Dilated cardiomyopathy mutation E525K in human beta-cardiac myosin stabilizes the interacting-heads motif and super-relaxed state of myosin
Figures
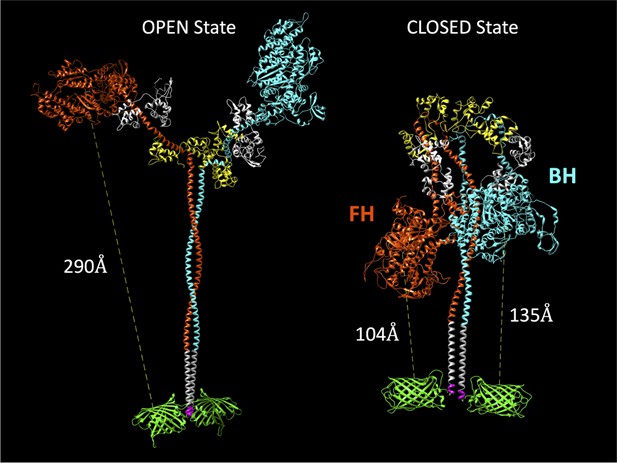
Molecular models of the M2β 15HPZ.GFP construct in open and closed (interacting-heads motif [IHM]) states.
From proximal to distal, the model contains myosin heavy chains (orange and cyan), each with one essential light chain (white) and one regulatory light chain (yellow). Following the last residue of the 15 heptad tail (E946), the leucine zipper (PDB: 1ZIL, gray) helps to dimerize the construct and is followed by a short, flexible glycine linker (pink), and then the C-terminal GFP molecule (PDB: 1GFL, green). Distances in the figure are measured from Y66 within the tripeptide fluorophore of GFP to S180 in the ATP-binding site of the myosin motor domain. Models were constructed by joining existing pdb files in UCSF Chimera software. The open state is based on a previously published model by the Spudich lab (Trivedi et al., 2018), while the closed state is based on the 5TBY cardiac homology model from the Padrón lab (Alamo et al., 2017). In the closed state, the free head (FH) of myosin lies closer to the C-terminal GFP molecules, as compared to the blocked head (BH).
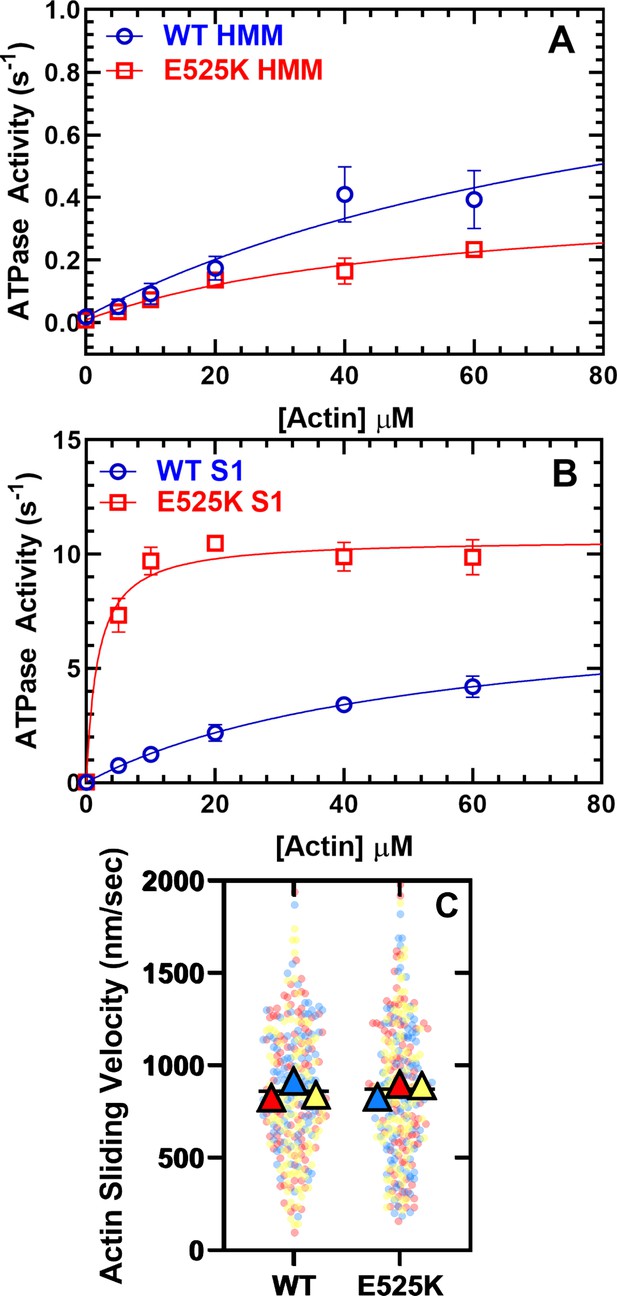
Steady-state actin-activated ATPase activity and in vitro motility.
(A) The ATPase activity of WT and E525K M2β 15HPZ.GFP (heavy meromyosin [HMM]) was measured as a function of actin concentration, and the data were fit to a hyperbolic function to determine kcat and KATPase. (B) The ATPase activity of WT and E525K M2β S1 (S1) was also measured as in panel A. (C) In vitro motility assays were used to examine actin gliding velocities of HMM constructs. The individual data points are plotted for three protein preparations, shown in three different colors (yellow, blue, and red), and the average for each preparation is represented by the colored triangles. The overall average velocity is represented by the black line. Measurements are from at least three protein preparations (± SD). See Table 1 for summary of values and statistical comparisons.
-
Figure 2—source data 1
Excel files with data from Figure 2.
- https://cdn.elifesciences.org/articles/77415/elife-77415-fig2-data1-v1.zip
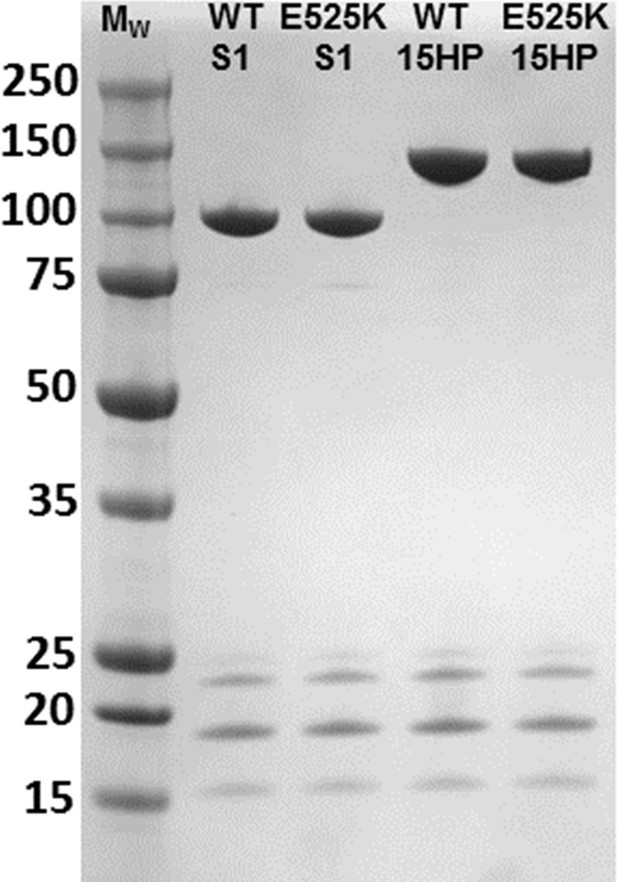
SDS-gel of M2β S1 and 15HPZ.
GFP preparations. Purified myosin was run on a 4–15% SDS-gel at a concentration of 1 µM (2.76 µg for M2β-S1 and 3.62 µg for M2β 15HPZ.GFP). The higher molecular weight bands are the human recombinant myosin heavy chains (predicted Mw of M2β S1=99,022 Da and M2β 15HPZ.GFP=140,674 Da), and the lower molecular weight bands are the mixture of endogenous mouse myosin light chains (between 15 and 25 kDa) that co-purify with M2β. We identified the myosin light chains in the S1 prep by mass spectrometry in a previous publication (Swenson et al., 2017).
-
Figure 2—figure supplement 1—source data 1
Uncropped SDS-PAGE of WT and E525K S1 and 15HPZ.GFP.
- https://cdn.elifesciences.org/articles/77415/elife-77415-fig2-figsupp1-data1-v1.zip
In vitro motility assay.
Representative movies of actin gliding generated by WT and E525K M2β 15HPZ.GFP. Myosin was directly attached to the nitrocellulose motility surface and Alexa-555 labeled actin was visualized by time lapsed microscopy (1 frame per second for 2 min). Images were exported to ImageJ to generate videos.
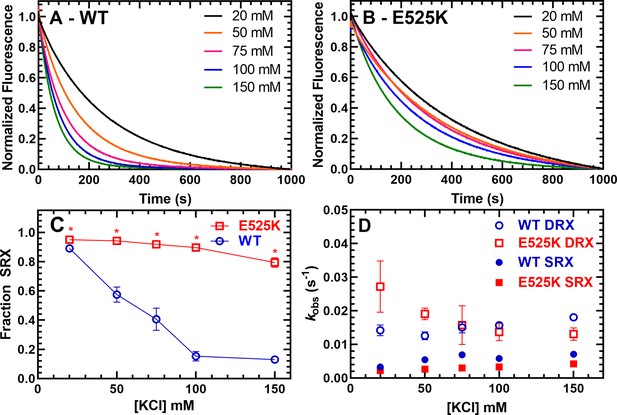
Single ATP turnover measurements.
The turnover of mantATP by M2β 15HPZ.GFP was examined at varying KCl concentrations. The fluorescence transients from (A) WT or (B) E525K M2β 15HPZ.GFP (0.25 µM) that was pre-incubated with mantATP (1 µM) for ~30 s and then mixed with saturating unlabeled ATP (2 mM) were observed for 1000 s. The mant fluorescence transients were best fit to a two-exponential function. (C) The relative amplitude of the slow rate constants from the fluorescence transients was used to determine the fraction of heads in the super-relaxed (SRX) state. The mutant had a significantly greater SRX state fraction than WT at all KCl concentrations. (D) The slow-rate constants (SRX state) were 5–10 times slower than the fast-rate constants (disordered relaxed [DRX] state), and relatively similar (~within twofold) at each KCl concentration for both the WT and E525K constructs. Error bars are ± SD, N=3 separate protein preparations with one technical replicate per prep (*p<0.005, comparing WT and E525K, unpaired Student’s t-test). See Table 2 for summary of values.
-
Figure 3—source data 1
Excel files with data from Figure 3.
- https://cdn.elifesciences.org/articles/77415/elife-77415-fig3-data1-v1.zip
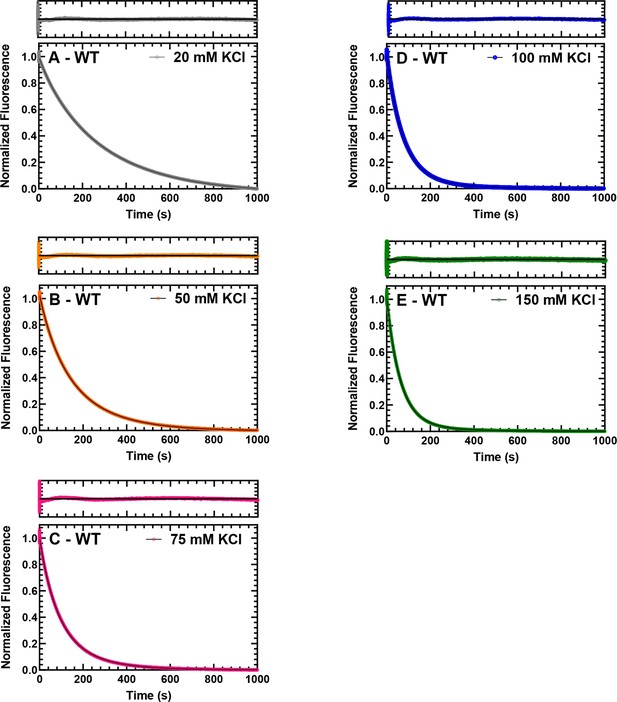
Single turnover with WT M2β 15HPZ.
GFP. Representative data from single mantATP turnover measurements that are presented in Figure 3A of the main text. The fluorescence transients and the fit of the transients to a two-exponential function at 20, 50, 75, 100, and 150 mM KCl are shown for WT M2β 15HPZ.GFP in panels A, B, C, D, and E, respectively. The residuals are shown above each plot.
-
Figure 3—figure supplement 1—source data 1
Excel files with data from Figure 3—figure supplement 1.
- https://cdn.elifesciences.org/articles/77415/elife-77415-fig3-figsupp1-data1-v1.zip
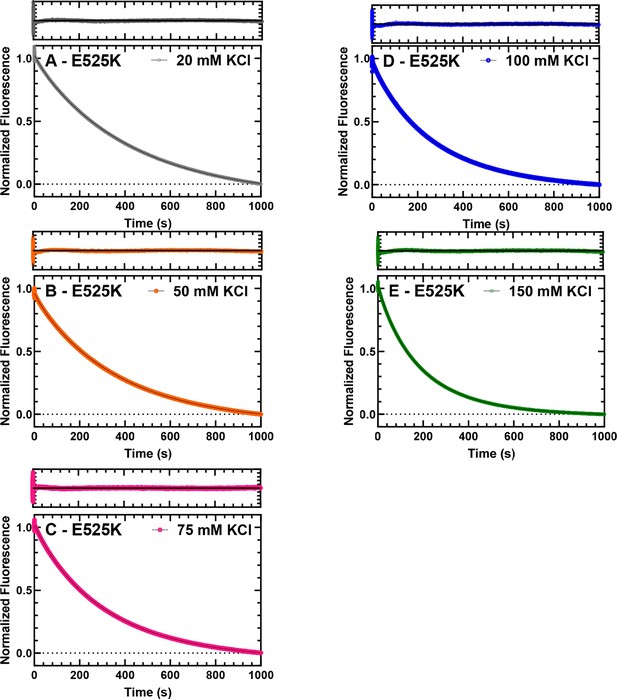
Single turnover with E525K M2β 15HPZ.
GFP. Representative data from single mantATP turnover measurements that are presented in Figure 3B of the main text. The fluorescence transients and the fit of the transients to a two-exponential function at 20, 50, 75, 100, and 150 mM KCl are shown for E525K M2β 15HPZ.GFP in panels A, B, C, D, and E, respectively. The residuals are shown above each plot.
-
Figure 3—figure supplement 2—source data 1
Excel files with data from Figure 3—figure supplement 2.
- https://cdn.elifesciences.org/articles/77415/elife-77415-fig3-figsupp2-data1-v1.zip
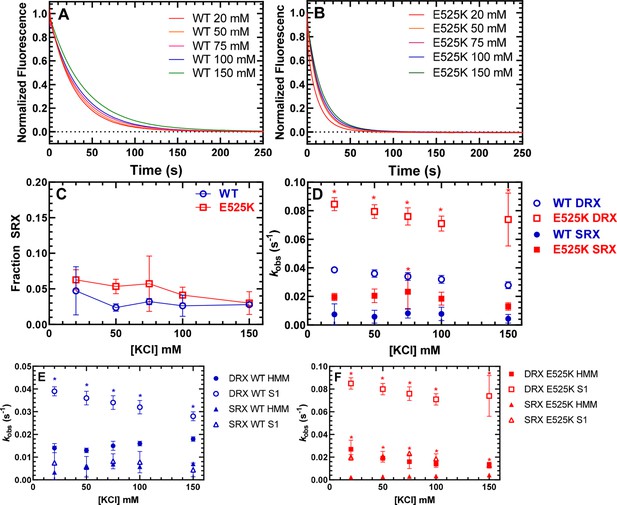
Single ATP turnover measurements with M2β S1.
The turnover of mantATP by M2β S1 WT (A) or E525K (B) was examined at varying KCl concentrations as in Figure 3. The mant fluorescence transients were best fit to a three-exponential function. There was a minor very fast phase (~5% of the signal), which can be attributed to a small amount of mantADP release from S1 (2–4 s–1) and therefore was not included in the analysis of the other two rate constants. (C) The relative amplitude of the slow-rate constant was used to determine the fraction of heads in the super-relaxed (SRX) state. (D) The slow rate constant (SRX state) was mostly similar in WT S1 and E525K S1 while the predominant rate constant (disordered relaxed [DRX] state), which dominated the fluorescence transients (90–95% of the signal), was significantly higher in E525K compared to WT. (E) The DRX state rate constants were faster in WT S1 compared to WT heavy meromyosin (HMM), while the SRX state rate constant was similar. (F) Both the DRX and the SRX rate constants were significantly faster in E525K S1 compared to E525K HMM. All rate constants were relatively insensitive to KCl concentration (see Tables 2 and 3 for summary of values; *p<0.005, unpaired Student’s t-test).
-
Figure 3—figure supplement 3—source data 1
Excel files with data from Figure 3—figure supplement 3.
- https://cdn.elifesciences.org/articles/77415/elife-77415-fig3-figsupp3-data1-v1.zip
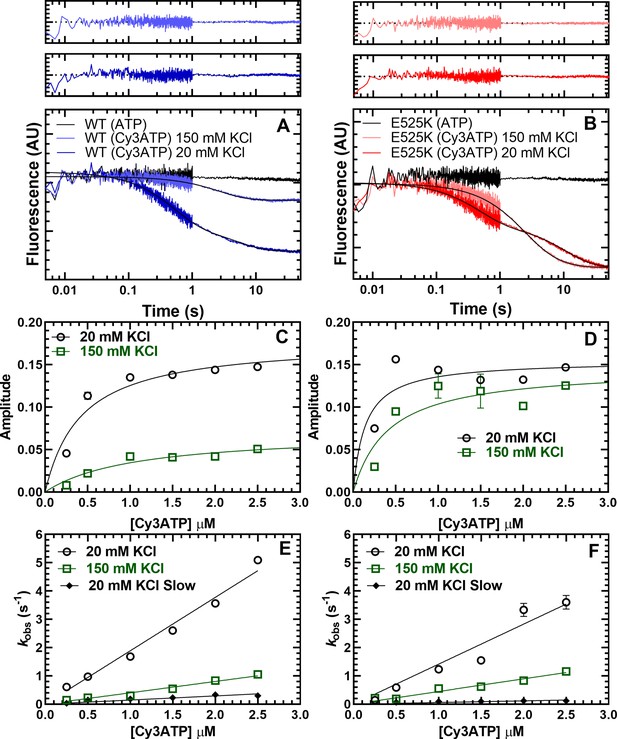
Cy3ATP binding to M2β 15HPZ.GFP monitored by FRET.
GFP fluorescence (0.25 µM; donor) quenching was examined upon Cy3ATP (1 µM; acceptor) binding to WT (A) or E525K (B) M2β 15HPZ.GFP. The fluorescence transients were best fit to a double-exponential function at low salt (20 mM KCl) and a single or double exponential function at high salt (150 mM KCl; fast phase was 65% and 25% of the signal at low salt in WT and E525K, respectively). Residuals are shown above each plot. The total amplitude of the fluorescence change was plotted as a function of Cy3ATP concentration in WT (C) and E525K (D). All rate constants were linearly dependent on Cy3ATP concentration in both WT (E) and E525K (F) M2β 15HPZ.GFP (see Table 4 for summary of linear fits in panel E and F). Each data point in C–F represents 2–3 replicates from a single preparation of myosin, and errors bars indicate ± SE of the fit.
-
Figure 4—source data 1
Excel files with data from Figure 4.
- https://cdn.elifesciences.org/articles/77415/elife-77415-fig4-data1-v1.zip
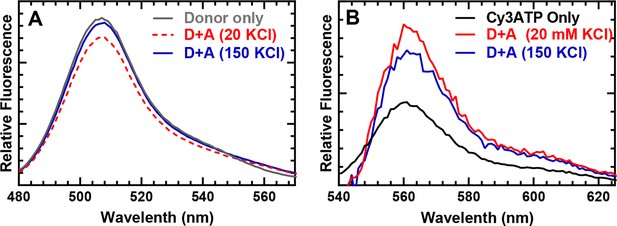
Steady-state FRET spectra.
Fluorescence emission spectra of WT M2β–15HPZ.GFP in the presence and absence of acceptor (Cy3ATP) were examined by exciting the sample at 470 nm and measuring the emission from 480 to 625 nm. (A) Fluorescence spectra of the donor highlight the donor quenching in the presence of Cy3ATP which was reduced at higher salt. (B) Fluorescence spectra of the acceptor, after subtracting donor fluorescence, highlight the acceptor enhancement in low- and high-salt conditions. Cy3ATP alone (absence of myosin) is shown for comparison.
-
Figure 4—figure supplement 1—source data 1
Excel files with data from Figure 4—figure supplement 1.
- https://cdn.elifesciences.org/articles/77415/elife-77415-fig4-figsupp1-data1-v1.zip
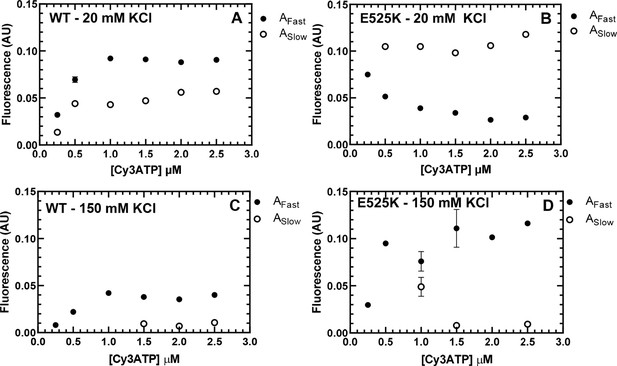
Amplitudes for Cy3ATP binding to M2β 15HPZ.
GFP. The amplitudes of the fast and slow components of the FRET transients examined in 20 mM (A and B) and 150 mM (C and D) KCl were plot as a function of Cy3ATP concentration (see Figure 4). Each data point represents a fluorescence transient (average of 2–3 replicates) that was fit to either a single or double exponential function (see source date for fit parameters). Error bars represent ± SEM.
-
Figure 4—figure supplement 2—source data 1
Excel files with data from Figure 4—figure supplement 2.
- https://cdn.elifesciences.org/articles/77415/elife-77415-fig4-figsupp2-data1-v1.zip
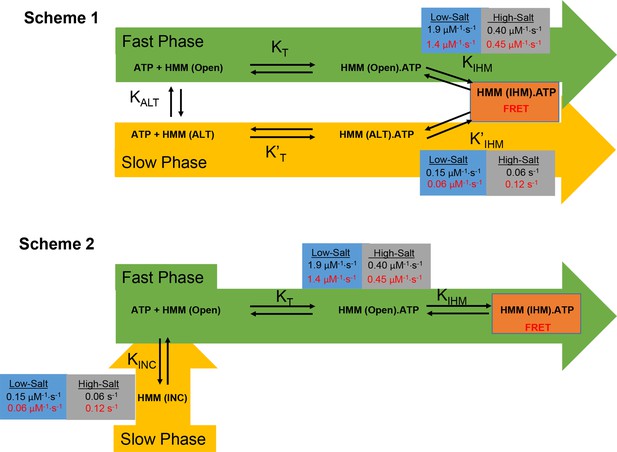
Kinetic models of interacting-heads motif (IHM) formation based on stopped-flow FRET.
Two kinetic schemes (Scheme 1 and 2) were considered for modeling the kinetics of IHM formation. In Scheme 1, we assumed the initial ATP-binding step (KT) was followed by transition into the IHM (KIHM) which was then detected by FRET. The model postulates that heavy meromyosin (HMM) can populate two conformations in the absence of nucleotide (Apo), one that is in the Open conformation and another in an Alternate (ALT) conformation. The top pathway (Fast Phase-green) represents ATP binding to the Open state followed by a favorable transition into the IHM in low salt. The lower pathway (Slow Phase-yellow) is only significantly populated at low salt and characterized by slower ATP binding to the ALT conformation and a favorable transition into the IHM. In Scheme 2, the slow phase is caused by a conformation that is incompetent to bind nucleotide (INC) and in equilibrium with the Open conformation. In both models, in the absence of nucleotide (Apo) in low-salt conditions, the E525K mutant favors the ALT or INC conformation, while WT favors the open conformation. Also, both models predict that in high-salt conditions, the rate of transition into the IHM slows for both WT and mutant, and it is unfavorable for WT but E525K still favors the IHM. Rate constants for WT are in black and E525K in red (see Table 4 for summary of rate constants).
-
Figure 4—figure supplement 3—source data 1
Excel files with data from Figure 4—figure supplement 3.
- https://cdn.elifesciences.org/articles/77415/elife-77415-fig4-figsupp3-data1-v1.zip
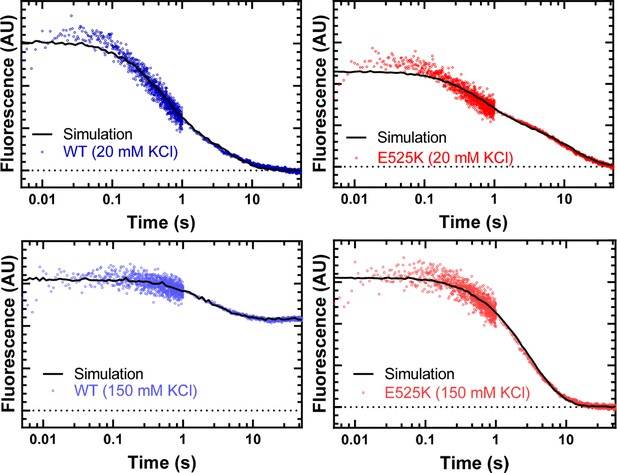
FRET transients fit to the proposed kinetic model.
The fluorescence transients from the FRET experiments are fit to the kinetic model described in Figure 4—figure supplement 3. The values of the rate and equilibrium constants are described in the legend of Figure 4—figure supplement 3. The ATP-binding rates determined from the linear fit of the rate constants as a function of Cy3ATP concentration were held constant (k+T and k’+T µM–1∙s–1) (see Table 4) and the rate and equilibrium constants for the transition into the interacting-heads motif (IHM) (KIHM and K’IHM) we allowed to float to best fit the transients. We conclude that under the conditions of the experiments, the FRET was limited by the rate of ATP binding, and thus, the transition into the IHM was ≥5 s–1.
-
Figure 4—figure supplement 4—source data 1
Excel files with data from Figure 4—figure supplement 4.
- https://cdn.elifesciences.org/articles/77415/elife-77415-fig4-figsupp4-data1-v1.zip
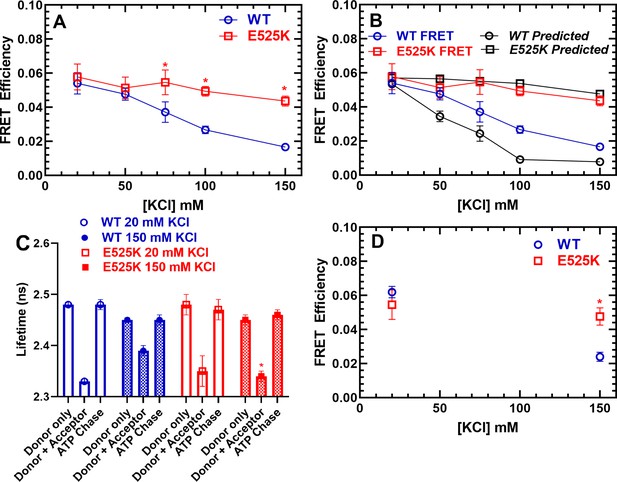
FRET monitors stability of the closed (interacting-heads motif [IHM]) conformation.
Steady-state FRET between the C-terminal GFP tag and Cy3ATP bound to the motor domain was examined in the stopped-flow as a function of KCl concentration. (A) The average FRET efficiency (± SD) was examined in three separate protein preparations. (B) The FRET efficiency in panel A was compared to the predicted FRET efficiency, based on the single turnover measurements in Figure 3. Error bars in panels A and B are ± SD, N=3 separate protein preparations with three technical replicates per prep. (C) Time-resolved FRET was examined by monitoring the GFP fluorescence lifetime in the presence and absence of Cy3ATP. Summary of fluorescence lifetime data with WT and E525K M2β 15HPZ.GFP. Adding saturating ATP to the sample containing WT M2β 15HPZ.GFP and Cy3ATP (ATP chase) was used to rule out donor fluorescence changes in the presence of ATP. (D) Time-resolved FRET (three technical replicates performed with a single protein preparation) demonstrated similar results to steady-state FRET shown in panel A (*p<0.05 comparing WT and E525K, unpaired Student’s t-test).
-
Figure 5—source data 1
Excel files with data from Figure 5.
- https://cdn.elifesciences.org/articles/77415/elife-77415-fig5-data1-v1.zip
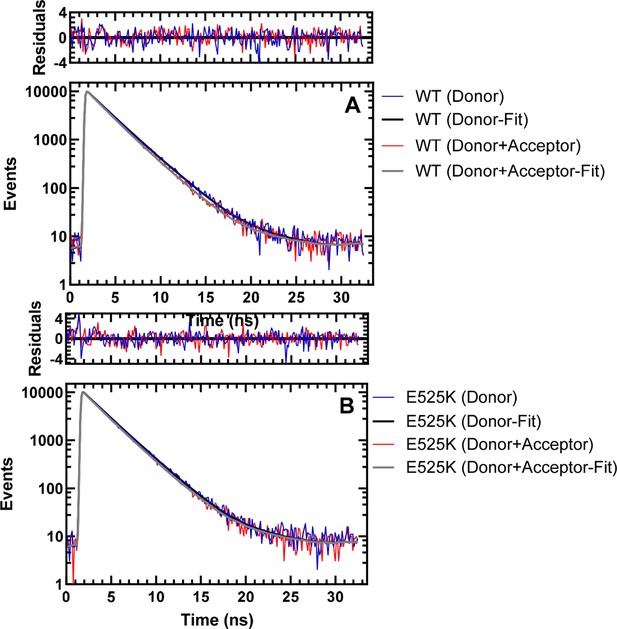
Representative fluorescence lifetime decays for TR-FRET analysis.
Fluorescence lifetime decays were collected by exciting the donor (GFP) using a 479 nm picosecond laser and examining donor fluorescence emission (515 long-pass filter) in the presence and absence of acceptor (Cy3ATP). Time-correlated single photon counting was used to collect lifetime decays. The donor only (60 nM M2β 15HPZ.GFP) is compared to the donor+acceptor (60 nM M2β 15HPZ.GFP+0.1 µM Cy3ATP) in 20 mM KCl conditions in WT (panel A) and E525K (panel B) M2β 15 HZ.GFP. The residuals are shown above the fits. The decays were fit well to a two-exponential function to determine the average lifetime (see Figure 5C for summary of average lifetime decay values and source data for all lifetime decay fit parameters).
-
Figure 5—figure supplement 1—source data 1
Excel files with data from Figure 5—figure supplement 1.
- https://cdn.elifesciences.org/articles/77415/elife-77415-fig5-figsupp1-data1-v1.zip
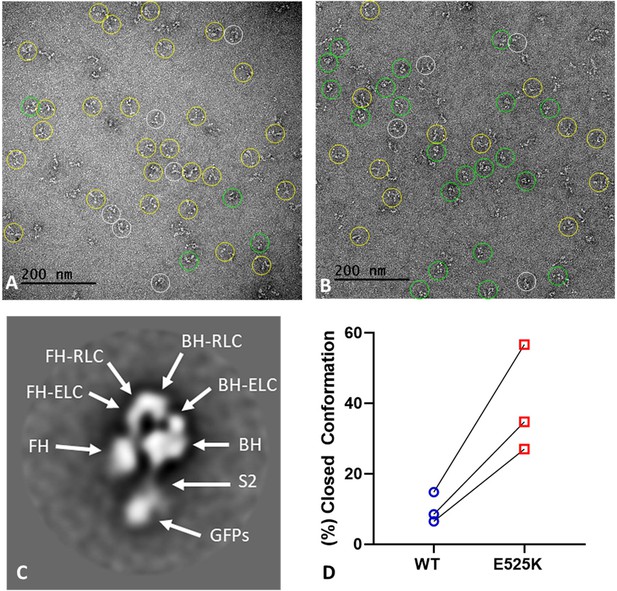
EM structure of WT and E525K constructs.
(A and B) Negative stain images of WT and E525K, respectively, showing the conformational state of the molecules (yellow – open; green – closed; white circle – not clear/partially folded). (C) 2D class average of E525K, showing subdomains (Figure 1, ‘closed’). (D) Results of three biological replicate experiments comparing % of closed (interacting-heads motif [IHM]) conformations in WT and E525K. We attribute the variable results to unavoidable differences in grid surface characteristics on the different experimental days. Despite these differences, the same trend occurred in each experiment, with E525K showing 3-4 times as many IHMs as WT (see Table 5 for summary, N=250-300 molecules per preparation).
-
Figure 6—source data 1
Electron micrographs used for classifying molecules in the folded-back interacting-heads motif (IHM) or open conformation .
- https://cdn.elifesciences.org/articles/77415/elife-77415-fig6-data1-v1.zip
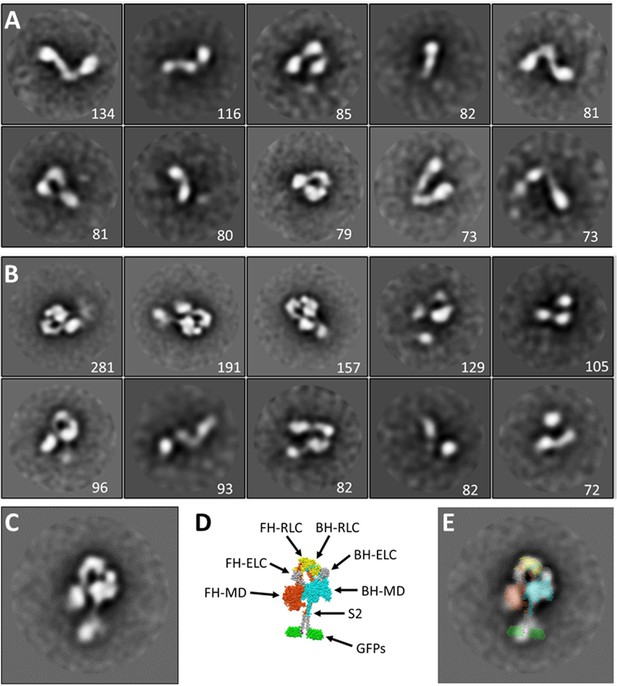
2D class averaging of M2β 15HPZ.
GFP negative stain images. (A) WT and (B) E525K. The numeral in each panel indicates the number of particles contributing to the particular class average. (C–E) Superposition of M2β 15HPZ.GFP atomic model on E525K class average. (C) Class average. (D) Atomic model based on 5tby (Figure 1). (E) Superposition, demonstrating good agreement between EM imaging and model, supporting our identification of the molecules as bona fide interacting-heads motifs (IHMs).
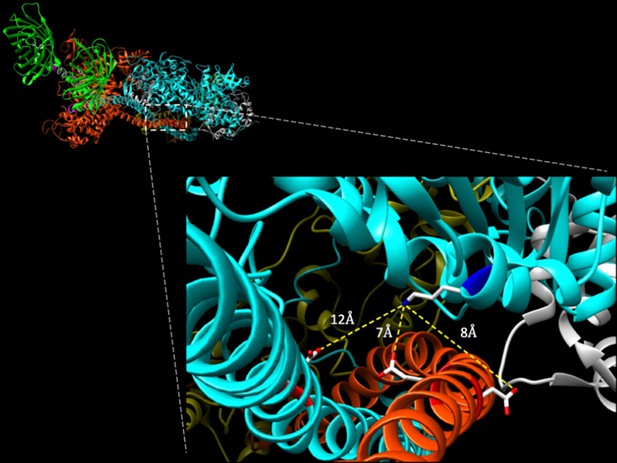
Potential intramolecular interactions of E525K with S2.
Top left: oblique view of folded myosin hexamer from the C-terminal end. Note C-terminal GFP molecules for orientation of molecule with blocked head (BH, cyan) and free head (FH, orange) of myosin. Inset: potential intramolecular interactions between the mutated 525 K residue (blue, positively charged amine group) on BH of myosin with nearby acidic residues on S2 (red, negatively charged carboxylic acids) of both BH and FH. Highlighted residues include E902 on S2 of BH and E894 and D896 on S2 of FH. Intramolecular interactions were mapped using UCSF Chimera on same closed structure (5TBY template) described in Figure 1 (Alamo et al., 2017; Pettersen et al., 2004).
Tables
Summary of steady-state ATPase and in vitro motility measurements (± SE).
Parameter | WTM2β 15HPZ.GFP (N=3–5) | E525KM2β 15HPZ.GFP (N=3) | WTM2β S1(N=3–6) | E525KM2β S1(N=3) |
---|---|---|---|---|
v0 (s–1) | 0.02±0.01, N=5 | 0.01±0.01 | 0.02±0.01, N=6 | 0.03±0.01 |
kcat (s–1) | 1.1±0.49, N=5 | 0.41±0.09 | *7.8±0.7, N=6 | †10.6±0.3 |
KATPase (µM) | 99.9±64.5, N=5 | 53.3±20.8 | 52.2±8.6, N=6 | †1.8±0.4 |
Average sliding velocity (nm/s) | 853±54, N=3 | 852±58 | *, ‡1591±16, N=3 | N.D. |
Percent stuck filaments | 27.8±9.5, N=3 | 34.8±5.4 | ≤5, N=3 | N.D. |
-
*
p<0.005 comparing WT M2β S1 to WT M2β 15HPZ.GFP.
-
†
p<0.05 comparing E525K M2β S1 to WT M2β S1 (unpaired Student’s t-test).
-
‡
Data is from Tang et al., 2021.
Summary of single mantATP turnover measurements for WT and E525K M2β 15HPZ.GFP (N=3,± SD).
Conditions | Disordered relaxed (DRX) rate | DRX fraction | Super-relaxed (SRX) rate | SRX fraction |
---|---|---|---|---|
WT | ||||
20 mM KCl | 0.014±0.002 | 0.11±0.01 | 0.0033±0.0001 | 0.89±0.01 |
50 mM KCl | 0.013±0.001 | 0.43±0.05 | 0.0054±0.0005 | 0.57±0.05 |
75 mM KCl | 0.015±0.002 | 0.59±0.08 | 0.0069±0.0009 | 0.41±0.08 |
100 mM KCl | 0.016±0.001 | 0.85±0.03 | 0.0058±0.0004 | 0.15±0.03 |
150 mM KCl | 0.018±0.001 | 0.87±0.02 | 0.0071±0.0008 | 0.13±0.02 |
E525K | ||||
20 mM KCl | 0.027±0.008 | 0.05±0.01 | 0.0022±0.0002 | 0.95±0.01 |
50 mM KCl | 0.019±0.002 | 0.06±0.01 | 0.0026±0.0001 | 0.94±0.01 |
75 mM KCl | 0.016±0.006 | 0.08±0.02 | 0.0030±0.0001 | 0.92±0.02 |
100 mM KCl | 0.014±0.003 | 0.10±0.02 | 0.0033±0.0001 | 0.90±0.02 |
150 mM KCl | 0.013±0.002 | 0.21±0.03 | 0.0042±0.0001 | 0.79±0.03 |
Summary of single mantATP turnover measurements for WT and E525K M2β S1 (N=3, ± SD).
Conditions | Disordered relaxed (DRX) rate | DRX fraction | Super-relaxed (SRX) rate | SRX fraction |
---|---|---|---|---|
WT | ||||
20 mM KCl | 0.039±0.002 | 0.95±0.03 | 0.0075±0.0074 | 0.05±0.03 |
50 mM KCl | 0.036±0.003 | 0.98±0.01 | 0.0058±0.0045 | 0.02±0.01 |
75 mM KCl | 0.034±0.003 | 0.97±0.01 | 0.0082±0.0033 | 0.03±0.01 |
100 mM KCl | 0.032±0.003 | 0.97±0.01 | 0.0078±0.0047 | 0.03±0.01 |
150 mM KCl | 0.028±0.002 | 0.97±0.01 | 0.0044±0.0030 | 0.03±0.01 |
E525K | ||||
20 mM KCl | 0.085±0.005 | 0.94±0.03 | 0.0197±0.0025 | 0.06±0.03 |
50 mM KCl | 0.080±0.005 | 0.95±0.01 | 0.0204±0.0048 | 0.05±0.01 |
75 mM KCl | 0.076±0.006 | 0.94±0.04 | 0.0233±0.0012 | 0.06±0.04 |
100 mM KCl | 0.071±0.005 | 0.96±0.01 | 0.0185±0.0045 | 0.04±0.01 |
150 mM KCl | 0.074±0.018 | 0.97±0.02 | 0.0129±0.0026 | 0.03±0.02 |
Summary of kinetic parameters from Cy3ATP binding experiments.
(N=1,± SE of the fit).
Parameter | WT | E525K |
---|---|---|
kfast (20 mM KCl) (µM–1 s–1) | 1.9±0.1 | 1.4±0.1 |
kslow (20 mM KCl) (µM–1 s–1) | 0.15±0.01 | 0.06±0.01 |
Afast (20 mM KCl) | 0.65±0.04 | 0.25±0.05 |
kfast (150 mM KCl) (µM–1 s–1) | 0.40±0.02 | 0.45±0.02 |
kslow (150 mM KCl) (s–1) | 0.06±0.03 | 0.12±0.06 |
Afast (150 mM KCl) | 0.81±0.03 | 0.89±0.16 |
Summary of negative stain EM images classified as open vs. closed conformation.
Preparation | WT(% closed) | WT(% open) | E525K(% closed) | E525K(% open) | % E525K closed/% WT closed |
---|---|---|---|---|---|
1 | 16.9 | 83.1 | 56.9 | 43.1 | 3.4 |
2 | 8.5 | 91.5 | 34.8 | 65.2 | 4.1 |
3 | 6.5 | 93.5 | 27.0 | 73.0 | 4.2 |