A cryptic K48 ubiquitin chain binding site on UCH37 is required for its role in proteasomal degradation
Figures
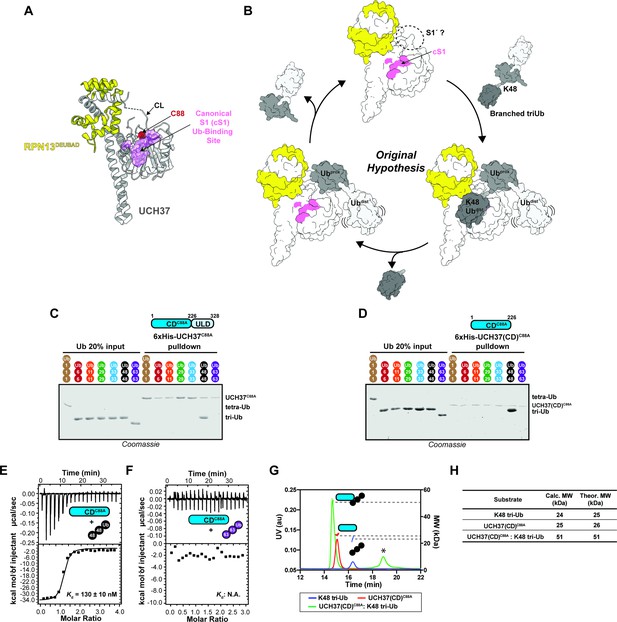
The catalytic domain (CD) of UCH37 confers K48 linkage specificity.
(A) Structure of UCH37 bound RPN13DEUBAD (PDB ID: 4UEL; see also 4WLR) highlighting residues comprising the canonical S1 (cS1) Ubiquitin (Ub)-binding site (shown in pink). (B) Original hypothesis for how UCH37 uses the cS1 site along with an unknown S1' site to catalyze K48 chain debranching. (B) SDS-PAGE analysis of 6×His-UCH37C88A-mediated pulldowns with M1-, K6-, K11-, K29-, K33-, K48-, and K63-linked Ub chains. Each chain (50 pmol) was mixed with 6×His-UCH37C88A (5 nmol) immobilized on Ni-NTA resin. (C) SDS-PAGE analysis of 6×His-UCH37(CD)C88A with M1-, K6-, K11-, K29-, K33-, K48-, and K63-linked Ub chains. Each chain (50 pmol) was mixed with 6×His-UCH37(CD)C88A (5 nmol) immobilized on Ni-NTA resin. (D–E) Isothermal titration calorimetry analysis of UCH37(CD)C88A binding to K48 tri-Ub (C), and K63 tri-Ub. (D) (F) Size exclusion chromatography coupled with multiangle light scattering (SEC-MALS) analysis of the interaction between UCH37(CD)C88A and K48 tri-Ub. * denotes a UV peak without corresponding light scattering data. (G) Theoretical and calculated molar mass of complexes detected by SEC-MALS.
-
Figure 1—source data 1
ITC and SEC-MALs analysis of UCH37C88A and UCH37C88A (CD) with Ub chains.
- https://cdn.elifesciences.org/articles/76100/elife-76100-fig1-data1-v1.xlsx
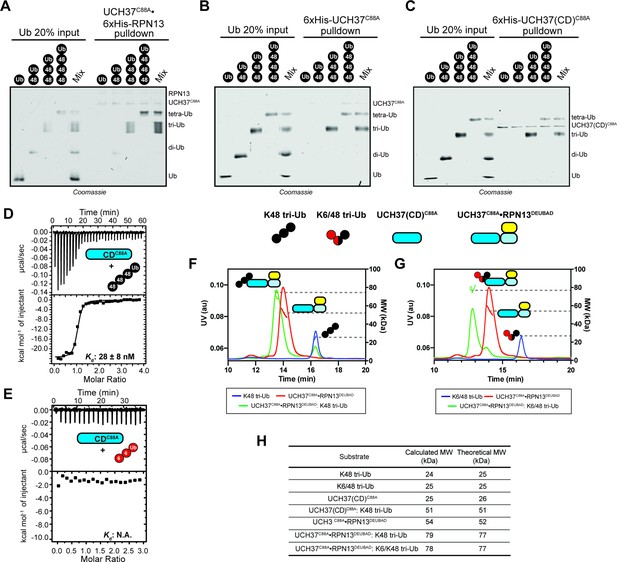
The catalytic domain (CD) of UCH37 confers K48 linkage specificity.
(A) SDS-PAGE analysis of pulldowns using Ni-NTA immobilized UCH37C88A•6xHis-RPN13 and mono-, K48 di-, K48 tri-, and K48 tetra-ubiquitin (Ub). (B) SDS-PAGE analysis of pulldowns using Ni-NTA immobilized 6xHis-UCH37C88A and mono-, K48 di-, K48 tri-, and K48 tetra-Ub. (C) SDS-PAGE analysis of pulldowns using Ni-NTA immobilized 6xHis-UCH37(CD)C88A and mono-, K48 di-, K48 tri-, and K48 tetra-Ub. (D) Isothermal titration calorimetry (ITC) analysis of UCH37(CD)C88A binding to K48 tetra-Ub. (E) ITC analysis of UCH37(CD)C88A binding to K6 tri-Ub. (F) Size exclusion chromatography coupled with multiangle light scattering (SEC-MALS) analysis of the interaction between UCH37C88A•RPN13DEUBAD and K48 tri-Ub. (G) SEC-MALS analysis of the interaction between UCH37C88A•RPN13DEUBAD and K6/K48 tri-Ub. (H) Theoretical and calculated molar mass of complexes detected by SEC-MALS.
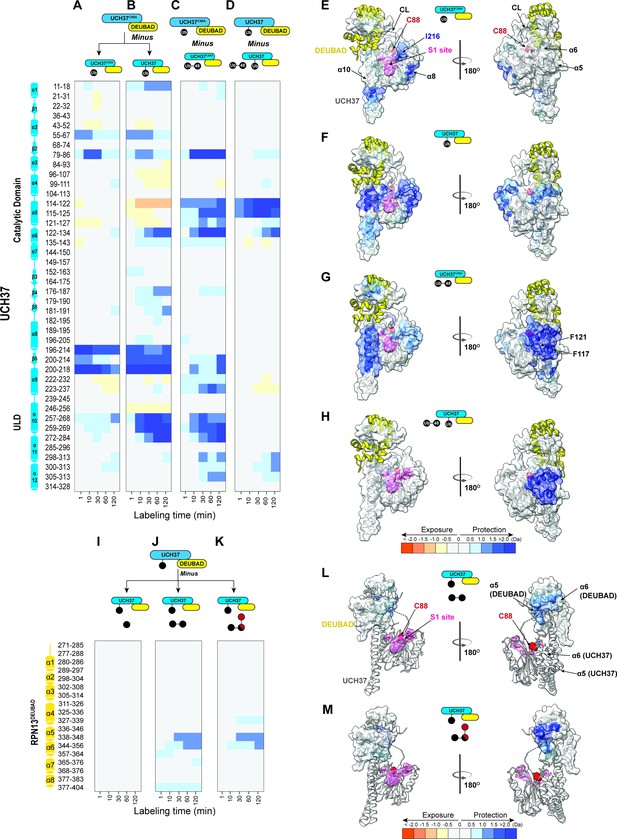
Hydrogen-deuterium exchange mass spectrometry (HDX-MS) uncovers a cryptic K48 chain-specific binding site.
(A) Differential deuterium uptake plot comparing mono-ubiquitin (Ub) bound UCH37C88A•RPN13DEUBAD to free UCH37C88A •RPN13DEUBAD. (B) Differential deuterium uptake plot comparing UCH37•RPN13DEUBAD covalently linked to Ub propargylamine at the active site Cys (Ub~UCH37•RPN13DEUBAD) to free UCH37C88A •RPN13DEUBAD. (C) Differential deuterium uptake plot comparing K48 di-Ub-bound UCH37C88A•RPN13DEUBAD to mono-Ub-bound UCH37C88A•RPN13DEUBAD. (D) Differential deuterium uptake plot comparing K48 di-Ub-bound Ub ~UCH37•RPN13DEUBAD to free Ub ~UCH37•RPN13DEUBAD. (E–G) Structure of UCH37 (PDB ID: 4UEL) showing regions with statistically significant differences in exchange upon noncovalent binding to mono-Ub (E), covalent attachment of Ub to the active site (F), and noncovalent binding to K48 di-Ub. (G) Data correspond to 2 hr of deuterium labeling. Other highlighted regions include the catalytic Cys (C88), the crossover loop (CL), and the canonical S1 site (pink). (H) Structure of UCH37 (PDB ID: 4UEL) showing statistically significant differences in exchange upon noncovalent binding of K48 di-Ub to Ub ~UCH37•RPN13DEUBAD. Data correspond to 2 hr of deuterium labeling. (I–K) Differential deuterium uptake plot comparing the effects of mono-Ub (I), K48 di-Ub (J), and K6/K48 tri-Ub (K) binding to Ub ~UCH37•RPN13DEUBAD on the exchange of residues in RPN13DEUBAD. (L–M) Heat map showing regions of RPN13DEUBAD with statistically significant differences in deuterium exchange upon noncovalent binding to K48 di-Ub (L), and noncovalent binding to K6/K48 tri-Ub (M). Data correspond to 2 hr of deuterium labeling.
-
Figure 2—source data 1
HDX-MS analysis of UCH37 and RPN13DEUBAD.
- https://cdn.elifesciences.org/articles/76100/elife-76100-fig2-data1-v1.xlsx
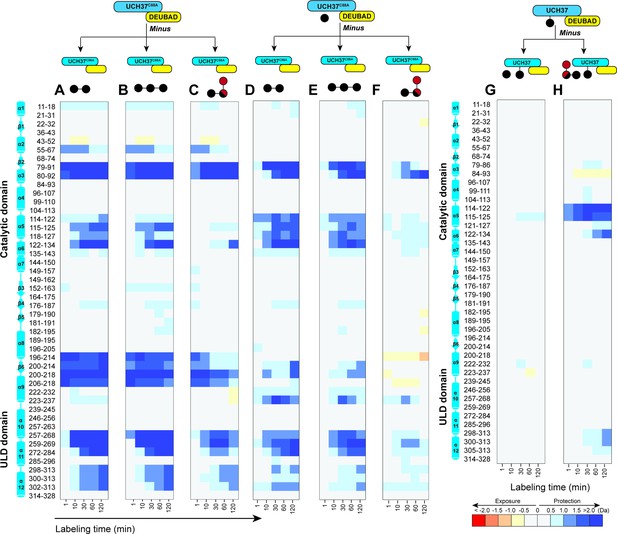
Hydrogen-deuterium exchange mass spectrometry uncovers a cryptic K48 chain-specific binding site.
(A) Differential deuterium uptake plot comparing K48 di-ubiquitin (Ub)-bound UCH37•RPN13DEUBAD and free UCH37•RPN13DEUBAD. (B) Differential deuterium uptake plot comparing K48 tri-Ub-bound UCH37•RPN13DEUBAD and free UCH37•RPN13DEUBAD. (C) Differential deuterium uptake plot comparing K6/K48 tri-Ub-bound UCH37•RPN13DEUBAD and free UCH37•RPN13DEUBAD. (D) Differential deuterium uptake plot comparing K48 di-Ub-bound UCH37•RPN13DEUBAD and mono-Ub-bound UCH37•RPN13DEUBAD. (E) Differential deuterium uptake plot comparing K48 tri-Ub-bound UCH37•RPN13DEUBAD and mono-Ub-bound UCH37•RPN13DEUBAD. (F) Differential deuterium uptake plot comparing K6/K48 tri-Ub-bound UCH37•RPN13DEUBAD and mono-Ub-bound UCH37•RPN13DEUBAD. (G) Differential deuterium uptake plot comparing mono-Ub-bound Ub~UCH37•RPN13DEUBAD and Ub~UCH37•RPN13DEUBAD. (H) Differential deuterium uptake plot comparing K6/K48 tri-Ub-bound Ub~UCH37•RPN13DEUBAD and Ub~UCH37•RPN13DEUBAD.
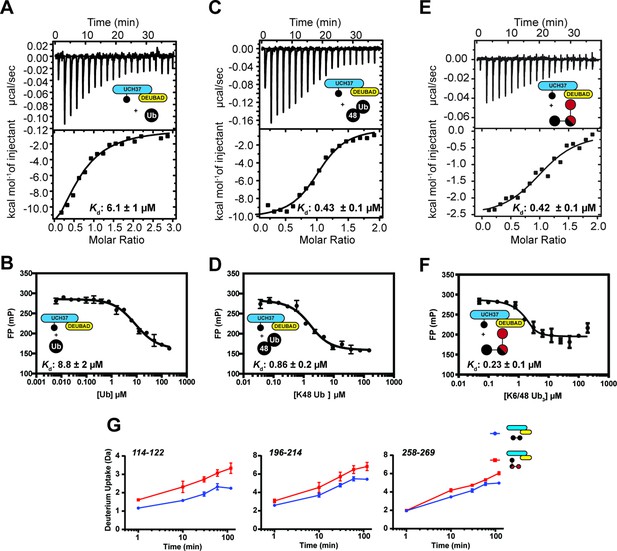
Isothermal titration calorimetry (ITC) and fluorescence polarization analysis of binding to ubiquitin (Ub) ~UCH37•RPN13DEUBAD.
(A) ITC analysis of mono-Ub binding to Ub ~UCH37•RPN13DEUBAD. (B) Competitive FP analysis of the interaction between mono-Ub and Ub ~UCH37•RPN13DEUBAD. (C) ITC analysis of K48 di-Ub binding to Ub ~UCH37•RPN13DEUBAD. (D) Competitive FP analysis of the interaction between K48 di-Ub and Ub~UCH37•RPN13DEUBAD. (E) ITC analysis of K6/K48 tri-Ub binding to Ub ~UCH37•RPN13DEUBAD. (F) Competitive fluorescence polarization analysis of the interaction between K6/K48 tri-Ub and Ub~UCH37•RPN13DEUBAD. (G) Deuterium uptake profiles comparing the effects of K6/K48 tri-Ub and K48 di-Ub on three different regions of UCH37.
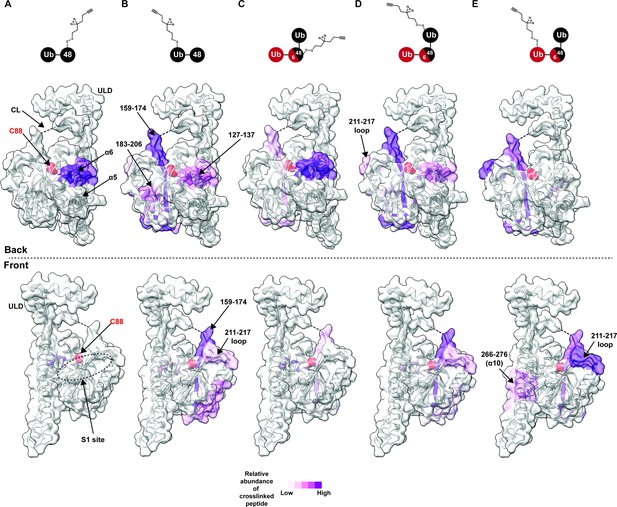
Chemical crosslinking confirms the presence of a K48 chain-specific binding site on the backside of UCH37.
(A–E) A photolabile diazirine-based crosslinker was appended to individual ubiquitin (Ub) subunits of K48 chains. Crosslinked peptides were mapped onto the structure of UCH37 (PDB: 4UEL) according to their relative abundance based on the area under the curve of the extracted ion chromatogram. (A) Map of the crosslinking data for K48 di-Ub binding to UCH37C88A•RPN13DEUBAD with the proximal Ub subunit labeled with the diazirine colored by normalized relative abundance of crosslinked peptide. (B) Map of the crosslinking data for K48 di-Ub binding to UCH37C88A•RPN13DEUBAD with the distal Ub subunit labeled with the diazirine colored by normalized relative abundance of crosslinked peptide. (C) Map of the crosslinking data for K6/K48 tri-Ub binding to UCH37C88A•RPN13DEUBAD with the proximal Ub subunit labeled with the diazirine colored by normalized relative abundance of crosslinked peptide. (D) Map of the crosslinking data for K6/K48 tri-Ub binding to UCH37C88A•RPN13DEUBAD with the K48-linked distal Ub subunit labeled with the diazirine colored by normalized relative abundance of crosslinked peptide. (E) Map of the crosslinking data for K6/K48 tri-Ub binding to UCH37C88A•RPN13DEUBAD with the K6-linked distal Ub subunit labeled with the diazirine colored by normalized relative abundance of crosslinked peptide.
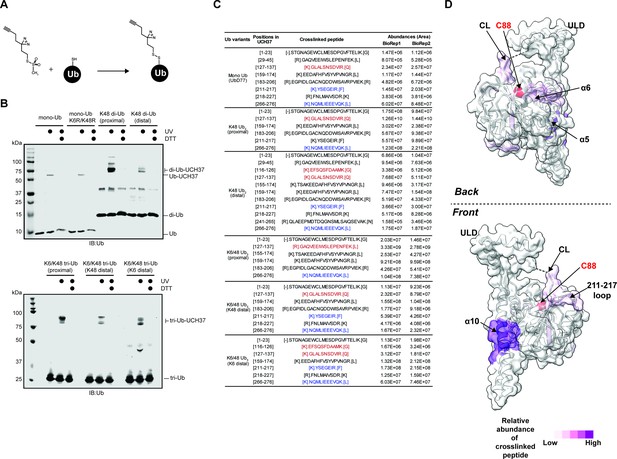
Chemical crosslinking confirms the presence of a K48 chain-specific binding site on the backside of UCH37.
(A) Scheme showing the installation of the diazirine crosslinker at position-46 of ubiquitin (Ub). (B) Western blot analysis of crosslinking reactions with the indicated Ub variants. Immunoblotting was performed with the α-Ub antibody. (C) Crosslinked peptides detected by mass spectrometry in two biological replicates. Color coded based on location. (D) Map of the crosslinking data for mono-Ub binding to UCH37C88A•RPN13DEUBAD colored by normalized relative abundance of crosslinked peptide.
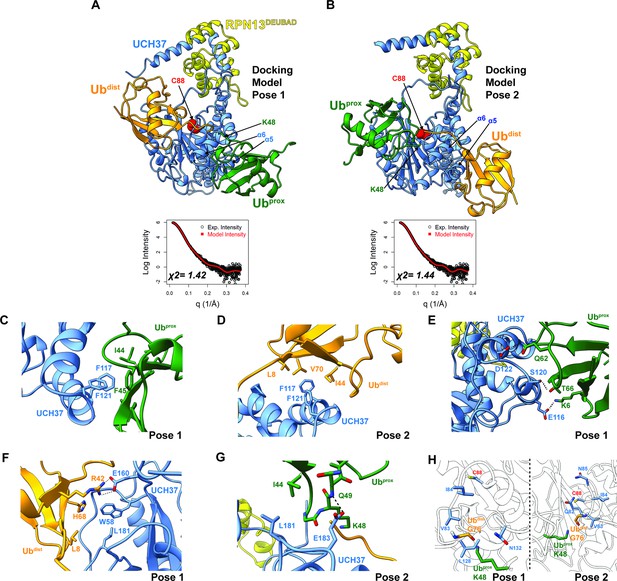
Docking models of the K48 di-ubiquitin (Ub):UCH37•RPN13DEUBAD complex.
(A–B) HADDOCK docking models show two poses corresponding to the interaction between K48 di-Ub and UCH37 along with their fit to experimental small-angle X-ray scattering data of the K48 di-Ub:UCH37C88A•RPN13DEUBAD complex. The goodness of fit to the experimental intensity is represented by χ2 values. In the first pose (A), the proximal Ub (Ubprox; green) interacts with α5–6 of UCH37. In the second pose (B), the distal Ub (Ubdist; orange) interacts with α5–6 of UCH37. (C) Residues highlighting the interaction between the aromatic-rich region of UCH37 α5–6 and the I44 patch of Ubprox in pose 1 (A). (D) Residues highlighting the interaction between the aromatic-rich region of UCH37 α5–6 and the I44 patch of Ubdist in pose 2 (B). (E) Polar contacts between Ubprox and UCH37 α5–6 in pose 1 (A). (F) Contacts between Ubdist and residues of UCH37 located outside the α5–6 motif in pose 1 (A). (G) Contacts between Ubprox and residues of UCH37 located outside the α5–6 motif in pose 2 (B). (H) The relative location of active site and the scissile, K48 isopeptide bond in molecular docking poses 1 and 2. In pose 1, residues of α6 and the loop leading into the catalytic Cys (C88) form a barrier for the isopeptide bond. In pose 2, only Q82 of UCH37 blocks the K48 isopeptide bond.
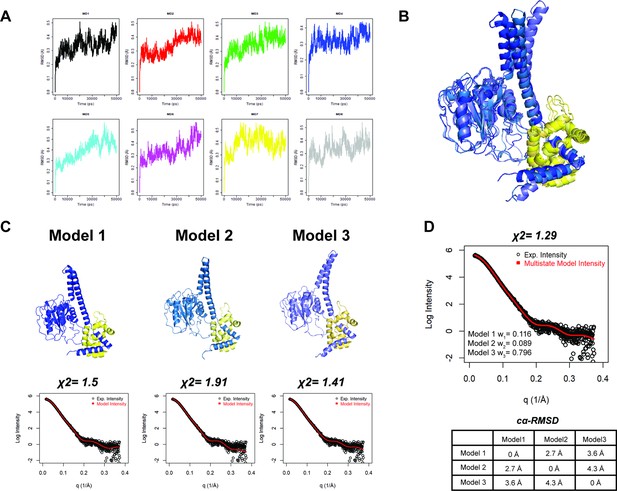
Structural models of the K48 di-ubiquitin (Ub):UCH37•RPN13DEUBAD complex.
(A) Molecular dynamics (MD) trajectories for eight independent simulations plotted as Cα-RMSD of UCH37 over time. (B) Overlay of MD-derived UCH37•RPN13DEUBAD conformations that afford the best fit to experimental small-angle X-ray scattering (SAXS) data. Flexible N- and C-termini of RPN13DEUBAD are omitted. (C) Individual structures of UCH37•RPN13DEUBAD (models 1–3) with the corresponding fits to the SAXS data. Flexible N- and C-termini of RPN13DEUBAD are omitted. (D) Theoretical and experimental SAXS data for the best-fitting multistate model comprising models 1–3. Pairwise Cα-RMSD is shown between models 1 and 3.
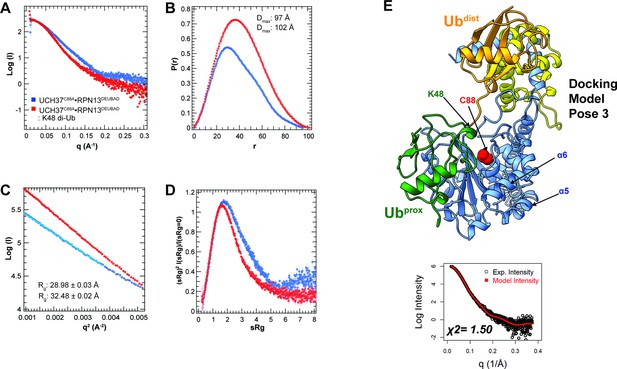
Small-angle X-ray scattering (SAXS) of the K48 di-ubiquitin (Ub):UCH37•RPN13DEUBAD complex.
(A–D) Size-exclusion chromatography-SAXS analyses of UCH37C88A•RPN13DEUBAD (blue) and K48 di-Ub:UCH37C88A•RPN13DEUBAD (red) showing distance distribution (E–F), Guinier analyses (G), and Kratky plots (G). (E) HADDOCK docking model of pose 3 showing Ubdist (orange) interacting with RPN13DEUBAD while Ubprox (green) is situated near the C-terminal end of the crossover loop (CL). The fit to the experimental scattering intensity is also shown; the goodness of fit is represented by χ2.
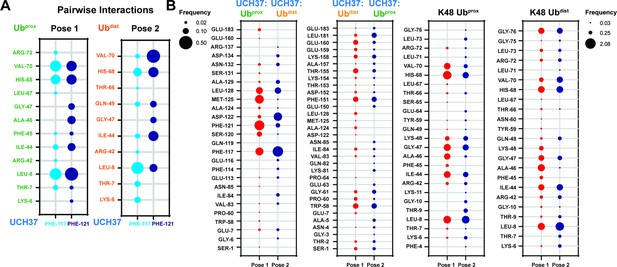
Contact frequency analysis of the K48 di-ubiquitin (Ub):UCH37•RPN13DEUBAD complex.
(A) Frequency analysis of pairwise interactions between Ubprox or Ubdist of K48 di-Ub and F117/F121 of UCH37 shown in the form of a bubble plot. (B) Contact frequencies shown for UCH37 residues that are interacting with either Ubprox or Ubdist of K48 di-Ub in poses 1 and 2. Contact frequencies for K48 di-Ub residues interacting with UCH37 in poses 1 and 2.
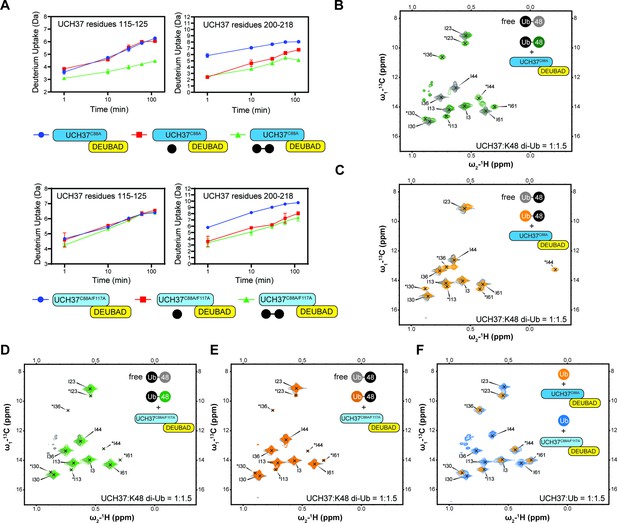
Backside mutant impairs K48 chain binding.
(A) Deuterium uptake plots showing how the F117A mutation affects hydrogen-deuterium exchange in a peptide corresponding to residues 115–125 located in the α5–6 motif of UCH37. For comparison, the uptake plots corresponding to a region located outside the α5–6 motif (residues 200–218) are also shown. The data on the top represents the rate of exchange with UCH37C88A•RPN13DEUBAD and the data on the bottom corresponds to UCH37C88A/F117A•RPN13DEUBAD. At least two replicates of each experiment were performed. (B)1H13C-methyl TROSY NMR spectra of the Ile region of ILV-labeled K48 di-ubiquitin (Ub) proximal subunit (Ubprox) free in solution (gray) and bound to UCH37C88A•RPN13DEUBAD (the C88A complex; green). Ratio of UCH37 to K48 di-Ub is 1:1.5. Concentrations used: 45 μM UCH37C88A•RPN13DEUBAD and 30 μM K48 di-Ub. (C)1H13C-methyl TROSY NMR spectra of the Ile region of ILV-labeled K48 di-Ub distal subunit (Ubdist) free in solution (gray) and bound to the C88A complex (orange). Ratio of UCH37 to K48 di-Ub is 1:1.5. Concentrations used: 45 μM UCH37C88A •RPN13DEUBAD and 30 μM K48 di-Ub. (D) 1H13C-methyl TROSY NMR spectra of the Ile region of K48-linked Ubprox free in solution (gray) and bound to UCH37C88A/F117A•RPN13DEUBAD (the F117A complex; green). Ratio of UCH37 to K48 di-Ub is 1:1.5. Concentrations used: 45 μM UCH37C88A/F117A•RPN13DEUBAD and 30 μM K48 di-Ub. (E)1H13C-methyl TROSY NMR spectra of the Ile region of K48-linked Ubdist free in solution (gray) and bound to the F117A complex (orange). Ratio of UCH37 to K48 di-Ub is 1:1.5. Concentrations used: 45 μM UCH37C88A/F117A•RPN13DEUBAD and 30 μM K48 di-Ub. (F)1H13C-methyl TROSY NMR spectra of the Ile region of mono-Ub bound to the C88A complex (orange) and the F117A complex (blue). Ratio of UCH37 to mono-Ub is 1:1.5. Concentrations used: 45 μM UCH37 •RPN13DEUBAD complex and 30 μM mono-Ub.
-
Figure 5—source data 1
Full NMR spectra of mono-Ub and K48 di-Ub in presence and absence of UCH37.
- https://cdn.elifesciences.org/articles/76100/elife-76100-fig5-data1-v1.pdf
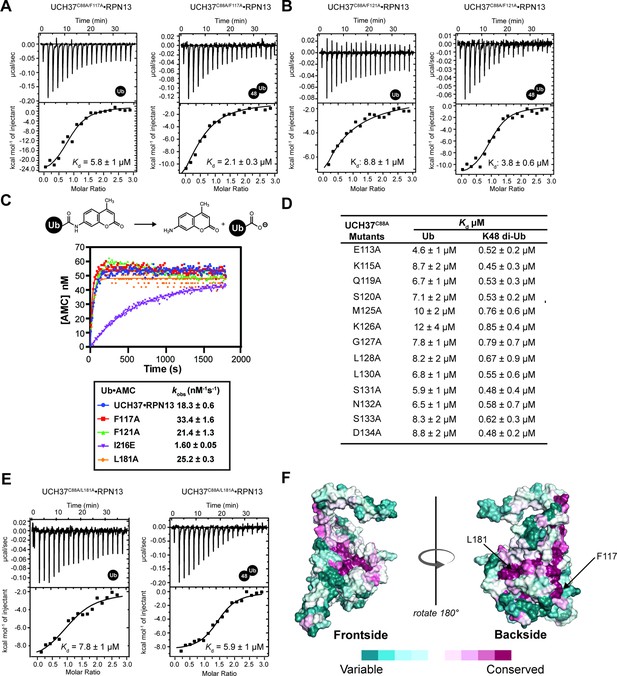
Backside mutant impairs K48 chain binding.
(A) Isothermal titration calorimetry (ITC) analyses of mono-ubiquitin (Ub) and K48 di-Ub binding to UCH37C88A/F117A•RPN13. (B) ITC analyses of mono-Ub and K48 di-Ub binding to UCH37C88A/F121A•RPN13. (C) Hydrolysis of Ub-AMC by the indicated UCH37 variants. Each variant is in complex with RPN13. (D) Table of binding affinities for different UCH37 variants measured by ITC. (E) ITC analyses of mono-Ub and K48 di-Ub binding to UCH37C88A/L181A•RPN13. (F) Surface representation of UCH37 colored by conservation.
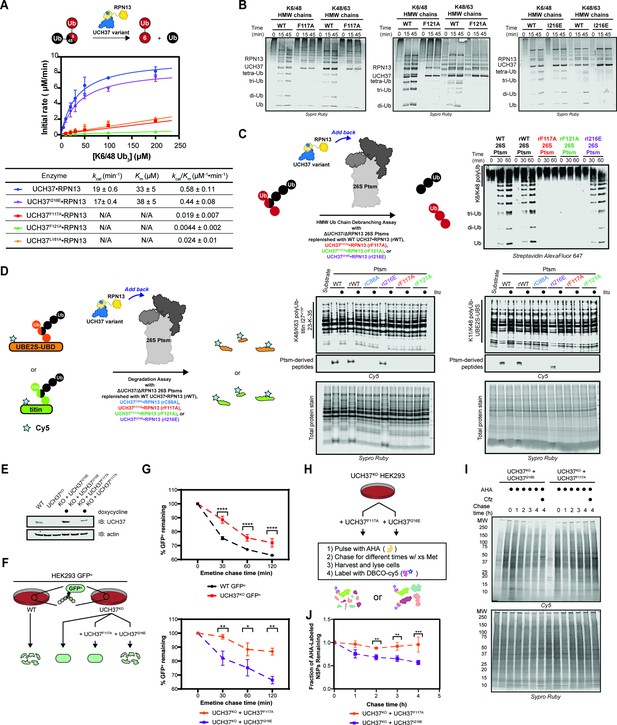
Backside mutants impair K48 chain debranching and UCH37-dependent proteasomal degradation.
(A) Steady-state kinetic analyses of the cleavage of K6/K48 tri-ubiquitin (Ub) with different UCH37 variants (WT, F117A, F121A, and I216E) in complex with RPN13. The data corresponding to WT and I216E were fit to the nonlinear Michaelis-Menten equation. The linear equation, rate = kcat/Km[K6/K48 tri-Ub][Enzyme], was sufficient to fit the data corresponding to L181A, F117A, and F121A. (B) SDS-PAGE analysis of the cleavage of K6/K48 and K48/K63 high-molecular weight (HMW) Ub chains with different UCH37 variants (WT, F117A, F121A, and I216E) in complex with RPN13. Gels were imaged by Sypro Ruby staining. (C) Western blot analysis of cleavage of K6/K48 HMW Ub chains by WT Ptsm, WT UCH37•RPN13-replenished Ptsm (rWT), UCH37F117A•RPN13-replenished Ptsms (rF117A), UCH37F121A•RPN13-replenished Ptsms (rF121A), and UCH37I216E•RPN13-replenished Ptsms (rI216E). 0.2 mg of Ptsm complex was used in each reaction. Immunoblotting was performed with Alexa Fluor 647-conjugated streptavidin. (D) Fluorescent SDS-PAGE analyses of the degradation of Cy5-labeled, K48/K63-Ubn-titin-I27V15P-23-K-35 and K11/K48-polyUb-UBE2S-UBD using WT, rWT, rC88A, rI216E, rF117A, and rF121A Ptsm complexes. Reactions were performed under multiturnover conditions and quenched after 1 hr. Gels stained for total protein are shown for reference. (E) Western blot analysis of UCH37 levels in WT, UCH37KO (KO), and KO HEK293 cells expressing UCH37 variants under a doxycycline-inducible promoter. Immunoblotting was performed with α-UCH37 and α-actin antibodies. (F) Schematic showing the different degradation profiles of the Ptsm activity reporter GFPu in WT and UCH37KO HEK293 cells expressing UCH37 variants under a doxycycline-inducible promoter. (G) Plots showing percent GFPu remaining in the indicated cell lines after shutting off translation with emetine. (H) Scheme showing the labeling and visualization of newly synthesized proteins (NSPs) with azidohomoalanine (AHA) in UCH37KO cells expressing either UCH37I216E or UCH37F117A. (I) Fluorescent SDS-PAGE analysis of the turnover of AHA-labeled NSPs in UCH37KO cells expressing either UCH37I216E or UCH37F117A. (J) Quantitation of the results shown in (I).
-
Figure 6—source data 1
Emetine chase analysis and AHA pulse chase analysis of WT, UCH37KO and UCH37 mutants (F117A or I216E) transduced cells.
- https://cdn.elifesciences.org/articles/76100/elife-76100-fig6-data1-v1.xlsx
-
Figure 6—source data 2
Uncropped gel images of gel-based branched tri-Ub kinetic assay, HMW Ub chain cleavage analysis, Western blot analysis of in vitro Ptsm degradation assay, and fluorescent gel analysis of AHA pulse chase assay.
- https://cdn.elifesciences.org/articles/76100/elife-76100-fig6-data2-v1.pdf
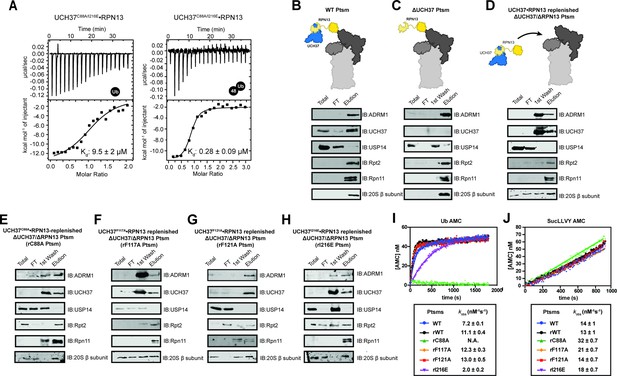
Backside mutants impair K48 chain debranching and UCH37-dependent proteasomal degradation.
(A) Isothermal titration calorimetry analyses of mono-ubiquitin (Ub) and K48 di-Ub binding to UCH37C88A/I216E•RPN13. (B–H) Western blot analyses of the purification of different 26 S Ptsm complexes with and without UCH37 variants. (I) Hydrolysis of Ub-AMC by the indicated 26 S Ptsm complexes. (J) Hydrolysis of suc-LLVY-AMC by the indicated 26 S Ptsm complexes.
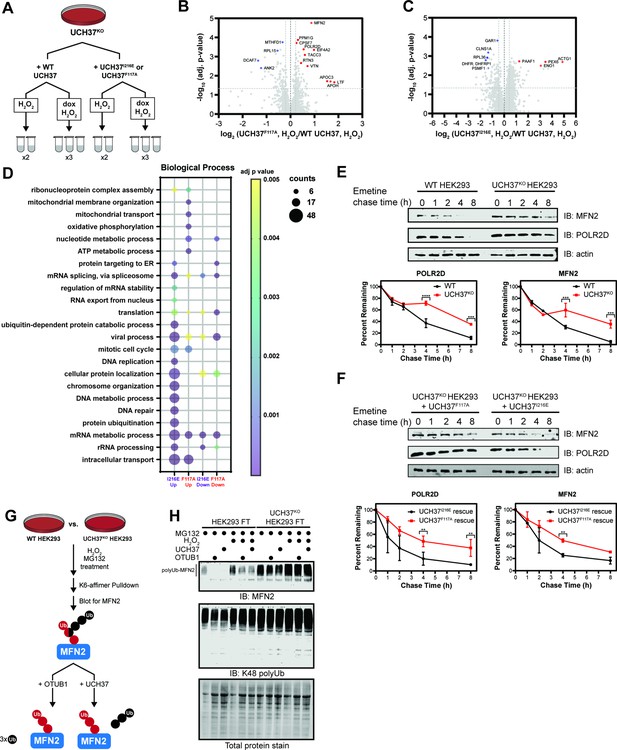
Identifying proteins dependent on the K48 chain binding and debranching activity of UCH37 for degradation.
(A) Scheme for tandem mass tagging (TMT)-based proteomics of UCH37KO cells expressing either wild-type (WT) UCH37, UCH37I216E, or UCH37F117A. (B–C) Volcano plots comparing the proteomes of UCH37F117A- and WT UCH37-expressing UCH37KO cells (B), and UCH37I216E- and WT UCH37-expressing UCH37KO cells. (C) In both cases, the cells are treated with H2O2 to induce oxidative stress. (D) Biological process analysis of proteins upregulated in UCH37F117A- and UCH37I216E-expressing UCH37KO cells. (E) Western blot analysis of the turnover of POLR2D and mitofusin-2 (MFN2) after shutting off translation with emetine in WT and UCH37KO HEK293 cells. (F) Western blot analysis of the turnover of POLR2D and MFN2 after shutting off translation with emetine in UCH37KO cells reconstituted with either UCH37F117A or UCH37I216E. Immunoblotting with α-POLR2D, α-MFN2, and α-actin antibodies. (G) Scheme showing the enrichment and analysis K6-linked ubiquitin chains. (H) Western blot analysis of K6-affimer-enriched ubiquitinated species from WT and UCH37KO HEK293 cells. The enriched conjugates were treated with OTUB1 (1 µM) or UCH37•RPN13 (2 µM) at rt for 1 hr. Immunoblotting with α-MFN2 and α-K48-linkage specific antibodies.
-
Figure 7—source data 1
Tandem mass tagging proteomics analysis of WT, UCH37KO and UCH37 mutants (F117A or I216E) transduced cells.
- https://cdn.elifesciences.org/articles/76100/elife-76100-fig7-data1-v1.xlsx
-
Figure 7—source data 2
Uncropped gel images of Western blot analysis of emetine chase and K6-affimer enrichment pulldown assay.
- https://cdn.elifesciences.org/articles/76100/elife-76100-fig7-data2-v1.pdf
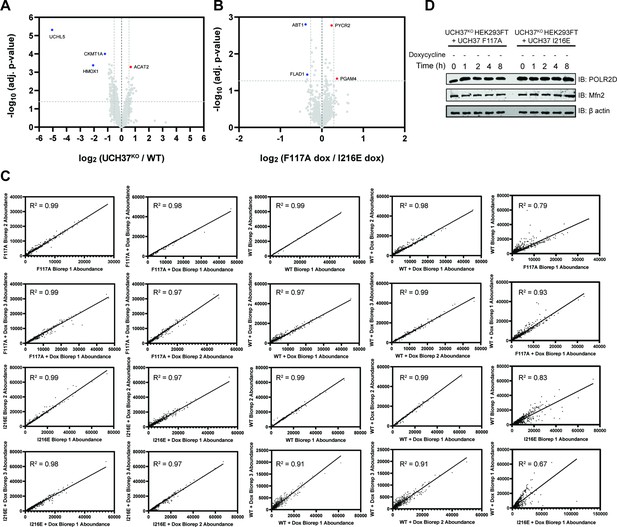
Identifying proteins dependent on the K48 chain binding and debranching activity of UCH37 for degradation.
(A) Volcano plot comparing the proteomes of untreated wild-type (WT) HEK293 and UCH37KO cells. (B) Volcano plot comparing the proteomes of untreated UCH37F117A- and UCH37I216E-expressing UCH37KO cells. (C) Pearson’s correlation analysis of biological replicates of each cell line and treatment. (D) Western blot analysis of translation shutoff experiments performed with UCH37F117A- and UCH37I216E-expressing UCH37KO cells in the absence of doxycycline.
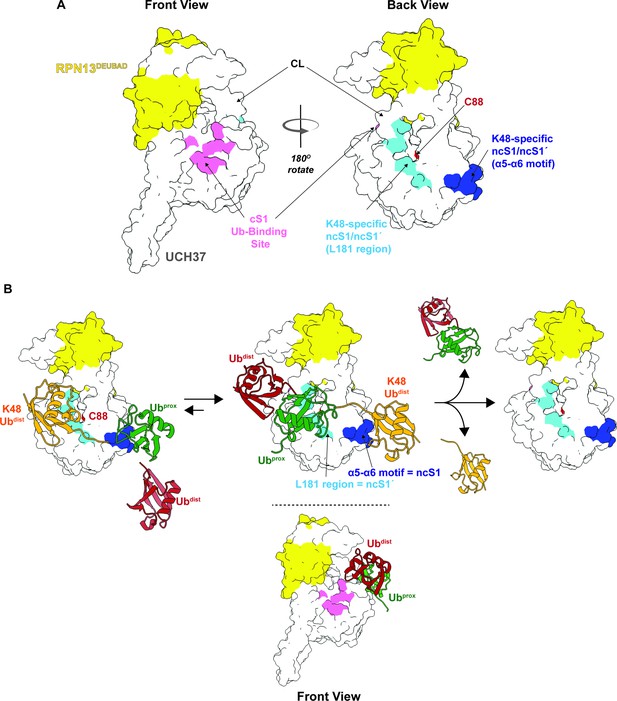
Proposed debranching model.
(A) Surface depiction of UCH37 showing the canonical S1 (cS1) ubiquitin (Ub)-binding site and the new K48-specific binding sites identified in this study. (B) Proposed mechanism for chain debranching using the K48-specific binding sites. The K48-linked portion of a branched chain engages the K48-specific Ub-binding sites in two different orientations: one with the proximal Ub (Ubprox) docked at the α5–6 motif and the other with the distal Ub (Ubdist) bound to that site. As the docking models show (Figure 4H), the K48 isopeptide bond is less obstructed and closer to the catalytic C88 residue when the K48 Ubdist moiety is bound to α5–6 and Ubprox is bound near the L181 region. In this orientation, the other Ubdist at the branchpoint is positioned near the frontside of the enzyme. With K48 isopeptide bond cleavage occurring on the backside, the α5–6 motif would serve as the noncanonical S1 (ncS1) site and the L181 region would be the ncS1´ site.
Tables
Active and passive restraints used for HADDOCK modeling.
UCH37 | DEUBAD | |
---|---|---|
Active residues | 117, 121, 154 | |
Passive residues | 81, 82, 83, 84, 85, 118, 119, 120, 122, 123, 124, 125, 126, 127, 128, 155, 156, 157, 158, 159, 160, 161, 162, 181, 182, 183, 184 | 403, 406, 410, 419, 422 |
Flexible residues | 329–347, 444–468 | |
Ub2K48-proximal subunit | Ub2K48-proximal subunit | |
Active residues | 8,44, 70 | 8,44 |
Passive residues | 36, 42, 43, 45, 46, 49, 50, 57, 58, 59, 60, 68, 71, 72, 73, 74, 75, 76 | 42, 43, 45, 46, 47, 48, 49, 50, 57, 58, 59, 60, 68, 70, 71 |
Flexible residues | 48 | 72–76 |
Additional files
-
Transparent reporting form
- https://cdn.elifesciences.org/articles/76100/elife-76100-transrepform1-v1.docx
-
Source data 1
Tables of ITC, SEC-MALs, FP, Ub-AMC kinetics, SucLLVY, emetine chase, AHA pulse chase and TMT correlation analysis.
- https://cdn.elifesciences.org/articles/76100/elife-76100-data1-v1.xlsx
-
Source data 2
Hydrogen Deuterium Exchange Mass Spectrometry analysis of UCH37 and RPN13DEUBAD.
- https://cdn.elifesciences.org/articles/76100/elife-76100-data2-v1.xlsx
-
Source data 3
Tandem Mass Tagging proteomics analysis.
- https://cdn.elifesciences.org/articles/76100/elife-76100-data3-v1.xlsx
-
Source data 4
Uncropped gel images of Western blot analysis and gel based kinetic analysis.
- https://cdn.elifesciences.org/articles/76100/elife-76100-data4-v1.pdf
-
Source data 5
Full NMR spectra of mono-Ub and K48 di-Ub in presence and absence of UCH37.
- https://cdn.elifesciences.org/articles/76100/elife-76100-data5-v1.pdf