Glutathione in the nucleus accumbens regulates motivation to exert reward-incentivized effort
Figures
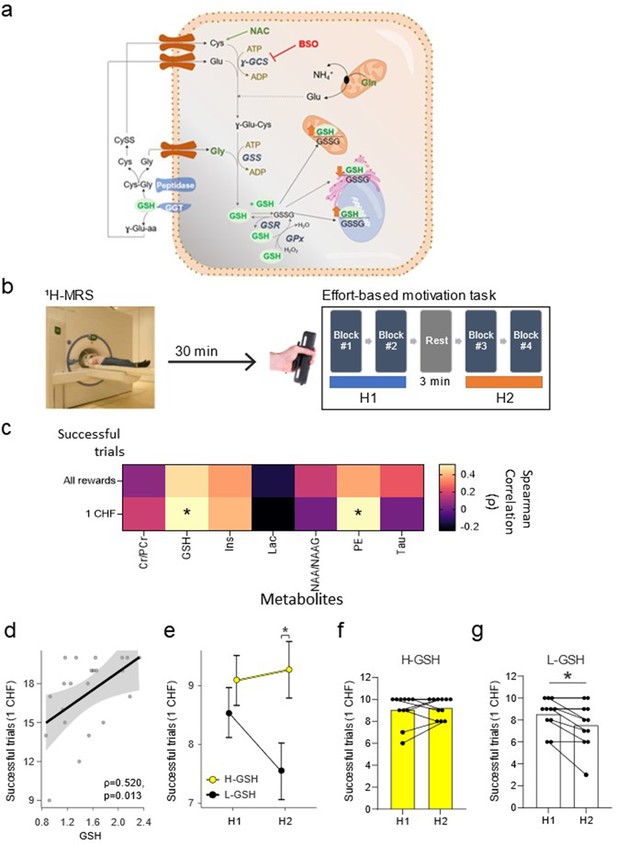
Glutathione (GSH) levels in the nucleus accumbens are associated with the number of successful trials on an effort-based motivation task.
(a) Schematic depiction of GSH metabolic pathways. GSH or γ-L-glutamyl-L-cysteinylglycine, is a tripeptide, that can exist in a reduced (GSH) or in an oxidized (Glutathione disulfide, GSSG) state. It is synthesized in the cytosol by an ATP-dependent two-step process catalyzed by γ-glutamyl cysteine synthase/ligase (γ-GCS) and GSH synthetase (GSS), with γ-GCS as the rate-limiting enzyme. GSH is freely distributed in the cytosol (up to 10 mM concentration) and compartmentalized in the nucleus, endoplasmic reticulum, and mitochondria (up to 15%). GSH participates in the neutralization of H2O2 in the cytosol in collaboration with the glutathione peroxidase (GPx) which takes hydrogens from two GSH molecules, converting one molecule of H2O2 into two of H2O and one of GSSG. Glutathione reductase (GSR) reduces GSSG back to GSH using NADPH as an electron donor. The main enzyme that initiates the process of GSH degradation is named glutamyl transpeptidase (GGT). This enzyme is localized in the plasma membrane, facing outward and acting on the extracellular pool of GSH released by the cells. The degradation of GSH by GGTs generates γ-glutamyl-aminoacids and cysteine-glycine dipeptides, which are subjected to the action of membrane-bound peptidase to yield cysteine (Cys) and glycine (Gly). Therefore, the major function of GGT is to recover GSH precursors from extracellular pools which can be taken up by cells and used for the new synthesis of GSH. N-acetyl-cysteine (NAC) is a donor of Cys (the rate-limiting substrate), and buthionine sulfoximine (BSO) is a specific inhibitor of γ-GCS. In this work, the systemic treatment of NAC in the drinking water was used to increase GSH levels in nucleus accumbens (NuAc) and the BSO intra-nucleus accumbens infusion to reduce GSH in NuAc. (b) Experimental paradigm depiction. The 1H-MRS nucleus accumbens scan precedes the effort-based motivation task, which is composed by two halves (H1 and H2), separated by a 3-min rest block. (c) Matrix showing all pairwise correlations between metabolites of interest and the number of successful trials in the entire task for all rewards and for 1 CHF rewards. (d) Detailed scatterplot for the correlation between GSH levels in the nucleus accumbens and the number of successful 1 CHF trials (ρ=0.52, p=0.01, corrected p=0.049). (e) Participants with lower GSH in the nucleus accumbens (L-GSH) show a lower number of successful 1 CHF trials in the second half of the experiment (H2) than those with higher nucleus accumbens GSH levels (H-GSH) (H-GSH=9.27 ± 0.91) successful 1 CHF trials wins, L-GSH=7.55 ± 2.07 successful 1 CHF trials wins; t(20) = −2.45, p=0.02, corrected p=0.04, Cohen’s d=1.08. This difference is not present in the first half (H1) (H-GSH=9.09 ± 1.36 successful 1 CHF trials wins, L-GSH=8.55 ± 1.44 successful 1 CHF trials wins; t(20) = −0.91, p=0. 24, corrected p=0.24, Cohen’s d=1.08). Participants with high GSH levels in the nucleus accumbens are able to maintain an elevated number of successful 1 CHF trials (f) while those with low GSH levels in the nucleus accumbens show a significant reduction of performance in H2 (g) (e–f: H-GSH: t(10) = 0.61, p=0.28, corrected p=0.28, Cohen’s d=0.19; L-GSH: Wilcoxon W=21.0, p=0.02, corrected p=0.03, rank biserial correlation = 1.00). Significance levels: * p<0.05. Spearman correlation coefficient (ρ). N=11/group. See Figure 1—figure supplement 1 and Figure 1—source data 1.
-
Figure 1—source data 1
Spectroscopy on human raw data and statistical analysis.
- https://cdn.elifesciences.org/articles/77791/elife-77791-fig1-data1-v1.xlsx
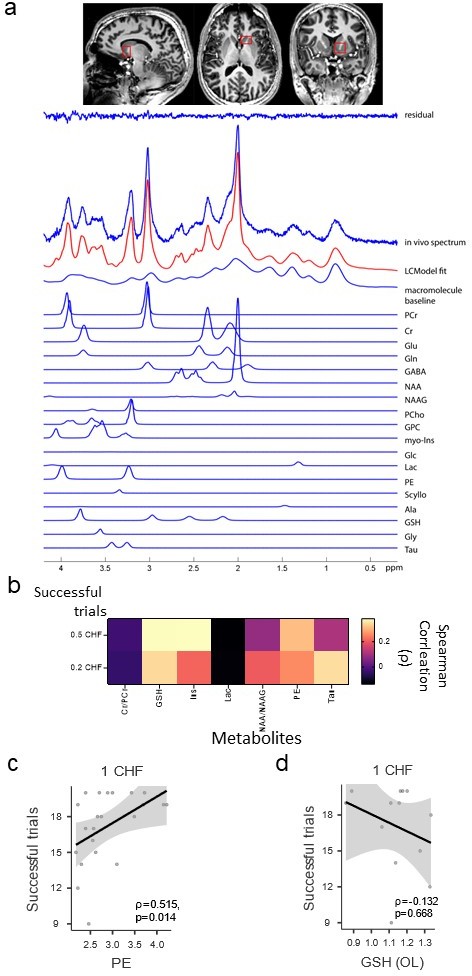
Glutathione (GSH) levels in the nucleus accumbens are not associated with the number of successful trials for lower rewards (0.5 CHF and 0.2 CHF).
(a) Region of interest in the human brain (i.e. nucleus accumbens) and examples of metabolites spectra. (b) Matrix showing all pairwise correlations between metabolites of interest and the number of successful trials in the entire task for 0.5 CHF and 0.2 CHF rewards (two-tailed p-values false discovery rate (FDR) corrected (Benjamini–Hochberg) for GSH/0.5 CHF p=0.29; GSH/0.2 CHF p=0.40). (c) Detailed scatterplot for the correlation between phosphatidylethanolamine (PE) levels in the nucleus accumbens and the number of successful 1 CHF trials (Spearman correlation ρ=0.52, p=0.01). (d) Detailed scatterplot for the correlation between GSH levels in the occipital lobe and the number of successful 1 CHF trials (Spearman correlation ρ=0.13, p=0.67; Bayesian correlation for the null hypothesis (H0: there is no positive correlation between occipital GSH levels and number of 1 CHF successful trials) yields a BF01=5.18).
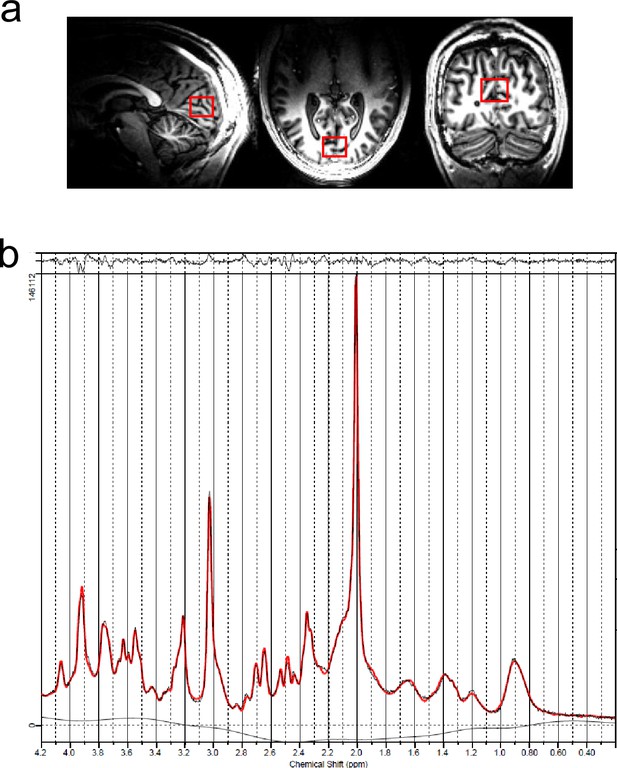
Representative scans and trace from the human occipital lobe.
(a) Region of interest in the human brain (occipital lobe) and (b) example of metabolite spectra in the same region.
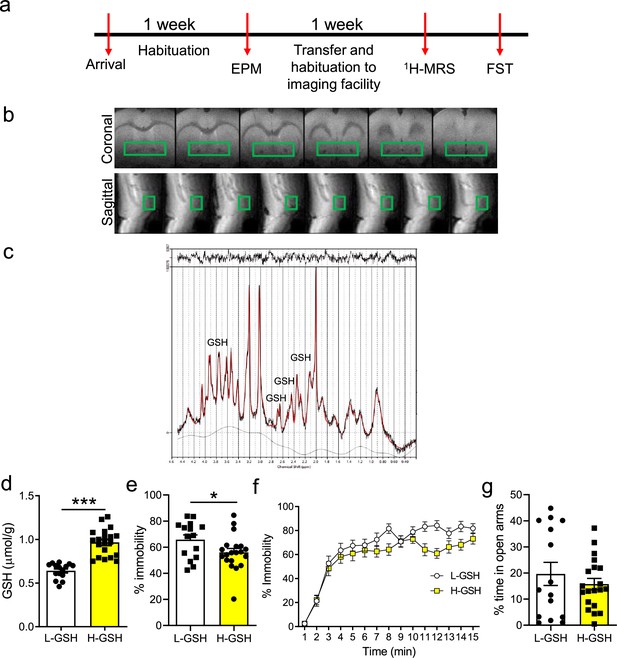
Rats differing in accumbal glutathione (GSH) levels show differences in immobility in the forced swim test (FST).
(a) Experimental design: Upon arrival to the facilities, rats were allowed to acclimatize to the conditions and were handled to habituate to the experimenters. Then they were tested in the elevated plus maze. Subsequently, they were transferred to the imaging facilities and after a further week of habituation, they were scanned in the proton magnetic resonance spectroscopy (1H-MRS) scanner. A few days after the scanning session, rats were exposed to a 15-min FST and subsequently euthanized. (b) Representative scans from the nucleus accumbens, of animals used in the FST. (c) Representative trace from the nucleus accumbens. (d) A median split was performed to separate rats into two groups, high-GSH (H-GSH) and low-GSH (L-GSH) (two-tailed t-test, t(33) = 7.27, p<0.001). (e) H-GSH rats spent less time immobile in the FST (two-tailed t-test, t(33) = 2.10, p=0.04). (f) When FST performance was analyzed in 1-min bins, two-way repeated measures ANOVA revealed a significant time × GSH interaction (F(14, 504)=2.10, p=0.01), a marginally non-significant effect of GSH (F(1,36)=3.66, p=0.06), and a significant effect of time ([F(36, 504)=11.87, P<0.001], N=16–20/group). (g) L-GSH and H-GSH did not show significant differences in anxiety (two-tailed t-test, t(33) = 0.85, p=0.40; Bayesian independent samples t-test for the null hypothesis (H0: there is no difference in the % of time in open arms between L-GSH and H-GSH groups) yields a BF01=2.30). See Figure 2—source data 1.
-
Figure 2—source data 1
Raw data Figure 2.
- https://cdn.elifesciences.org/articles/77791/elife-77791-fig2-data1-v1.xlsx
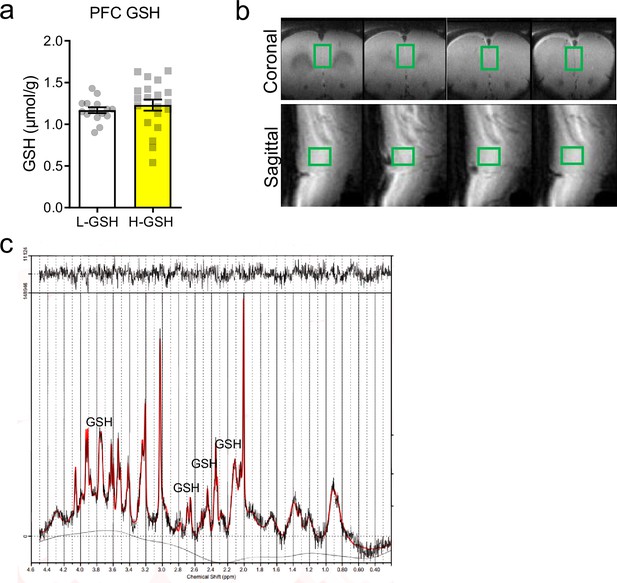
Rats differing in accumbal glutathione (GSH) levels show no significant differences in GSH levels in medial prefrontal cortex (mPFC).
(a) Plot showing GSH levels in the mPFC of low/high GSH (L/H-GSH) groups (median-split by GSH levels in the nucleus accumbens; unpaired two-tailed t-test, t(33) = 0.74, p=0.47; Bayesian independent samples t-test [H0: there is no difference in mPFC GSH levels between L and H-GSH groups] yields a BF01=2.47). (b) Representative scan images with the region of interest (ROI) corresponding to the mPFC in the coronal and sagittal sections. (c) Representative GSH trace from the mPFC.
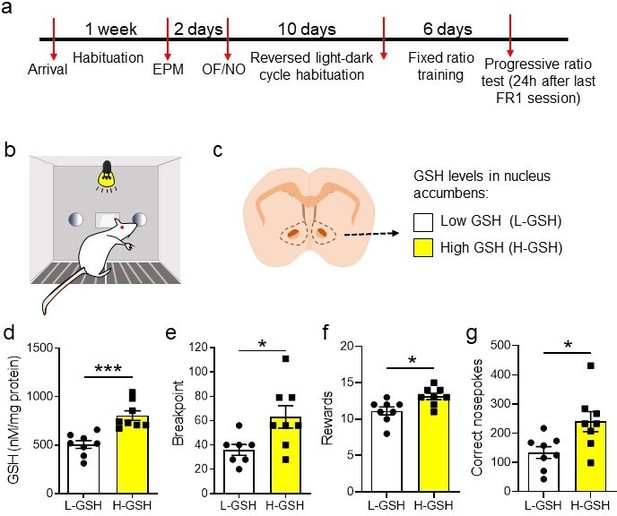
High glutathione (H-GSH) levels in nucleus accumbens are associated with an improvement in progressive ratio (PR) test performance.
(a) Timeline showing the experimental design. After initial acclimatization, handling, and behavioral characterization for anxiety and exploratory behavior, rats were placed in reversed light-dark cycle to habituate for 10 days. Subsequently, rats were trained in the fixed ratio 1 (FR1) paradigm for a total of six sessions (depicted in b). Once the PR test was performed, rats were euthanized, their nucleus accumbens was microdissected and snap frozen on liquid nitrogen. Accumbal GSH levels were measured using high-performance liquid chromatography (scheme depicted in c). (d) Rats were split into two groups based on their accumbal GSH levels (H-GSH and low GSH [L-GSH]), using a median split (t(14) = 4.69, p<0.001) In the PR test, H-GSH rats reached a higher breakpoint (t(14) = 4.69, p<0.001) (e), obtained more rewards (t(14) = 2.84, p=0.01) (f), and performed more correct nosepokes (t(14) = 2.66, p=0.02) (g), indicating that animals with high levels of GSH in nucleus accumbens performed better in the PR paradigm. Unpaired t test two-tailed (***p<0.0001, * p<0.05, N=7–9/group). See Figure 3—figure supplement 1 and Figure 3—source data 1. EPM: elevated plus maze; OF: open field; NO: novel object.
-
Figure 3—source data 1
Raw data Figure 3.
- https://cdn.elifesciences.org/articles/77791/elife-77791-fig3-data1-v1.xlsx
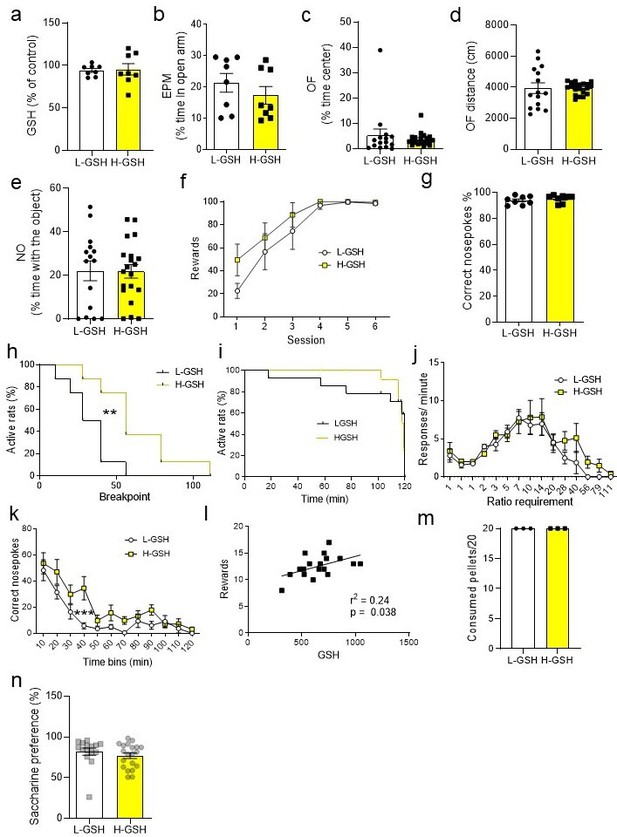
High accumbal glutathione (GSH) levels affect the probability to get a higher breakpoint and the persistence of nosepoking over time.
(a) High GSH (H-GSH) and low GSH (L-GSH) rats showed no significant differences in medial prefrontal cortex (mPFC) GSH levels measured by high-performance liquid chromatography (unpaired two-tailed t-test, t(14) = 0.18, p=0.86; Bayesian independent samples t-test for the null hypothesis [H0: there is no increase in mPFC GSH levels in H-GSH group] yields a BF01=2.61). (b) These groups neither showed significant differences in the percentage of time spent in the open arms of the elevated plus maze (EPM; t(14) = 0.97, p=0.35; Bayesian independent samples t-test for the null hypothesis [H0: there is no increase in mPFC GSH in H-GSH group] yields a BF01=1.71). No significant difference was observed between the two groups in the time the rats spent in the center of the open field (OF; t(33) = 0.71, p=0.48; Bayesian independent samples t-test for the null hypothesis [H0: there is no difference between the two groups in the time the rats spent in the center of OF] yields a BF01=2.51) (c), in the distance traveled in the OF (t(33) = 0.014, p=0.99; Bayesian independent samples t-test for the null hypothesis [H0: there is no difference between the two groups in the distance traveled in the OF] yields a BF01=3.05, moderate evidence), (d) or the percentage of time spent exploring a novel object (NO) (e) (t(33) = 0.047, p=0.96; Bayesian independent samples t-test for the null hypothesis [H0: there is no difference between the two groups in the time spent exploring a novel object] yields a BF01=3.05, moderate evidence). (f) There were no significant differences between H-GSH and L-GSH rats in the training performance in the operant conditioning. Two-way repeated measures ANOVA revealed a non-significant time × GSH interaction (F(5,65) = 0.80, p=0.56), a significant effect of time (F(5,65) = 20.04, p<0.0001), and a non-significant effect of GSH (F(1,13) = 1.62, p=0.23). (g) The percentage of correct nosepokes over total nosepokes in the progressive ratio (PR) test was not different between the groups (t(14) = 0.88, p=0.39; Bayesian independent samples t-test for the null hypothesis [H0: there is no difference between the two groups in the percentage of correct nosepokes over total nosepokes in the PR] yields a BF01=1.81). (h) Survival analysis showed that the H-GSH group had higher probability to reach higher breakpoints than the L-GSH group (Long-rank Mantel-Cox test, Chi2=6.88, p<0.01). (i) Survival analysis showed that L-GSH and H-GSH rats did not show significant differences in how long they kept performing nosepokes (Long-rank Mantel-Cox test, Chi2=0.0, p=0.99). (j) There were no significant differences between groups considering the response/minute by ratio requirements. Two-way repeated measures ANOVA showed a non-significant GSH × ratio requirements (F(14,168)=0.74, p=0.74), a significant effect of ratio requirement (F(14,168)=13.87, p<0.001), and a non-significant effect of GSH (F(1,12) = 0.90, p=0.36). (k) When considering the number of correct nosepokes over time, two-way repeated measures ANOVA revealed a non-significant GSH × time interaction (F(11, 154)=1.53, p=0.12) a significant effect of time (F(11,154) = 21.85, p<0.001), and a significant effect of GSH levels (F(1,14) = 6.81, p=0.02). (l) Correlation between GSH levels in nucleus accumbens and rewards obtained during the PR task (Pearson r=0.49, r2=0.24, p=0.038). (m) L- and H-GSH rats did not exhibit significant differences in the consumption of pellets when they were given free access to them. (n) No significant differences were observed between groups in the saccharine preference test (unpaired two-tailed t-test, t(33) = 0.98, p=0.33; Bayesian independent samples t-test for the null hypothesis [H0: there is no difference between L- and H-GSH in the saccharine preference test] yields a BF01=2.11).
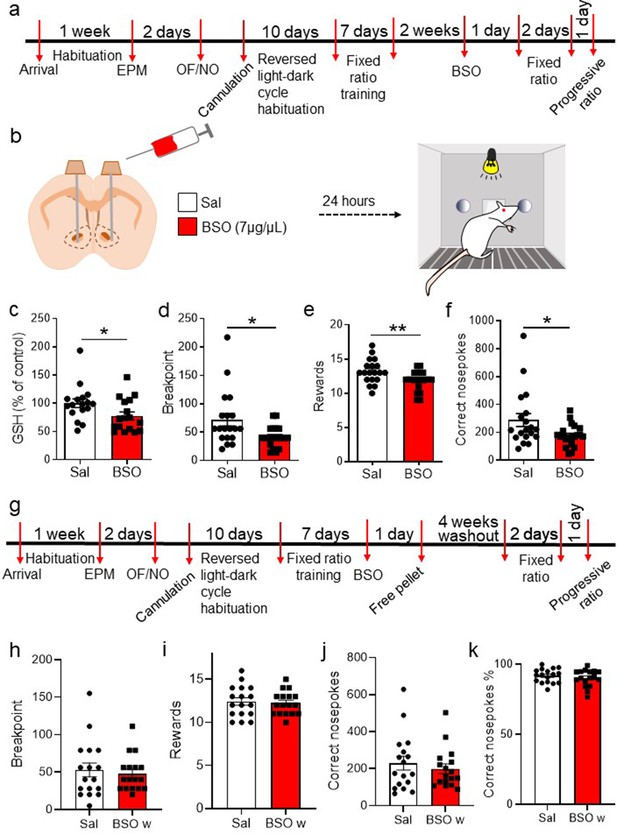
Intra-accumbal administration of buthionine sulfoximine (BSO) impairs progressive ratio (PR) performance.
(a) Cannulated rats were habituated for 10 days in reversed light-dark cycle during their recovery from surgery. Subsequently, they were trained in a fixed ratio 1 schedule for a total of six sessions. (b) 24 h before the PR test, BSO or saline (Sal) was infused via a cannula into the nucleus accumbens. (c) Glutathione (GSH) levels were measured in the nucleus accumbens of Sal- and BSO-infused rats by high-performance liquid chromatography, demonstrating the ability of BSO to reduce GSH levels (unpaired two-tailed t-test t(32) = 2.15, p=0.039 N=17/group). (d) PR performance was impaired in BSO-infused animals compared to the vehicle-treated group, as indicated by the lower breakpoint (unpaired two-tailed t-test t(38) = 2.64, p=0.012). (e) The number of obtained rewards was also lower following intra-accumbal BSO treatment (unpaired two-tailed t-test t(38) = 2.72, p=0.01). (f) BSO-treated rats performed fewer correct nosepokes compared to vehicle-treated rats (unpaired two-tailed t-test t(38) = 2.38, p=0.023, N=19–21/group). (g) Timeline showing the experimental design for the washout experiment. Rats treated with vehicle or Sal were tested in the PR task 4 weeks after the BSO infusion. (h) The breakpoint was not decreased in BSO-infused rats (unpaired two-tailed t-test, t(32) = 0.47, p=0.64; Bayesian independent samples t-test for the null hypothesis [H0: there is no decrease in the breakpoint in the BSO-infused rats] yields a BF01=4.08, moderate evidence). (i) The number of obtained rewards was not significantly decreased in the BSO-infused groups (unpaired two-tailed t-test, t(32) = 0.323, p=0.75; Bayesian independent samples t-test for the null hypothesis [H0: there is no decrease in the obtained rewards in the BSO-infused rats] yields a BF01=3.75, moderate evidence). (j) The number of correct nosepokes was not significantly different between groups (unpaired two-tailed t-test, t(32) = 0.68, p=0.50; Bayesian independent samples t-test for the null hypothesis [H0: there is no decrease in the number of correct nosepokes performed by BSO-infused rats] yields a BF01=4.59, moderate evidence). (k) Rats previously treated with BSO showed the same percentage of correct nosepokes, over the total number of nosepokes in the washout session (unpaired two-tailed t-test, t(32) = 0.87, p=0.39, N=17 per group; Bayesian independent samples t-test for the null hypothesis [H0: there is no decrease in the percentage of correct nosepokes in the BSO-infused rats] yields a BF01=5.04, moderate evidence). OF: open field; NO: novel object. See Figure 4—figure supplement 1 and Figure 4—source data 1.
-
Figure 4—source data 1
Raw data Figure 4.
- https://cdn.elifesciences.org/articles/77791/elife-77791-fig4-data1-v1.xlsx
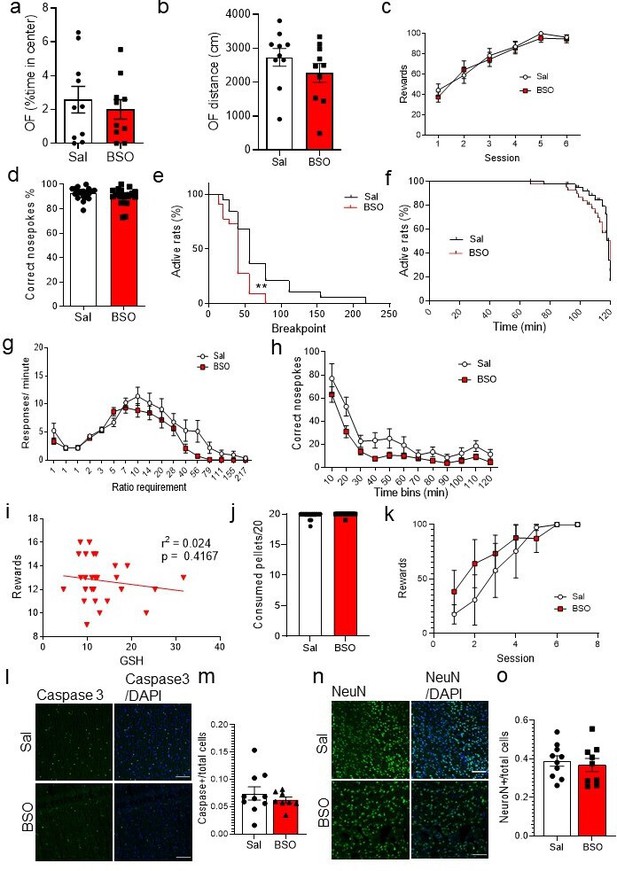
Accumbal buthionine sulfoximine (BSO) infusion decreases the probability of reaching higher breakpoints in progressive ratio (PR) task.
(a) No significant differences were observed in the percentage of time spent in the center of the open field (OF; unpaired two-tailed t-test, t(18) = 0.60, p=0.56; Bayesian independent samples t-test for the null hypothesis [H0: there is no difference in the percentage of time spent in the center of the OF between groups] yields a BF01=2.22) or (b) the distance traveled in the OF between groups (unpaired two-tailed t-test, t(18) = 1.20, p=0.24; Bayesian independent samples t-test for the null hypothesis [H0: there is no difference in the distance traveled in the OF between saline [Sal] and BSO-infused rats] yields a BF01=1.51). (c) The Sal and BSO groups did not show differences for their operant training performance in the fixed ratio 1 (FR1) training. Repeated measures two-way ANOVA showed a non-significant group × time interaction (F(5, 230)=0.41, p=0.84), a significant effect of time (F(5, 230)=45.27, p<0.0001), and a non-significant effect of treatment (F(1, 46)=0.14, p=0.71). (d) Regarding the percentage of correct nosepokes over the total number of nosepokes performed in the PR test, there were no significant differences between groups (unpaired two-tailed t-test, t(37) = 1.34, p=0.19; Bayesian independent samples t-test for the null hypothesis [H0: there is no difference in the percentage of correct nosepokes over the total number in the PR between groups] yields a BF01=1.59). (e) There was a lower probability of reaching a higher breakpoint for the BSO group than the Sal (Long-rank Mantel-Cox test, Chi2 (1)=7.87, p=0.005). (f) BSO rats did not show a significant decrease in the probability to make correct nosepokes over time, compared to the Sal group (Long-rank Mantel-Cox test, Chi2 (1)=0.27, p=0.61). (g) No significant difference was observed in the rate of responses per ratio requirement between the two groups. Two-way ANOVA revealed a non-significant ratio requirement × treatment interaction (F(16, 608)=1.49, p=0.096), a significant ratio requirement effect (F(16, 608)=32.93, p<0.0001), and a non-significant effect of treatment (F(1,38) = 2.61, p=0.11). (h) There was a non-significant treatment × time interaction (F(11, 418)=0.77, p=0.68), but significant effects of time (F(11, 418)=36.47, p<0.0001) and treatment (F(1, 38)=6.17, p=0.018) when comparing the correct nosepokes over time. (i) No correlation was observed between glutathione (GSH) levels in nucleus accumbens (NuAc) and rewards obtained in PR task after BSO infusion in NuAc (Pearson r=–0.16, r2=0.025, p=0.42; Bayesian correlation for the null hypothesis [H0: there is no correlation between GSH levels in NuAc and rewards obtained in the PR task after BSO infusion in NuAc] yields a BF01=3.16, moderate evidence). (j) Sal- and BSO-infused rats did not show significant differences in the consumption of pellets when they were given free access to them (unpaired two-tailed t-test, t(32) = 1.19, p=0.24; Bayesian independent samples t-test for the null hypothesis [H0: there is no difference in the pellet consumption between groups] yields a BF01=1.77). (k) No significant differences were observed in the rewards obtained during the modified FR1 reward learning training per session after BSO or Sal infusion (two-way repeated measures ANOVA, treatment: F(1,49) = 1.57, p=0.22, time: F(6,49) = 6.61, p<0.0001, Time × treatment interaction: F(6,49) = 0.46, p=0.84). (l–m) Confocal images and the quantification showing no significant differences in Caspase 3 activation following BSO treatment in the NuAc (unpaired two-tailed t-test, t(16) = 0.83, p=0.42; Bayesian independent samples t-test for the null hypothesis [H0: there is no difference in Caspase 3 activation between groups] yields a BF01=1.91). Scale bar: 100 µm. (n and o) NeuN staining revealed no significant difference in the number of NeuN+ cells in the NuAc, following BSO treatment (unpaired two-tailed t-test, t(17) = 0.47, p=0.65; Bayesian independent samples t-test for the null hypothesis [H0: there is no difference in the number of NeuN+ cells between groups] yields a BF01=2.29). N=8–10/group.
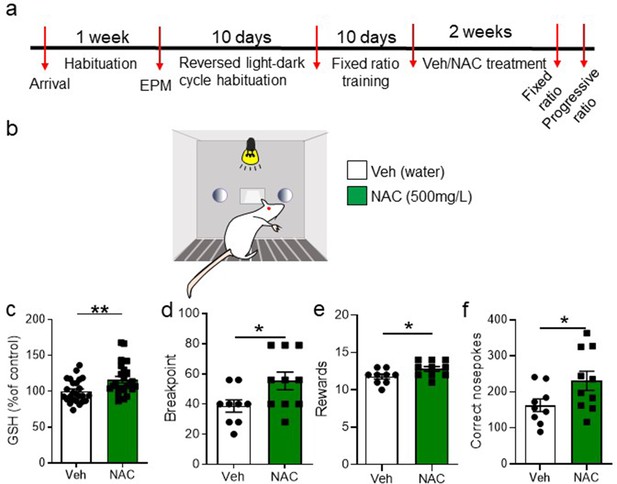
N-acetyl-cysteine (NAC) systemic treatment increases glutathione (GSH) levels in nucleus accumbens and ameliorates progressive ratio (PR) task accomplishment.
(a) Timeline of the experiment. After initial habituation, handling, and behavioral characterization for anxiety, rats were placed in reverse light-dark cycle and trained in an FR1 schedule. Once matched for performance and weight, rats were distributed into vehicle and NAC groups and received normal drinking water or 500 mg/L of NAC in the drinking water, for 2 weeks. Then, both groups were tested in the PR test (b). (c) A separate cohort of vehicle- or NAC-treated animals was euthanized, and GSH levels were measured in the nucleus accumbens (unpaired two-tailed t-test, t(47) = 2.97, p=0.005, N=24–25/group). (d) NAC-treated rats reached a higher breakpoint than vehicle-treated rats (unpaired two-tailed t-test, t(17) = 2.27, p=0.036, N=8/group). (e) Similarly, NAC-treated rats obtained more rewards than vehicle-treated rats (unpaired two-tailed t-test, t(17) = 2.21, p=0.041, N=8/group). (f) NAC-treated rats performed more correct nosepokes than vehicle-treated rats (unpaired two-tailed t-test, t(15.24)=2.09, p=0.052) (**p<0.01, *p≤0.05, N=9–10/group). See Figure 5—figure supplement 1 and Figure 5—source data 1.
-
Figure 5—source data 1
Raw data Figure 5.
- https://cdn.elifesciences.org/articles/77791/elife-77791-fig5-data1-v1.xlsx
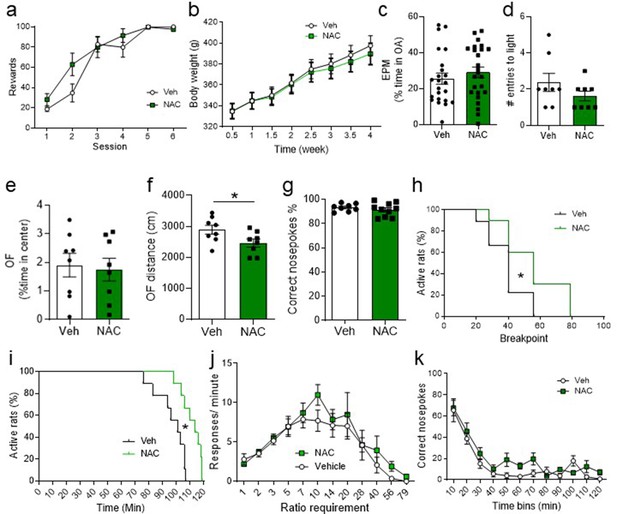
N-acetyl-cysteine (NAC) treatment affects the probability of obtaining a higher breakpoint and persistence of nosepoking over the time but does not change body weight or exploration levels.
(a) During operant conditioning training, no significant differences were observed between vehicle (Veh)- and NAC-treated groups (two-way repeated measures ANOVA, treatment: F(1,19) = 1.27, p=0.27, time: F(5,95) = 59.32, p<0.001, Time × treatment interaction: F(5,95) = 2.26, p=0.054). (b) The two groups did not differ in body weight over time in animals neither at baseline or after treatment (two-way repeated measures ANOVA, treatment: F(1,20) = 0.057, p=0.82, time: F(7,140) = 449.9, p<0.001, Time × treatment interaction: F(7, 140) = 2.29, p=0.031), nor in the percent time spent in elevated plus maze (EPM) the open arm before the treatment (unpaired two-tailed t-test, t(46) = 0.82, p=0.42; Bayesian independent samples t-test for the null hypothesis [H0: there is no difference in the percent time spent in the open arm of EPM before the treatment between groups] yields a BF01=2.65) (c). (d) No significant differences were observed in the light-dark box-anxiety test between Veh- and NAC-treated rats (unpaired two-tailed t-test, t(14) = 1.33, p=0.20; Bayesian independent samples t-test for the null hypothesis [H0: there is no difference in the light-dark box test between groups] yields a BF01=1.31). (e) NAC-treated rats from a different cohort showed no significant difference in the percentage of time spent in the center of the open field (OF) compared to the Veh group (unpaired two-tailed t-test, t(14) = 0.28, p=0.78; Bayesian independent samples t-test for the null hypothesis [H0: there is no difference in the percentage of time spent in the center of the OF between groups] yields a BF01=2.27). (f) However, rats that received NAC treatment traveled a shorter distance in the OF test (unpaired two-tailed t-test, t(14) = 2.22, p=0.043). (g) During the progressive ratio task, no significant differences were observed between Veh- and NAC-treated groups on the percentage of correct nosepokes over the total number of nosepokes (unpaired two-tailed t-test, t(16) = 0.82, p=0.42; Bayesian independent samples t-test for the null hypothesis [H0: there is no difference in the percentage of correct nosepokes over the total number of nosepokes between groups] yields a BF01=1.92). (h) Interestingly, the survival plots showed that NAC-treated rats had a higher probability to reach higher breakpoints than Veh-treated rats (Long-rank Mantel-Cox test, Chi2(1)=4.36, p=0.037). (i) NAC-treated rats had a higher probability to continue performing nosepokes for longer (Long-rank Mantel-Cox test, Chi2(1)=9.28, p=0.002). (j) No statistically significant differences were found between groups regarding the number of responses/minute for the different ratio requirements (two-way repeated measures ANOVA, treatment: F(1,17) = 0.92, p=0.35, time: F(11,187) = 15.45, p<0.001, time × treatment interaction: F(11,187) = 0.49, p=0.91), or the correct nosepokes over time (k) (two-way repeated measures ANOVA, treatment: F(1,17) = 3.72, p=0.071, time: F(11,187) = 34.93, p<0.001, Time × treatment interaction: F(11,187) = 1.38, p=0.18).
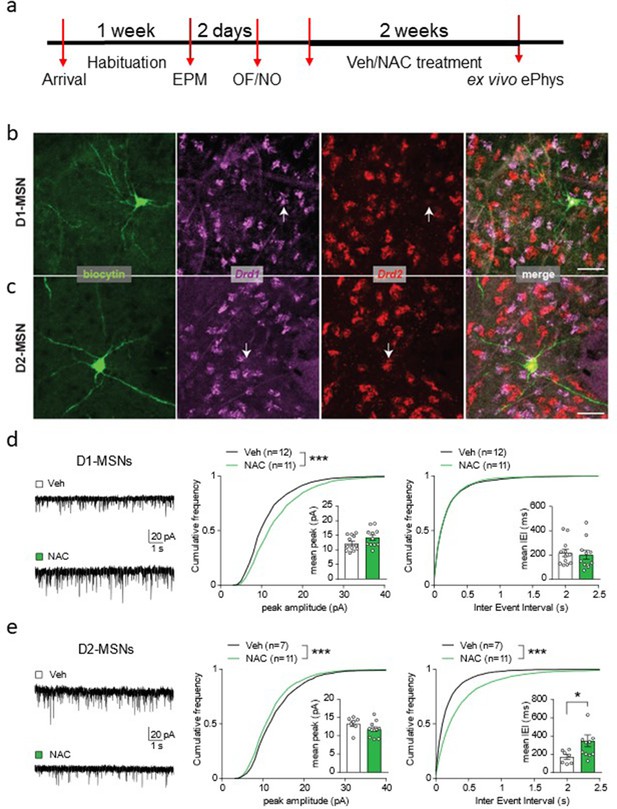
N-acetyl-cysteine (NAC) treatment affects excitatory inputs onto medium spiny neurons (MSNs) in a cell-type-specific manner.
(a) Experimental timeline for ex vivo electrophysiological recordings in NAC-treated rats. (b–c) Confocal micrographs showing neurons in the nucleus accumbens core filled with biocytin during patch-clamp recordings and identified post-hoc as dopamine receptor type 1-MSN (D1-MSN) (b) and dopamine receptor type 2-MSN (D2-MSN) (c) based on RNAscope reactivity for Drd1 and Drd2 mRNA (color-coded). Scale bar, 50 µm. (d) Left, example traces of miniature excitatory postsynaptic currents (mEPSCs) in D1-MSNs from vehicle (Veh)- and NAC-treated rats. Cumulative frequency plots indicate larger peak amplitude in NAC-treated rats (middle panel, Kolmogorov-Smirnov test, D=0.16, ***p<0.001, n=11–12/group), with no change in mEPSC interevent interval (right panel, Kolmogorov-Smirnov test, D=0.018, p=0.84). Insets with bar graphs represent mean values per cell (peak, unpaired t test, t(19.52)=1.69, p=0.11; Bayesian independent samples t-test for the null hypothesis [H0: there is no difference in mean values per cell between groups] yields a BF01=0.96; inter-event interval, Mann-Whitney test, U=56, p=0.57). (e) Left, example traces of mEPSCs in D2-MSNs from Veh- and NAC-treated rats. Cumulative frequency plots indicate smaller peak amplitude (middle panel, Kolmogorov-Smirnov test, D=0.075, ***p<0.001, n=7–11/group) and larger inter-event interval in NAC-treated rats (right panel, Kolmogorov-Smirnov test, D=0.22, ***p<0.001). Insets with bar graphs represent mean values per cell (peak, unpaired t test, t(13.79)=1.59, p=0.14; Bayesian independent samples t-test for the null hypothesis [H0: there is no difference in mean values per cell between groups] yields a BF01=1.08; inter-event interval, Mann-Whitney test, U=16, *p=0.04). See Figure 6—figure supplement 1 and Figure 6—source data 1.
-
Figure 6—source data 1
Raw data Figure 6.
- https://cdn.elifesciences.org/articles/77791/elife-77791-fig6-data1-v1.xlsx
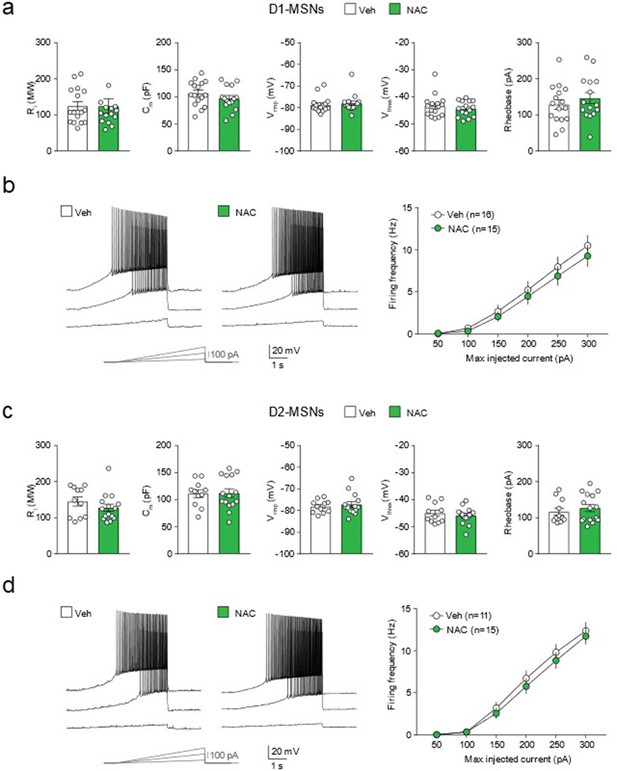
N-acetyl-cysteine (NAC) treatment does not affect medium spiny neuron (MSN) intrinsic excitability.
(a) Lack of significant differences in cell passive and active properties of nucleus accumbens (NuAc) core dopamine receptor type 1-MSNs (D1-MSNs) from vehicle (Veh)- and NAC-treated rats: input resistance (Ri) (Mann-Whitney test, U=110, p=0.71); membrane capacitance (Cm) (unpaired t test, t(28.96)=1.17, p=0.25; Bayesian independent samples t-test for the null hypothesis [H0: there is no difference in Cm per cell between groups] yields a (BF01=1.76) resting membrane potential (Vrmp) (Mann-Whitney test, U=92, p=0.28); firing threshold (Vthres) (Mann-Whitney test, U=111.5, p=0.75); rheobase (unpaired t test, t(27.86)=0.87, p=0.39; Bayesian independent samples t-test for the null hypothesis [H0: there is no difference in rheobase values per cell between groups] yields a BF01=2.20 n=15–16/group). (b) Left, example voltage responses elicited in D1-MSNs from Veh- and NAC-treated rats by injection of current ramps (protocol at the bottom; three of six ramps displayed). Right, frequency-current relationship of action potential discharges evoked by current ramps, indicating no treatment-induced differences (two-way ANOVA for factor treatment, F(1, 174)=1.70, p=0.19, n=15–16/group). (c) Lack of significant differences in cell passive and active properties of NuAc core dopamine receptor type 2-MSNs (D2-MSNs) from Veh- and NAC-treated rats: Ri (Mann-Whitney test, U=61, p=0.27, n=11–15/group), Cm (unpaired t test, t(23.92)=0.11, p=0.92; Bayesian independent samples t-test for the null hypothesis [H0: there is no difference in Cm per cell between groups]) yields a BF01=2.72) n=11–15/group; Vrmp (Mann-Whitney test, U=78, p=0.84, n=11–15/group); Vthres (unpaired t test, t(19.29)=0.64, p=0.53; Bayesian independent samples t-test for the null hypothesis [H0: there is no difference in Vthres per cell between groups] yields a BF01=2.33n=11–15/group); rheobase (Mann-Whitney test, U=73, p=0.64, n=11–15/group). (d) Same representation as in (b) for D2-MSNs (two-way ANOVA for factor treatment, F(1, 144)=1.48, p=0.23, n=11–15/group).
Tables
Reagent type (species) or resource | Designation | Source or reference | Identifiers | Additional information |
---|---|---|---|---|
Strain, strain background (Wistar, male) | Rats | Charles River, L’Arbresle, France | RRID:RGD_2312504 | |
Antibody | Anti-NeuN (rabbit polyclonal) | Millipore | Cat. #:ABN78 RRID:AB_10807945 | 1:1000 |
Antibody | Anti-cleaved caspase-3 (rabbit polyclonal) | Cell Signaling Technology | Cat. #:9661 RRID:AB_2341188 | 1:200 |
Antibody | Anti-mouse Alexa Fluor 488 (goat polyclonal) | Thermo Fisher Scientific | Cat. #:A-11029 RRID:AB_2534088 | 1:1000 |
Antibody | Anti-rabbit Alexa Fluor 488 (donkey polyclonal) | Thermo Fisher Scientific | Cat. #:A-21206 RRID:AB_2535792 | 1:1000 |
Peptide, recombinant protein | Streptavidin, Alexa Fluor 488 conjugate | Thermo Fisher Scientific | Cat. #:S‐11223 | 1:500 |
Commercial assay or kit | RNAscope Fluorescent Multiplex Reagent Kit | ACD, Bio-Techne | Cat. #:320850 | probes: Rn-Drd1a-C2 no.317031, Rn-Drd2-C1 no.315641 |
Chemical compound, drug | Biocytin hydrochloride | Sigma-Aldrich | Cat. #:B1758 CAS No:98930-70-2 | |
Chemical compound, drug | Tetrodotoxin | Latoxan | Cat. #:L8503 CAS No:4368-28-9 | |
Chemical compound, drug | Picrotoxin | abcam | Cat. #:ab120315 CAS No:124-87-8 | |
Chemical compound, drug | N-acetyl-cysteine (NAC) | Sigma-Aldrich | Cat. #:A9165 CAS No:616-91-1 | |
Chemical compound, drug | L-Buthionine-sulfoximine (BSO) | Sigma-Aldrich | Cat. #:B2515 CAS No:83730-53-4 | |
Chemical compound, drug | L-Glutathione (GSH) | Sigma-Aldrich | Cat. #:G4251 CAS No:70-18-8 | |
Chemical compound, drug | 4′,6-Diamidino-2-phenyl-indol -dihydrochlorid | Sigma-Aldrich | Cat. #:D9542 CAS No: 28718-90-3 | |
Software, algorithm | GraphPad Prism 9 | GraphPad Software | RRID:SCR_002798 | |
Software, algorithm | pClamp 10 | Molecular Devices, LLC | RRID:SCR_011323 | |
Software, algorithm | Mini Analysis Program 6.0.3 | Synaptosoft Inc | RRID:SCR_002184 | |
Software, algorithm | QuPath | PMID:29203879 | RRID:SCR_018257 | |
Other | 1H-MRS Magnetom 7T/68 cm head | Siemens, Erlangen, Germany | Human spectroscopy | |
Other | 1H-MRS horizontal 9.4T/31 cm | Magnex Scientific, Abingdon, UK | Rodent spectroscopy |