Distinct representation of cue-outcome association by D1 and D2 neurons in the ventral striatum’s olfactory tubercle
Figures
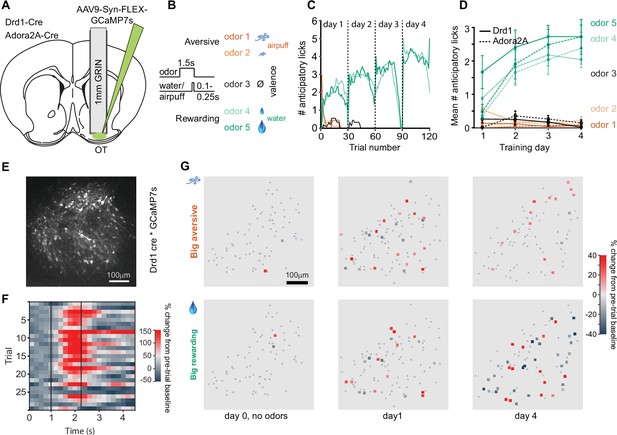
Two-photon calcium imaging of D1- and D2-type neurons in the olfactory tubercle (OT) during odor-outcome association learning.
(A) Cannula and Gradient-Index (GRIN) lens implantation targeting the OT in Drd1-Cre and Adora2A-Cre mice with AAV9-Syn-FLEX-GCaMP7s virus injection in the OT. (B) Odor-outcome task structure, odors 1–2 are paired with aversive airpuffs and odors 4–5 are paired with rewarding water drops in headfixed water restricted mice. Odor-outcome assignments are counterbalanced across mice. (C) Number of anticipatory licks in an example mouse in a 1 s period after odor onset and prior to water or airpuff delivery. Each training day has 30 trials of each of the five odors. (D) Mean number of anticipatory licks across 4 days of training in implanted Drd1-Cre mice (n=6, solid) and Adora2A-Cre mice (n=6, dashed). (E) Field of view of GCaMP7s expressing neurons in a Drd1-Cre mouse. (F) Example imaged neuron with activity in individual odor 5 trials in a single session. Dashed lines indicate odor onset and water onset. (G) Neuronal activity in field of view of a Drd1-Cre mouse in big airpuff and big water drop trials across days of training. Small gray dots indicate non-significantly responsive neurons. Day 0 indicates pre-training day in which no odors are presented, only water drops and airpuffs. Activity is shown in the 1 s period prior to outcome delivery, after odor onset in days 1 and 4.

Two-photon imaging of calcium activity in Drd1-Cre and Adora2A-Cre transgenic mice.
(A) 1 mm cannula targeting the olfactory tubercle (OT) in (left) Drd1-Cre mice injected with AAV9-Syn-FLEX-GCaMP7s and (middle and right) Adora2A-Cre mice injected with AAV9-Syn-FLEX-GCaMP7s. (B) Calcium transients recorded in 10 randomly selected D2 neurons.

Anticipatory licking in trained mice.
(A) The mean onset of the first lick to odor 5 (big reward predicting) during learning was similar for Drd1-Cre (n=6) and Adora2A-Cre (n=6) mice. (B) Time course of licking for all five odors in the same mice (day 4). (C) The full distribution of the times of first lick after odor onset of odor 5 (big reward predicting) in day 4 of training for both Drd1-Cre and Adora2A-Cre mice (m=360 trials in 12 mice). (D) The delay between the solenoid valve opening (which is denoted as time 0 throughout the manuscript) and the arrival of the odor at the mouse’s nostrils was measured with a miniPID instrument (Aurora Scientific, Inc), and was found to be reliably ~100 ms across trials and odors. For comparison, the time between successive imaging frames was 200 ms.
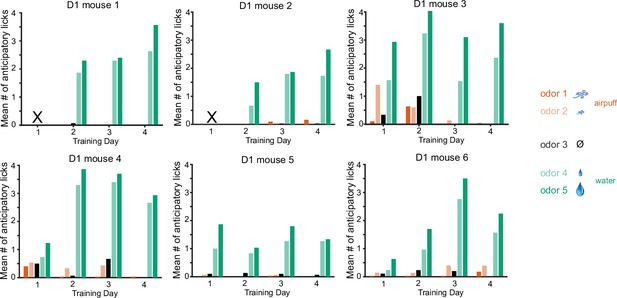
Anticipatory licking for each of the six Drd1-Cre mice.
Licking to rewarded cues develops rapidly by day 2, and any licking to aversive cues on day 1 is suppressed in later days. The number of licks for the cue predicting larger reward (odor 5) is larger than that for the cue predicting smaller reward (odor 4).

Cell size distributions for D1 and D2 neurons.
(A) Panels reproduced from the Allen mouse atlas, showing expression of Drd1 and A2A in the olfactory tubercle (OT). (B) Sample fluorescence images from Drd1-Cre and Adora2A-Cre mouse brains imaged in vivo, with putative islands of calleja marked as ‘IC?’. (C, D) Distribution of soma sizes (measured from fluorescence images) for D1 and D2 neurons reveal very few neurons with sizes less than 10 μm.

Anticipatory licking of C57BL/6 WT mice with no craniotomy/Gradient-Index (GRIN) lens implant.
Mice were trained in the odor-sound task with the same stimuli as the implanted mice, without prior training on the odor-odor task (n=13 mice). The odor-sound task is described in detail later in the paper.
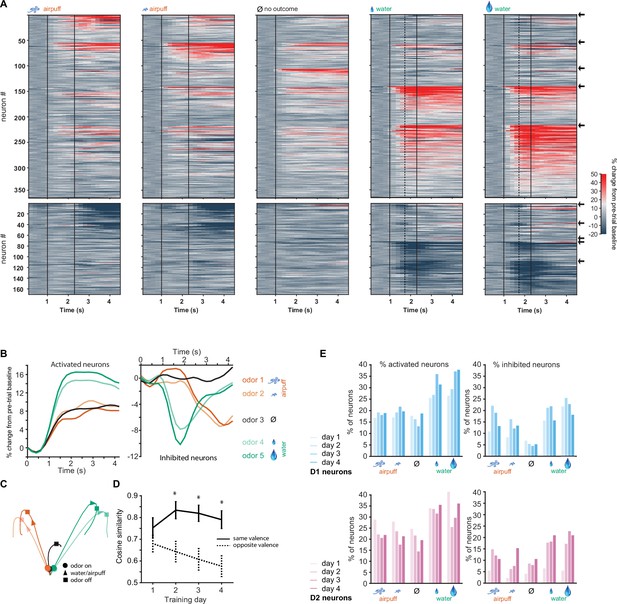
D1-type neurons in the olfactory tubercle (OT) respond most strongly to rewarded odors and respond similarly to odors of similar outcomes.
(A) Activity of all activated (top) and inhibited (bottom) neurons from six Drd1-Cre mice in five trial types on day 4 of training. Vertical black lines indicate odor onset and outcome delivery time. Vertical dashed lines in the two right-most panels indicate average time of first lick by mice. Neurons are grouped by preferred stimulus, arrows on right indicate boundaries between groups. Neuronal activity in the two aversive trial types (columns 1–2) is similar and neuronal activity in the two rewarding trial types (columns 4–5) is similar. (B) Mean population activity of all activated and inhibited D1 neurons in five trial types. Odor onset at 1 s and outcome onset at 2.3 s. (C) 3D D1 neural population trajectories in five trial types. (D) Cosine similarity between groups of neurons responding to odor pairs of same valence or opposite valence (five neuronal subgroups as in A with pairwise comparisons, *p<0.05 rank sum test). (E) Proportions of significantly activated and inhibited D1 and D2 neurons on days 1–4 of training (day 4: n=529 D1 neurons, n=338 D2 neurons).
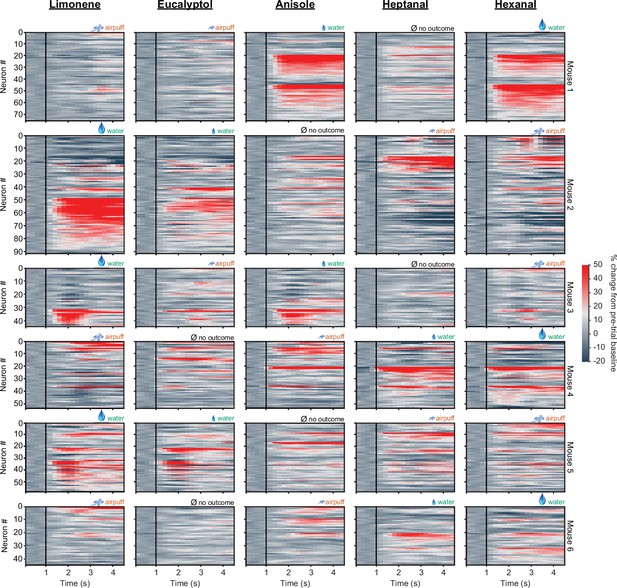
D1 neuronal activity for individual odors and mice.
Neural activity is shown for each of six mice (each row), and each column corresponds to a one of five odors. Odors were associated with different outcomes (randomly) for each mouse. Neurons for each mouse are grouped by preferred stimulus. Vertical black lines indicate odor onset.
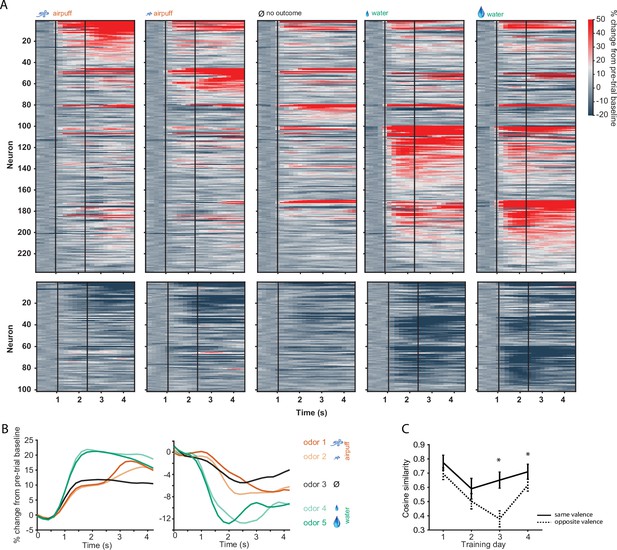
D2 neuronal activity in odor-outcome association task.
(A) Activity of all activated (top) and inhibited (bottom) D2-type neurons in five trial types on day 4 of training. Neurons are grouped by preferred stimulus. Vertical black lines indicate odor onset and outcome delivery time. (B) Mean population activity of all activated and inhibited D2 neurons in five trial types. Odor onset at 1 s and outcome onset at 1.3 s. (C) Cosine similarity between groups of neurons responding to odor pairs of same valence or opposite valence (five neuronal subgroups as in A, with pairwise comparisons, *p<0.05 rank sum test).
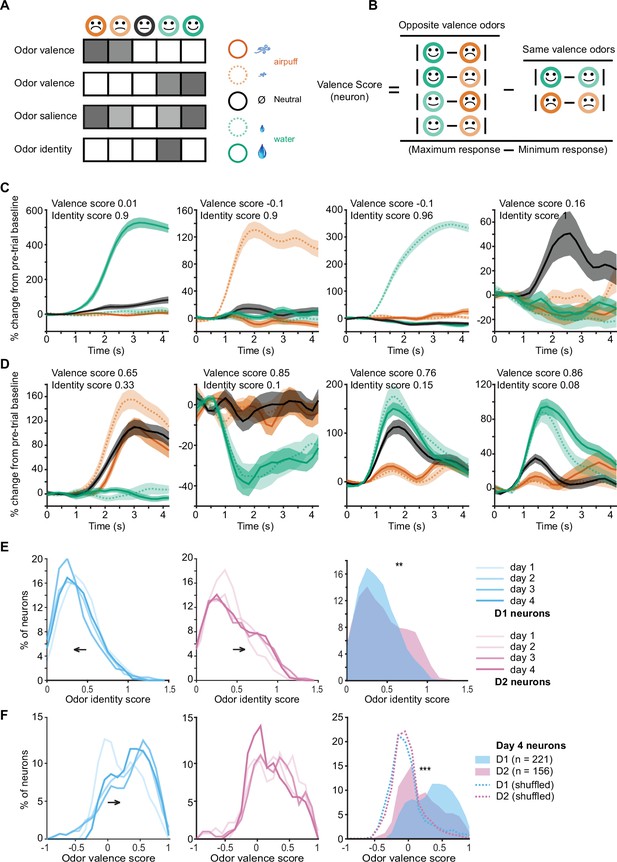
Odor valence and identity coding in individual D1 and D2 olfactory tubercle (OT) neurons develops with learning.
(A) Hypothetical responses to the five task odors in idealized odor valence coding neurons, salience coding neurons, or odor identity coding neurons. (B) Calculation of valence scores for individual neurons by comparison of responses to odors of opposite valence to odors of similar valence. (C) Examples of four individual neurons with high odor identity coding scores and low valence coding scores. (D) Examples of four individual neurons with high valence coding scores and low identity coding scores. (E) Distributions of odor identity coding scores in D1 (blue, left) and D2 (pink, middle) neurons across 4 days of training. D1 neuron identity scores decrease and D2 neuron identity coding scores increase with training days. Right, day 4 distributions of identity scores of D1 and D2 neurons (**p<0.001, rank sum test). (F) Distributions of odor valence coding scores in D1 (blue, left) and D2 (pink, middle) neurons across 4 days of training. D1 neuron valence scores increase with training days. Right, day 4 distributions of valence scores of D1 and D2 neurons (***p<0.000001, rank sum test). Dotted lines show shuffled (control) distributions where the the odor-valence pairing has been randomized.
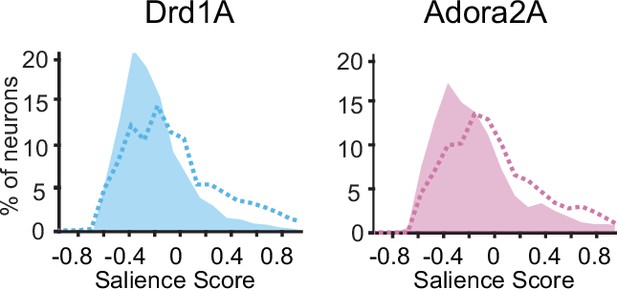
Distributions of salience scores for D1 and D2 neurons.
A comparison with shuffle control (dashed lines) indicates that there is greater than chance occurrence of negative scores (as expected if valence coding is strongly prevalent, skewing the salience scores). There were 294 D1 neurons and 177 D2 neurons.
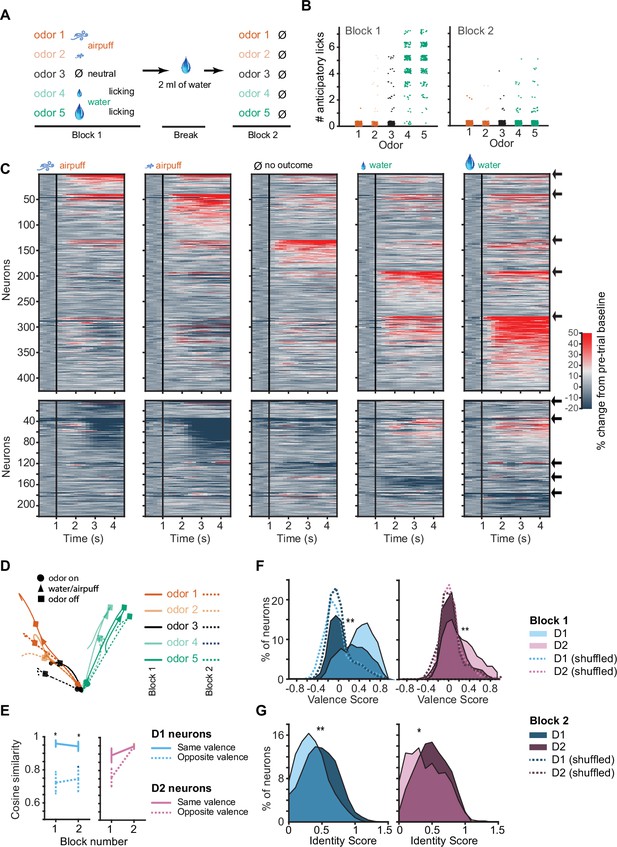
D1 neuronal valence coding is robustly preserved in the absence of licking response and absence of outcomes.
(A) Probe session structure. In Block 2 mice are sated with water, rarely exhibiting licking, and all outcomes are omitted. (B) Number of anticipatory licks in response to five-odor types in Blocks 1 and 2 (n=12 mice, 16 trials each odor type in each block). Rare trials with non-zero anticipatory licks in Block 2 were omitted from following neuronal analysis. (C) Activity of activated (top) and inhibited (bottom) D1 neurons in response to five-odor types in Block 2. Neurons are grouped by preferred stimulus, arrows on right indicate boundaries between groups. Black vertical lines indicate odor onset. No outcome was delivered. (D) 3D D1 neuronal population trajectories in Blocks 1 (solid lines) and 2 (dashed lines) in five trial types. (E) Cosine similarity between D1 and D2 neuronal activity averages in Blocks 1 and 2 for same valence and opposite valence odors (five neuronal subgroups as in C with pairwise comparisons, *p<0.05 rank sum test). (F) Distributions of D1 (left) and D2 (right) neuron valence scores in blocks 1 and 2 (n=294 D1 and 177 D2 neurons, **p<0.0001, paired t-test). Dotted lines show shuffled (control) distributions where the odor-valence pairing has been randomized. (G) Distributions of D1 and D2 neuron identity scores. Same neurons as in panel F.
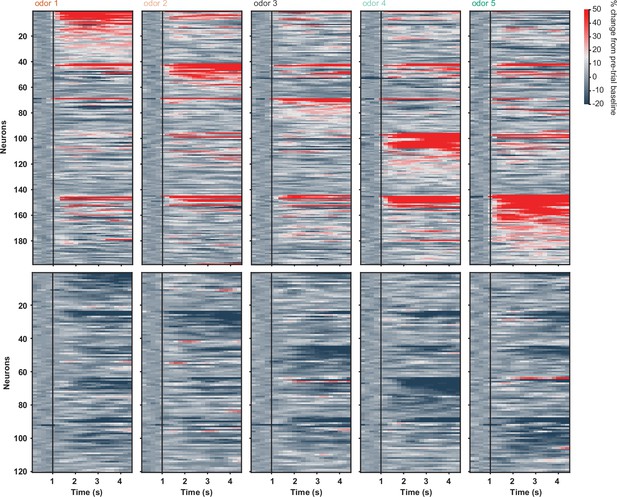
D2 neuronal activity in sated Block 2 with no licking and no water/airpuff outcomes.
Activity of all activated (top) and inhibited (bottom) D2 neurons in response to five-odor types in Block 2. Neurons are grouped by preferred stimulus. Black vertical lines indicate odor onset. No outcome was delivered and only trials with zero licks were included in analysis.
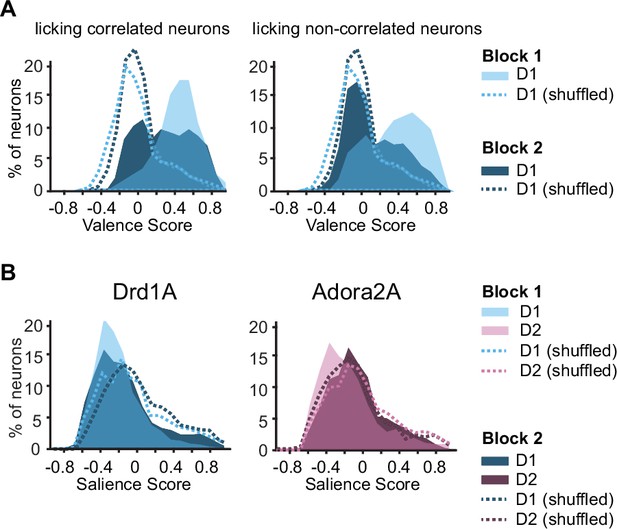
Further evidence for valence coding by D1 neurons in Block 2.
(A) Valence score distributions of responsive D1 neurons the activity of which was significantly correlated with number of anticipatory licks (left, n=72) and those which were not (right, n=222) in Blocks 1 and 2 of the five-odor task. The valence scores of the Block 1 licking correlated neurons were significantly higher in Block 2 (in the absence of licking) than those of the non-licking correlated neurons in Block 2 (rank sum test p<0.0001). (B) Distributions of D1 (left) and D2 (right) neuron salience scores in Blocks 1 and 2 (n=294 D1 and 177 D2 neurons). A comparison with shuffle control (dashed lines) indicates that there is greater than chance occurrence of negative scores (as expected if valence coding is strongly prevalent, skewing the salience scores).

Neutral odor responses of valence coding neurons.
(A) Left, difference between neuronal response to odors 3 vs. 4 (neutral vs. small water reward) and odor 3 vs. 2 (neutral vs. weak airpuff) for D1 neurons in Block 1 with valence scores >0.5 (n=101 neurons). Right, histogram of difference between odors 4 vs. 3 and odors 2 vs. 3. Neurons with negative values have neutral odor responses that trend with the aversive odor responses and neurons with positive values have neutral odor responses that trend with rewarded odor responses. (B) Same for D1 neurons in Block 2 with no licking or outcomes with valence scores >0.5 (n=52 neurons). (C) Same for D2 neurons in Block 1 with valence scores >0.5 (n=32 neurons). There were not sufficient D2 neurons in Block 2 with valence scores of >0.5 to plot.
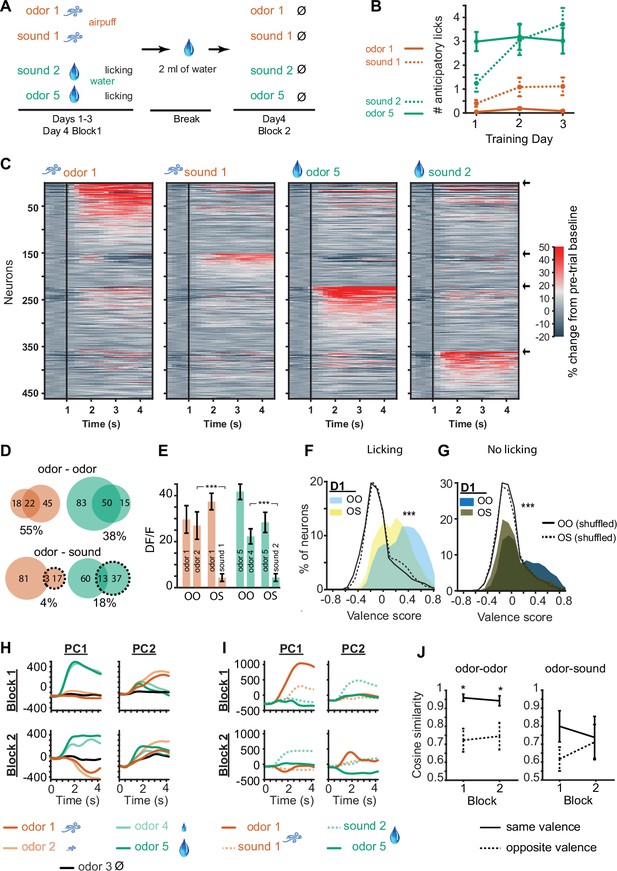
Odors and sound tones associated with identical aversive and rewarding outcomes activate different D1 neuronal subpopulations in the olfactory tubercle (OT).
(A) Odor-sound association task structure. Three days of odor-sound training beginning at the end of the prior five-odor task training were conducted. Odors 1 and 5 associated with the strongest aversive and rewarding outcomes from previously learned five-odor task were preserved and two sound tones (5 and 12 kHz) were introduced with matching outcomes. Sound tone–outcome assignments were counterbalanced across mice. On day 4, a probe session was conducted as previously in which mice were sated prior to the second block. In the second block, mice did not exhibit licking and all outcomes were omitted. (B) Anticipatory licking of mice across three training days. By day 2, mice exhibit similar levels of anticipatory licking in response to the rewarded sound tone as to the rewarded odor (n=10 mice). (C) D1 neuronal population activity in Block 2 of day 4, in response to the learned odors and sound tones, and in the absence of licking, airpuffs, or water delivery. Neurons are grouped by preferred stimulus, arrows on right indicate boundaries between groups. Unlike in Figure 4C, distinct sets of D1 neurons are activated in response to odors and sound tones associated with identical outcomes. (D) Overlap in neurons responding to stimuli predicting similar aversive (orange) and rewarding (green) outcomes in the sated condition in the odor-odor task (top) and odor-sound task (bottom). Top orange, numbers of neurons in Block 2 of the odor-odor task that were activated in response to either aversive odor 1, aversive odor 2, or both of them (overlap), with % overlap indicated below. Top green, same for rewarding odors 5 and 4. Bottom, same for Block 2 of the odor-sound task (sounds in dashed lines). (E) Orange, mean activity of odor 1 activated neurons to the corresponding matched valence odor stimulus in Block 2 of the odor-odor (OO) task and matched valence sound stimulus in Block 2 of the odor-sound (OS) task (n=50 and 101 neurons). Green, same for odor 5 responsive neurons (n=100 and 134). (F) Distributions of valence scores of D1 neurons in the odor-odor task and odor-sound task in the full task condition with licking and outcomes. Shuffle control distributions are also shown as lines. (G) Same for stimulus-only condition in Block 2. (H) Principal components of D1 neuronal population activity in Blocks 1 and 2 in odor-odor task. (I) Same for odor-sound task. (J) Cosine similarity in the neuronal trajectories of stimulus responsive neuronal groups in the odor-odor task as compared to the odor-sound task in same valence vs. opposite valence stimulus pairs (four neuronal subgroups as in C with pairwise comparisons, *p<0.05 rank sum test).
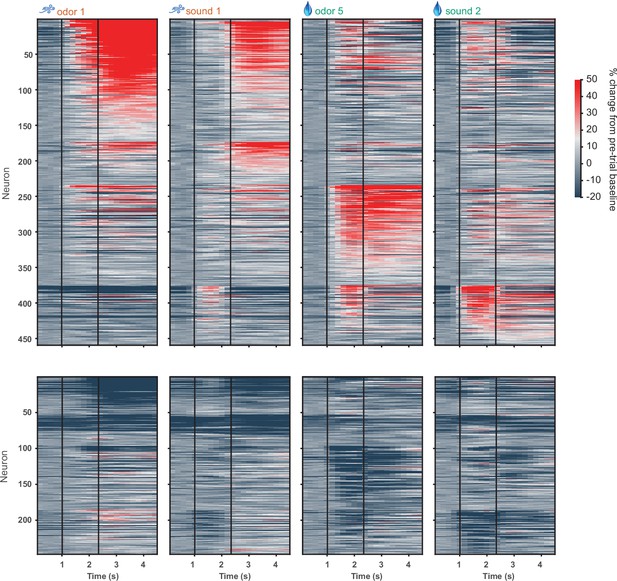
D1 neuronal activity in full odor-sound task, including licking and water/airpuff outcomes.
Activity of all activated (top) and inhibited (bottom) D1 neurons in four trial types on day 3 of training in the odor-sound task. Neurons are grouped by preferred stimulus. Vertical black lines indicate odor onset and outcome delivery time.
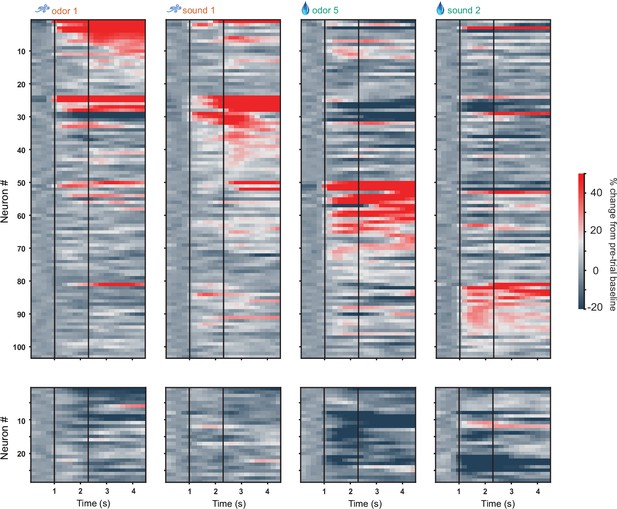
D2 neuronal activity in full odor-sound task, including licking and water/airpuff outcomes.
Activity of all activated (top) and inhibited (bottom) D2 neurons in four trial types on day 3 of training in the odor-sound task. Neurons are grouped by preferred stimulus. Vertical black lines indicate odor onset and outcome delivery time.

D2 neuronal activity in sated Block 2 of odor-sound task with no licking and no water/airpuff outcomes.
(A) D2 neuronal population activity in Block 2 of day 4, in response to the learned odors and sound tones, and in the absence of licking, airpuffs, or water delivery. Neurons are grouped by preferred stimulus. Black vertical lines indicate odor onset. No outcome was delivered and only trials with zero licking were included in the analysis. (B) Distributions of valence scores of D2 neurons in the odor-odor task and odor-sound task in the full task condition with licking and outcomes. (C) Similar distributions as in panel B for stimulus-only (no outcome) condition in Block 2. No significant differences were observed between these distributions.