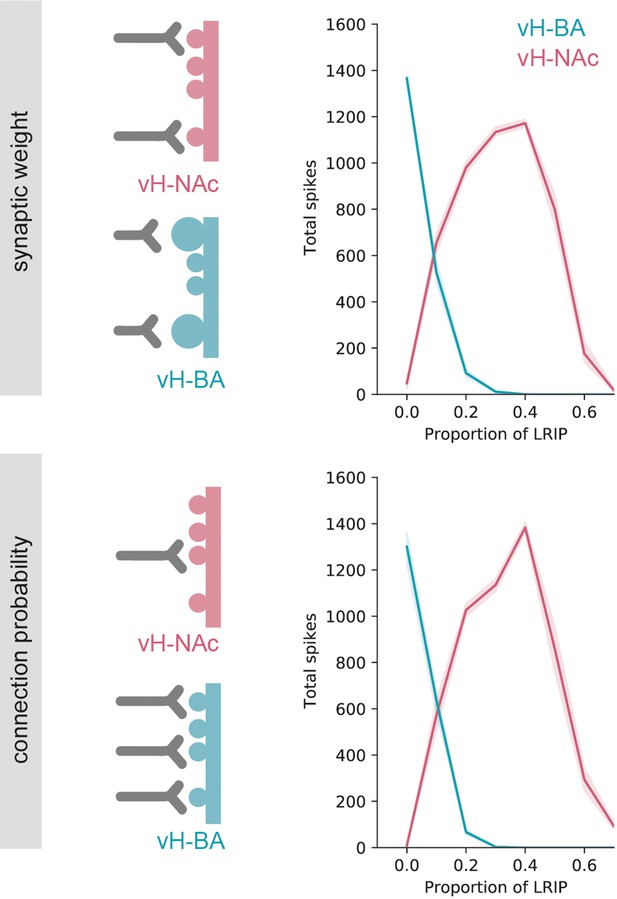
The switch in vHBA and vHNAc activity is robust to postsynaptic specialisation.
Two iterations of the model were run, the first (top, and main figure) where differences in basal amygdala (BA) input were due to differences in the synaptic weight of individual connections onto ventral hippocampus (vH) neurons. The second (bottom) was identical apart from the differences in BA input were due to the probability of BA connection with no change in synaptic weight. Note that both models display similar behaviour.
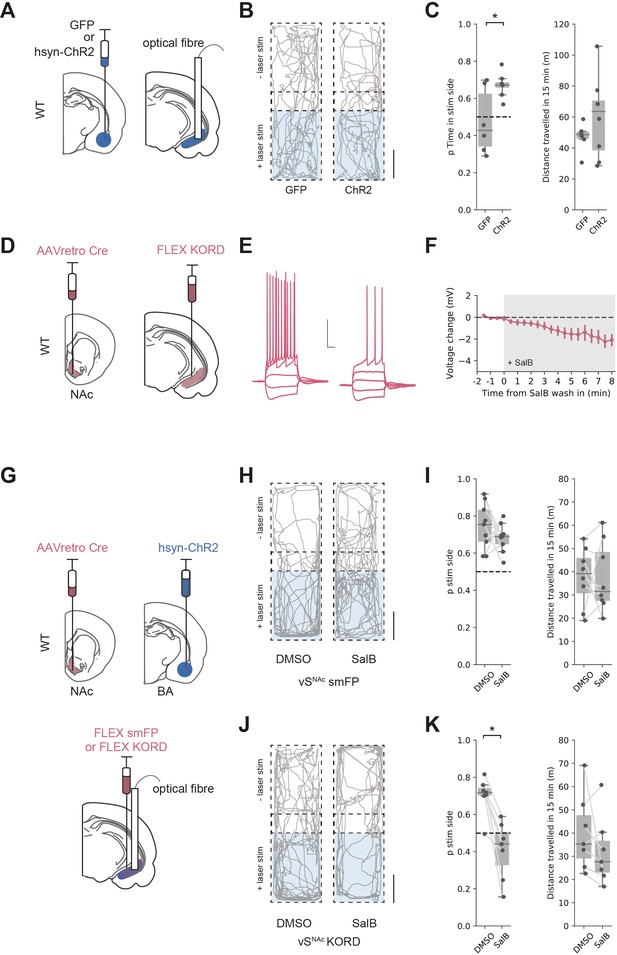
Basal amygdala (BA) input supports real-time place preference (RTPP) dependent on vHNAc neurons.
(A) Schematic of experiment. GFP or pan-neuronal ChR2 were expressed in BA and an optic fibre implanted in ventral hippocampus (vH). (B) RTPP assay. One side of a chamber was paired with 20 Hz blue light stimulation. Example trajectories of GFP (left) and ChR2 (right)-expressing animals over the 15 min RTPP session. Note increased occupancy of light-paired (stim) side in ChR2 animals. Scale bar = 15 cm. (C) Summary of RTPP. Left: proportion of time spent on stim side (left) and total distance travelled (right) in GFP and ChR2 animals. Note consistent preference for stim side in ChR2 animals. (D) Strategy to express KORD in vHNAc neurons. (E, F) Bath application of salvinorin B (SalB) (100 nm) hyperpolarises KORD-expressing vHNAc neurons and reduces AP firing. See Figure 7—figure supplement 1 for full quantification. Scale bar = 30 mV, 100 ms. (G) Schematic of strategy to inhibit vHNAc neurons during BA input-driven RTPP. (H, I) As (B, C) but comparing the effect of either DMSO (vehicle) or SalB (KORD agonist) injections 15 min before testing in control mice. Note consistent RTPP in both conditions indicating no effect of SalB in control mice. (J, K) As (H, I), but in mice expressing KORD in vHNAc neurons. Note loss of RTPP in SalB-injected mice compared to controls.
-
Figure 7—source data 1
Source data for Figure 7.
- https://cdn.elifesciences.org/articles/74758/elife-74758-fig7-data1-v2.xlsx
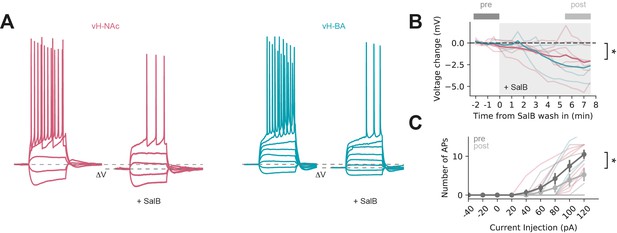
Salvinorin B (SalB) wash in reduces activity of KORD-expressing neurons.
(A) Example traces before and after SalB wash in in vHNAc neuron (red) and vHBA neuron. Note lower resting potential and reduced spiking after SalB wash in. (B) Summary of effect of SalB wash in on resting potential for vHNAc neurons (red) and vHBA neurons. Thin lines are individual experiments, old line is group average. (C) Summary of effect of SalB wash in on current injection-induced action potential firing. Thin lines show individual vHNAc neurons (red) and vH-BA neurons, grey lines show group average.
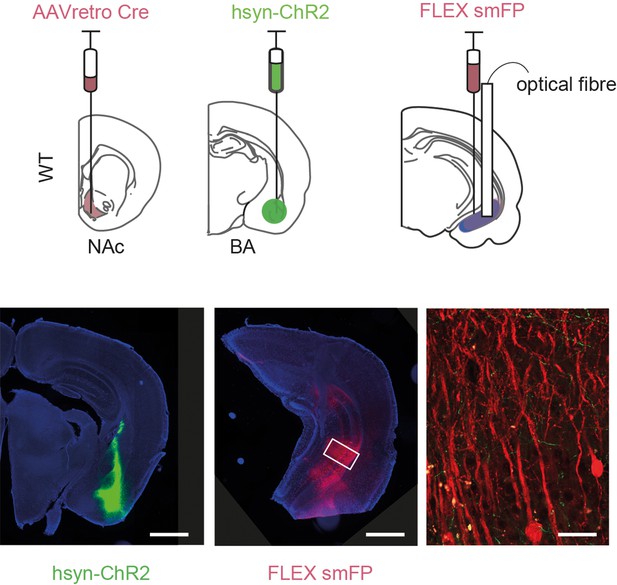
Example injection sites for KORD experiments.
Example injection of ChR2 in basal amygdala (BA) (left), cre-dependent smFP in ventral hippocampus (vH) (middle) and zoom (right) showing BA axons (green) innervating smFP+ neurons (red).
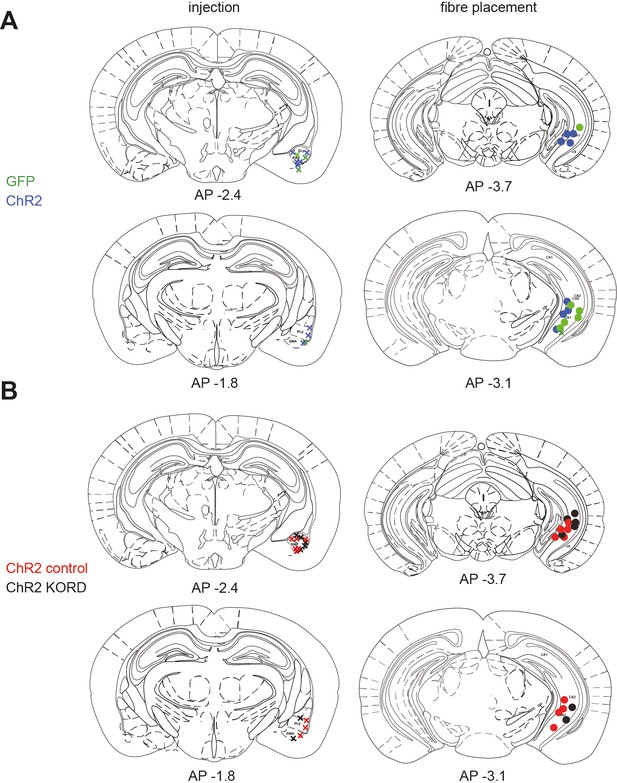
Histology for behavioural experiments in Figure 7.
(A) Histology for experiments in Figure 6A–C. (B) Histology for experiments in Figure 6G–K.
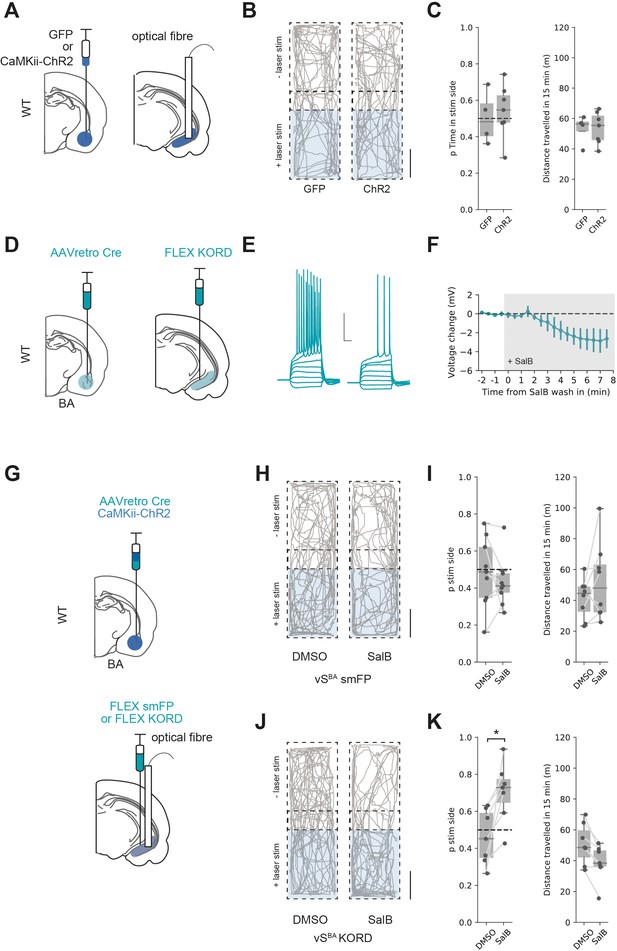
Excitatory basal amygdala (BA) input supports real-time place preference (RTPP) only after inhibition of vHBA neurons.
(A) Schematic of experiment. GFP or excitation-specific CaMKii ChR2 were expressed in BA and an optic fibre implanted in ventral hippocampus (vH). (B) RTPP assay. One side of a chamber was paired with 20 Hz blue light stimulation. Example trajectories of GFP (left) and ChR2 (right)-expressing animals over the 15 min RTPP session. Note lack of preference for light-paired (stim) side in either group. Scale bar = 15 cm. (C) Summary of RTPP. Left: proportion of time spent on stim side (left) and total distance travelled (right) in GFP and ChR2 animals. Note lack of preference for stim side in either condition. (D) Strategy to express KORD in vHBA neurons. (E, F) Bath application of salvinorin B (SalB) (100 nm) hyperpolarises KORD-expressing vHBA neurons and reduces AP firing. See Figure 6—figure supplement 1 for full quantification. Scale bar = 30 mV, 100 ms. (G) Schematic of strategy to inhibit vHBA neurons during BA input-driven RTPP. (H, I) As (B, C) but comparing the effect of either DMSO (vehicle) or SalB (KORD agonist) injections 15 min before testing in control mice. Note lack of RTPP in both conditions indicating no effect of SalB in control mice. (J, K) As (H, I), but in mice expressing KORD in vHBA neurons. Note induction of RTPP in SalB-injected mice compared to controls.
-
Figure 8—source data 1
Source data for Figure 8.
- https://cdn.elifesciences.org/articles/74758/elife-74758-fig8-data1-v2.xlsx
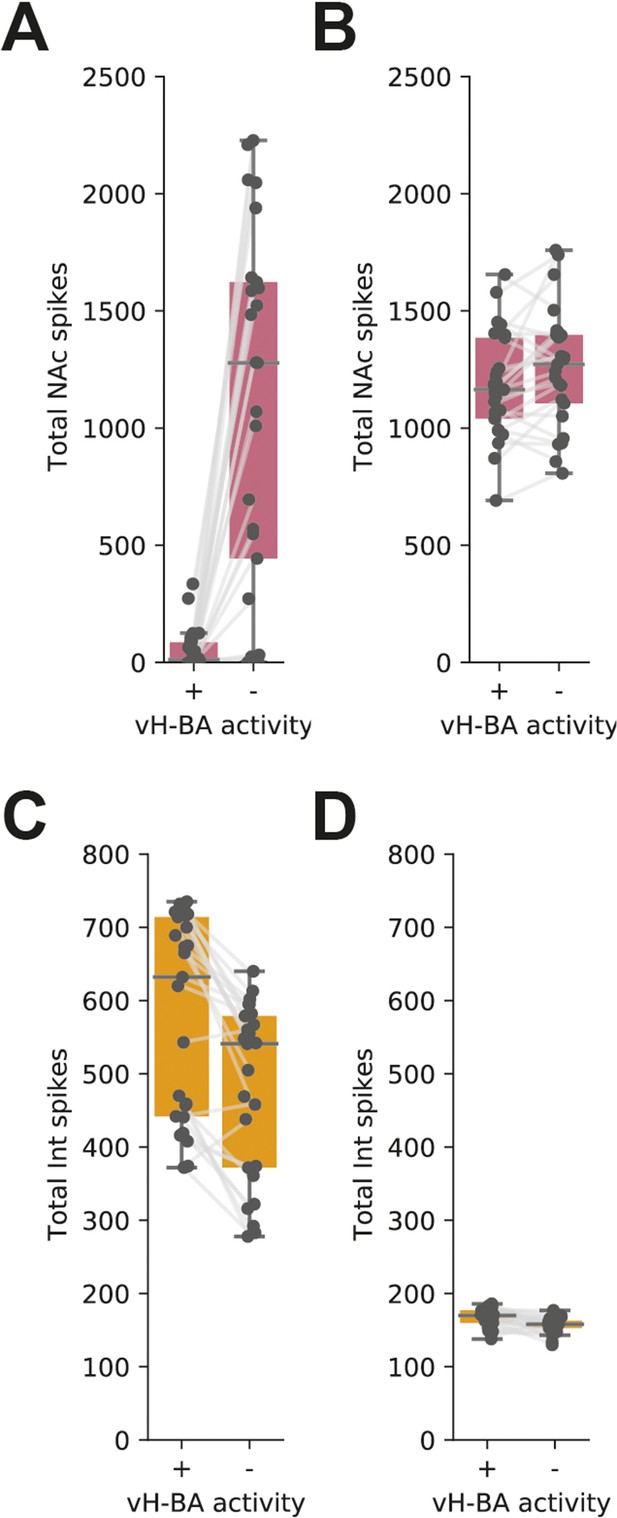
Removing vHBA activity from the integrate of fire model increases vHNAc activity in response to excitatory but not excitatory and inhibitory basal amygdala (BA) input.
(A) Total vHNAc activity with and without vHBA activity. Individual data points for all configurations of model presented in Figure 3. Note large increase in vHNAc activity on vHBA silencing across a wide range of feedback and feedforward connectivity. (B) As in (A) but with both excitatory and inhibitory input (inhibitory input scaled to 40% of excitatory input [as in example in Figure 5]). vHBA silencing has no effect as these neurons are already effectively silenced by BA inhibitory input. (C, D) As in (A,B) but for interneuron firing. vH-BA silencing reduces interneuron firing, contributing to the increase in vH-NAc activity.
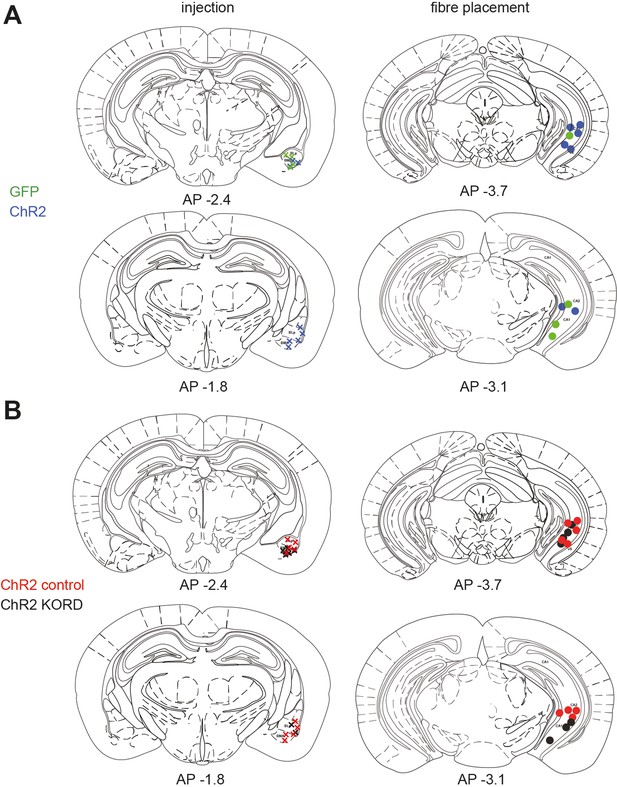
Histology for behavioural experiments in Figure 8.
(A) Histology for experiments in Figure 7A–C. (B) Histology for experiments in Figure 7G–K.
Tables
Reagent type (species) or resource | Designation | Source or reference | Identifiers | Additional information |
---|---|---|---|---|
Genetic reagent (Mus musculus) | Slc32a1(VGAT)- IRES-Cre(vGAT-cre) | Jackson Laboratory | Stock #016962;RRID:IMSR_JAX:016962 | |
Genetic reagent (M. musculus) | Ai14(RCL- tdT)-D(reporter mice) | Jackson Laboratory | Stock #007914;RRID:IMSR_JAX: 007914 | |
Genetic reagent (virus) | AAV2/1- CaMKII- GFP | Addgene | Stock #64545-AAV1 | A gift from Edward Boyden |
Genetic reagent (virus) | AAV2retro- CAG-Cre | UNC vector core (Tervo et al., 2016) | ||
Genetic reagent (virus) | AAV2/1-EF1a- FLEX-hChR2 (H134R)- EYFP | Addgene | Stock #20298-AAV1 | A gift from Karl Deisseroth |
Genetic reagent (virus) | AAV2/1-hSyn- hChR2 (H134R)-EYFP | Addgene | Stock #26973-AAV1 | A gift from Karl Deisseroth |
Genetic reagent (virus) | AAV2/1-CaMKII-hChR2 (H134R)-EYFP | Addgene | Stock #26969-AAV1 | A gift from Karl Deisseroth |
Genetic reagent (virus) | pAAV2/8-hSyn-dF-HA-KORD- IRES-mCitrine | Addgene | Stock #6541-AAV8 | A gift from Bryan Roth |
Genetic reagent (virus) | AAV2/1.CAG. FLEX. Ruby2sm- Flag.WPRE | Addgene | Stock #98928-AAV1 | A gift from Loren Looger |
Genetic reagent (virus) | AAV2/9-mDlx- NLS-mRuby2 | Addgene | Stock #99130-AAV1 | A gift from Viviana Gradinaru |
Genetic reagent (virus) | pAAV2/1-Ef1a- DIO mCherry | Addgene | Stock #114471-AAV1 | A gift from Karl Deisseroth |
Antibody | Anti-somatostatin antibody, clone YC7 (monoclonal) | Merck Millipore | MAB354;RRID:AB_2255365 | IHC (1:500) |
Chemical compound, drug | Salvinorin B (SalB) | Hello Bio | HB4887 | |
Chemical compound, drug | Cholera Toxin Subunit B (recombinant), Alexa Fluor 647 Conjugate | Thermo Fisher Scientific | C34778 | |
Chemical compound, drug | Cholera Toxin Subunit B (recombinant), Alexa Fluor 594 Conjugate | Thermo Fisher Scientific | C34777 | |
Chemical compound, drug | Cholera Toxin Subunit B (recombinant), Alexa Fluor 488 Conjugate | Thermo Fisher Scientific | C34775 | |
Software, algorithm | Python 3.7 | https://www.python.org/ | RRID:SCR_008394 | |
Software, algorithm | Jupyter Notebook | https://www.jupyter.org/ | RRID:SCR_018315 | |
Software, algorithm | ImageJ (Fiji) | https://www.fiji.sc/ | RRID:SCR_002285 |
Additional files
-
Supplementary file 1
Statistical summary of main figures.
- https://cdn.elifesciences.org/articles/74758/elife-74758-supp1-v2.docx
-
Supplementary file 2
Statistical summary of figure supplements.
- https://cdn.elifesciences.org/articles/74758/elife-74758-supp2-v2.docx
-
Transparent reporting form
- https://cdn.elifesciences.org/articles/74758/elife-74758-transrepform1-v2.docx