Self-assembly of pericentriolar material in interphase cells lacking centrioles
Figures
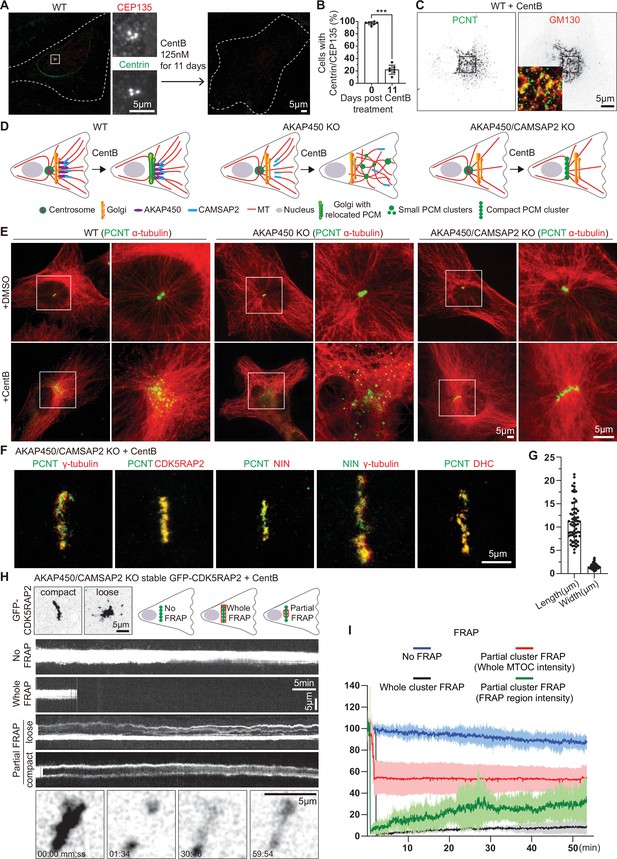
Formation and characterization of caMTOCs in AKAP450/CAMSAP2 knockout cells.
(A) Immunofluorescence images of control or centrinone-treated wild type (WT) RPE1 cells stained for centrioles (CEP135, red; centrin, green). The zooms of the boxed area show the centrioles stained with the indicated markers. (B) Quantification shows the percentage of cells with centrioles before and after the centrinone treatment. 350 cells (n=7 fields of view) analyzed for each measurement in three independent experiments. The statistical significance was determined by unpaired two-tailed Mann-Whitney test in Prism 9.1 (***p<0.001). Values represent mean ± SD. (C) Immunofluorescence images of centrinone-treated WT RPE1 cells stained for pericentrin (PCNT, green) and the Golgi marker GM130 (red). Inset shows the merged image of the boxed area. (D) Diagrams of the microtubule organization in WT and knockout (KO) cells used. (E) Immunofluorescence images of control and centrinone-treated WT and knockout RPE1 cell lines stained for pericentrin (green) and microtubules (α-tubulin, red). Enlargements on the right show the boxed areas. (F) Immunofluorescence images of centrinone-treated AKAP450/CAMSAP2 knockout RPE1 cells stained for different PCM components as indicated and imaged by STED microscopy. (G) Quantification of the length and width of cylindrical PCM clusters. n=65 cells analyzed in three independent experiments. Values represent mean ± SD. (H) (Top left) Two frames of time-lapse images of centrinone-treated AKAP450/CAMSAP2 knockout RPE1 cells stably expressing GFP-CDK5RAP2 prior to FRAP experiments. (Top right) Schemes show regions of caMTOC where photobleaching was performed. (Middle) Kymographs illustrating fluorescence of unbleached caMTOC (No FRAP), fully photobleached caMTOC (Whole FRAP) and partially photobleached caMTOC (Partial FRAP). (Bottom) Time-lapse images illustrating partial FRAP of a caMTOC. Time is min: s. (I) Normalized fluorescence intensity as a function of time. The blue line shows averaged intensity traces of unbleached caMTOCs (No FRAP), the black line shows averaged intensity traces of fully photobleached caMTOCs (Whole FRAP), the red line shows averaged intensity traces of whole caMTOC that were partially photobleached (whole caMTOC intensity, Partial FRAP) and the green line shows averaged intensity traces of the photobleached region of the partially photobleached caMTOC (FRAP region intensity, Partial FRAP). n=3 for No FRAP, 3 for Whole FRAP, 5 for Partial FRAP (whole caMTOC intensity) and 5 for Partial FRAP (FRAP region intensity); time-lapse images of ~1600 timepoints with 2 s interval were analyzed for each measurement. Values are mean ± SD.
-
Figure 1—source data 1
An Excel sheet with numerical data on the quantifications shown in panels B, G, and I.
- https://cdn.elifesciences.org/articles/77892/elife-77892-fig1-data1-v2.xlsx
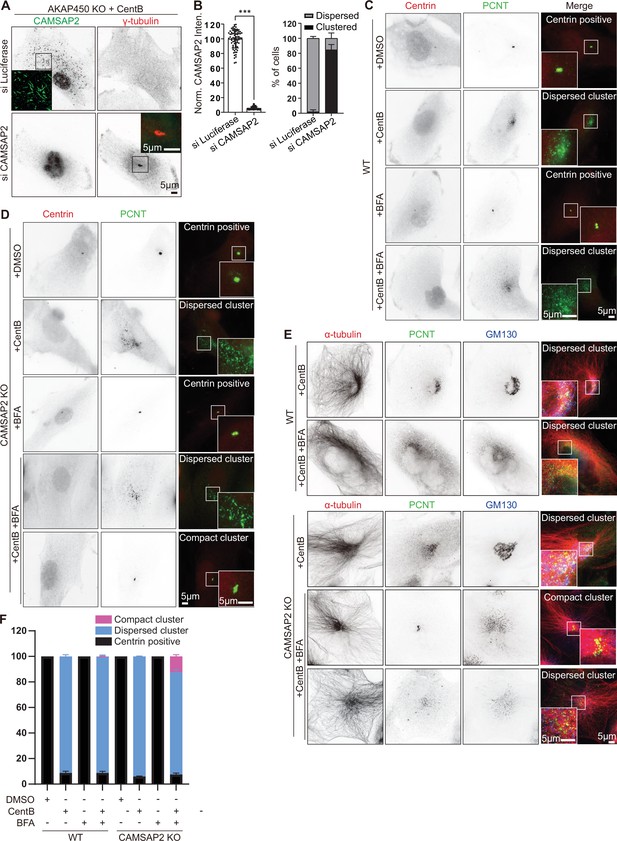
Characterization of caMTOCs in wild type, AKAP450 knockout and CAMSAP2 knockout cells.
(A) Immunofluorescence images of centrinone B treated AKAP450 knockout RPE1 cells stained for CAMSAP2 (green) and γ-tubulin (red), transfected either with control (Luciferase) or CAMSAP2 siRNAs. Insets show enlargements of the merged images of the boxed areas. (B) Quantification of CAMSAP2 depletion and caMTOC formation related to panel A. For normalized CAMSAP2 intensity, n=76 (siLuciferase) and 62 (siCAMSAP2) cells from three independent experiment were analyzed; to calculate the percentage of cells showing dispersed or clustered PCM, n=300 (siLuciferase) and 299 (siCAMSAP2) cells analyzed for each measurement in three independent experiments. The statistical significance was determined by unpaired two-tailed Mann-Whitney test in Prism 9.1 (***p<0.001). Values represent mean ± SD. (C–E) Immunofluorescence images of wild type and CAMSAP2 knockout cells treated with DMSO, CentB, Brefeldin A (BFA) (5 µg/ml (17.8 µM) for 2 hrs), and stained for centrioles (Centrin, red), PCM (PCNT, green), microtubules (α-tubulin, red), and Golgi apparatus (GM130, blue). Insets show enlargements of the merged channels of the boxed areas. (F) Quantification of the proportion of cells with different types of microtubule minus end organization in wild type (WT) and CAMSAP2 knockout RPE1 cell lines with indicated treatment. 319 (+DMSO), 413(+CentB), 412(+BFA), 353(+CentB + BFA) WT cells and 340(+DMSO), 445(+CentB), 384(+BFA), 553(+CentB + BFA) CAMSAP2 knockout RPE1 cells analyzed for each measurement in three independent experiments (n=3). Values represent mean ± SD.
-
Figure 1—figure supplement 1—source data 1
An Excel sheet with numerical data on the quantifications shown in panels B and F.
- https://cdn.elifesciences.org/articles/77892/elife-77892-fig1-figsupp1-data1-v2.xlsx
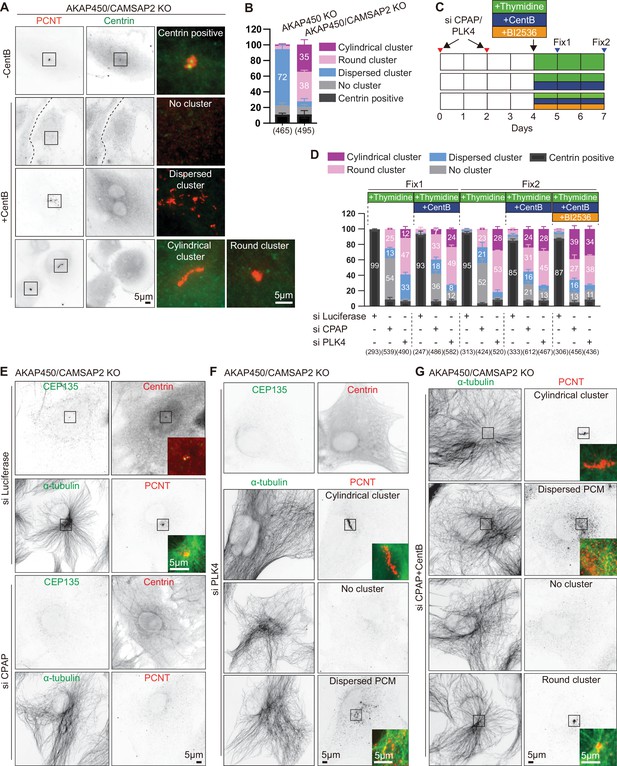
Characterization of caMTOCs in AKAP450/CAMSAP2 knockout cells.
(A) Immunofluorescence images of control (-CentB) and centrinone-treated AKAP450/CAMSAP2 knockout RPE1 cells stained for pericentrin and centrin to show the main PCM organization types (centrin-negative cells with cylindrical or round PCM cluster, dispersed PCM or no cluster, and centrin-positive centrosomes) in each condition. Enlargements of the merged channels of the boxed areas are shown on the right. (B) Quantification of the main PCM organization types in centrinone-treated AKAP450 knockout and AKAP450/CAMSAP2 knockout RPE1 cells. Numbers on the histogram show the percentages. 465 (AKAP450 KO) and 495 (AKAP450/CAMSAP2 KO) cells analyzed for each measurement in three independent experiments (n=3). Values represent mean ± SD. (C) Diagram showing the generation of CPAP or PLK4 depleted AKAP450/CAMSAP2 knockout RPE1 cells with different drug treatments. Cells were transfected with siRNAs to depleted CPAP and PLK4 respectively. After 2 days, the transfection was performed again to increase the depletion efficiency. After 2 more days, cells were treated with thymidine to block cell proliferation or with a combination of thymidine and centrinone B, or thymidine, centrinone B and the PLK1 inhibitor BI 2536 for one or three days. Cells were fixed for the first time (Fix1) after a 24 hr drug treatment, and for the second time (Fix2) after a 72 hr drug treatment. (D) Quantification of the main PCM organization types, as described in panel A, for cells prepared as described in panel E-G. Numbers on the histogram show the percentages and numbers in brackets show cells analyzed for each measurement in three independent experiments (n=3; 247–612 cells analyzed per condition). Values represent mean ± SD. (E–G) Immunofluorescence images of Fix2 (as described in panel C) showing control (transfected with siRNA against Luciferase), or depleted of PLK4 or CPAP and treated as indicated. Cells were stained for centrioles (CEP135, centrin), PCM proteins (PCNT) and microtubules (α-tubulin). Images illustrate the PCM organization types in each condition. Insets show the merged channels of the boxed areas.
-
Figure 1—figure supplement 2—source data 1
An Excel sheet with numerical data on the quantifications shown in panels B and D.
- https://cdn.elifesciences.org/articles/77892/elife-77892-fig1-figsupp2-data1-v2.xlsx
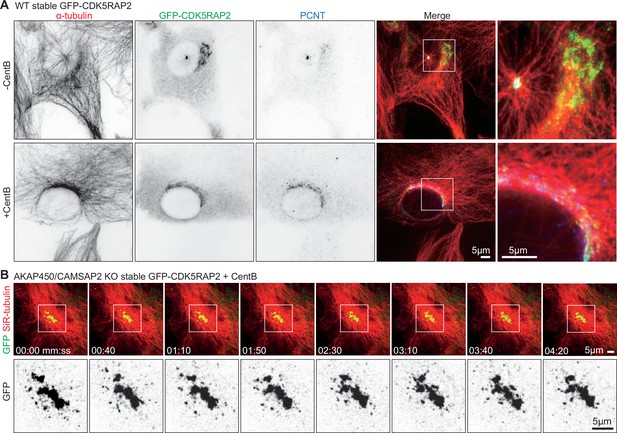
PCM dynamics visualized with GFP-CDK5RAP2.
(A) Immunofluorescence images of control (-CentB) and centrinone B treated WT RPE1 cell stably expressing GFP-CDK5RAP2 (green) stained for α-tubulin (red) and pericentrin (PCNT, blue). Zooms show enlargements of the boxed regions. (B) Time-lapse images of centrinone-treated AKAP450/CAMSAP2 knockout RPE1 cells stably expressing GFP-CDK5RAP2. Microtubules are visualized by treating the cells with 100 nM SiR-tubulin overnight. PCM labeled with GFP-CDK5RAP2 (green) forms a stable cluster that functions as the MTOC. Zooms show the enlarged GFP channel of the boxed regions. Time is min: s.

Molecular composition of caMTOCs in AKAP450/CAMSAP2 knockout cells.
All the cells used in this figure are AKAP450/CAMSAP2 knockout cells, except for panel E as indicated. (A–C) Immunofluorescence images of control or centrinone-treated AKAP450/CAMSAP2 knockout RPE1 cells stained for and depleted of the indicated proteins. (D, F–I) Immunofluorescence images of centrinone-treated AKAP450/CAMSAP2 knockout RPE1 cells stained for and depleted of the indicated proteins. (E) Immunofluorescence images of centrinone-treated AKAP450/CAMSAP2/p53 knockout and AKAP450/CAMSAP2/p53/pericentrin knockout RPE1 cells stained as indicated. In panels A-I, insets show enlargements of the merged channels of the boxed areas, and dashed lines indicate cell edges. (J) Quantification of the main PCM organization types, as described in Figure 1—figure supplement 2A, for cells prepared as described in panel A-C, E-I. Numbers on the histogram show the percentages. 1293 (-CentB), 1547(+CentB), 2021(siLuci), 1822(siCEP152), 1345(siCEP192), 1161(siNEDD1), 2302 (AKAP450/CAMSAP2/p53/PCNT knockout (PCNT KO)), 2264(siCDK5RAP2), 2510(siγ-tubulin), 2408(siNIN) and 2526(siDHC) cells were analyzed for each measurement in three independent experiments (n=3). Values represent mean ± SD. (K) Summarizing table of PCM localization and the depletion effects on caMTOC formation in AKAP450/CAMSAP2 knockout RPE1 cells. NT, not tested.
-
Figure 2—source data 1
An Excel sheet with numerical data on the quantifications shown in panel J.
- https://cdn.elifesciences.org/articles/77892/elife-77892-fig2-data1-v2.xlsx
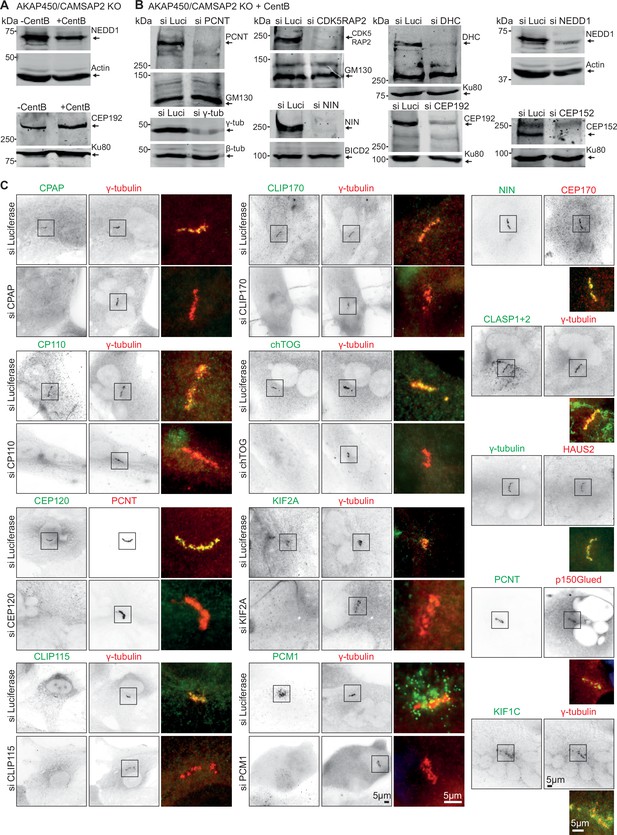
Characterization of PCM components localizing to caMTOCs in AKAP450/CAMSAP2 knockout cells.
(A) Western blot showing that endogenous NEDD1 and CEP192 are present in centrinone-treated AKAP450/CAMSAP2 knockout cells. (B) Western blot results showing the depletion of the indicated PCM proteins. (C) Immunofluorescence images of centrinone-treated AKAP450/CAMSAP2 knockout RPE1 cells showing the localization and the effects of depletion of the indicated proteins on the caMTOCs. siRNA against luciferase was used as a control. Zooms show enlargements of the merged channels of the boxed areas.
-
Figure 2—figure supplement 1—source data 1
Full raw unedited western blots shown in panels A and B.
- https://cdn.elifesciences.org/articles/77892/elife-77892-fig2-figsupp1-data1-v2.zip
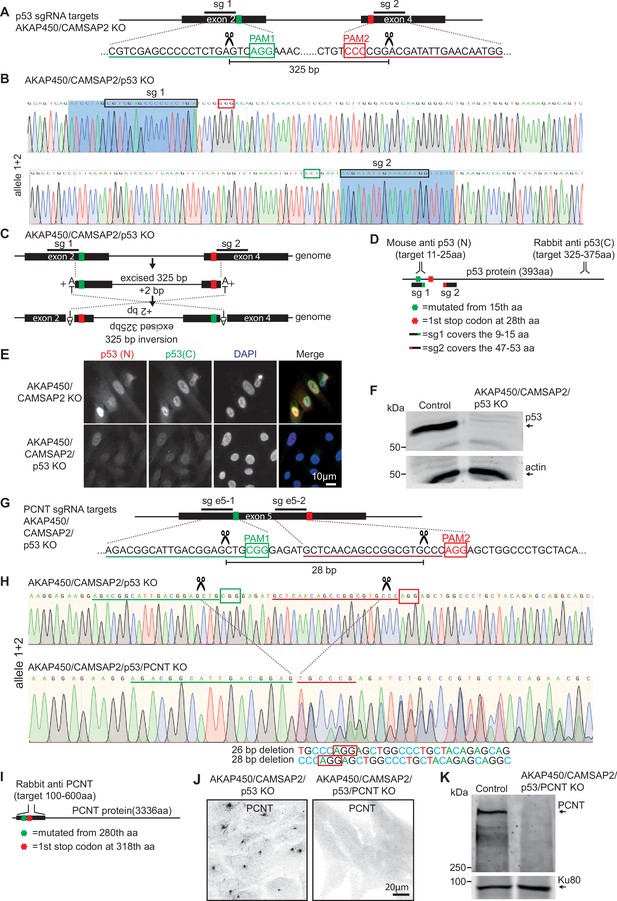
Generation of the AKAP450/CAMSAP2/p53/pericentrin knockout RPE1 cell line.
(A) Diagram illustrating two sgRNA sites targeting p53 exon 2 and exon 4. The green and red boxes indicate the position of the PAM sites, and the predicted Cas9 cut sites are indicated by scissors. (B,C) Genotyping results of the genomic mutation using gel-purified PCR product which covers exon 2 and exon 4 of the p53-encoding gene in AKAP450/CAMSAP2/p53 knockout cell line and a diagram illustrating the induced inversion. (D) Diagram illustrating the binding sites of p53 antibodies and the position of the mutation induced in p53-encoding gene. (E,F) Immunofluorescence and Western blot images showing p53 expression in control and AKAP450/CAMSAP2/p53 knockout cells. (G–H) Genotyping results of the genomic mutation using gel-purified PCR product which covers the two sgRNAs targeting sites within pericentrin-encoding gene in AKAP450/CAMSAP2/p53/pericentrin knockout cell line and a diagram illustrating the induced genomic mutations within exon 5 of the pericentrin-encoding gene. The green and red boxes indicate the position of the PAM sites, and the predicted Cas9 cut sites are indicated by scissors. (I) Diagram illustrating the binding domain of the pericentrin antibody and the positions of the mutations induced downstream of the Cas9 cutting sites. (J) Immunofluorescence images confirming the loss of pericentrin in AKAP450/CAMSAP2/p53/pericentrin knockout cells. (K) Western blot confirming the loss of p53 and pericentrin in AKAP450/CAMSAP2/p53/pericentrin knockout cells.
-
Figure 2—figure supplement 2—source data 1
Full raw unedited western blots shown in panels F and K.
- https://cdn.elifesciences.org/articles/77892/elife-77892-fig2-figsupp2-data1-v2.zip
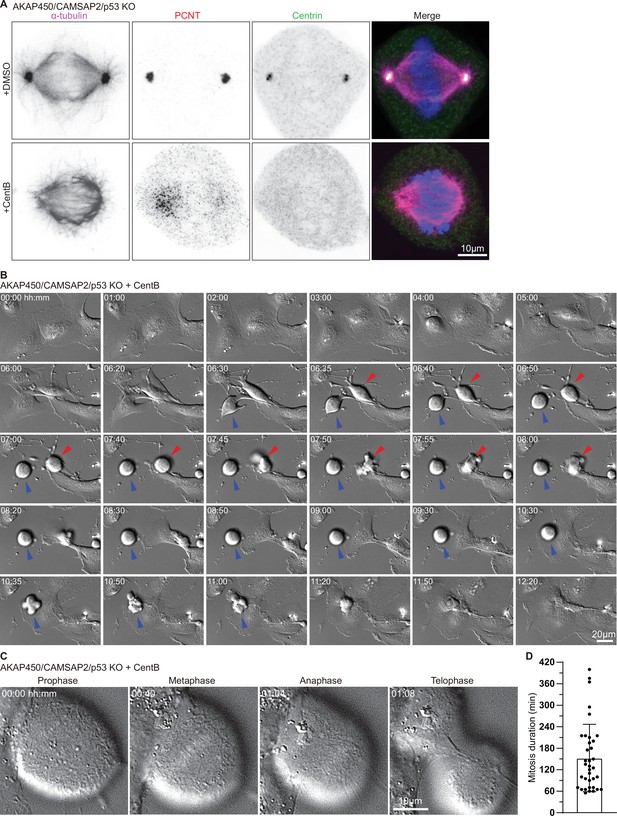
Characterization of cell division in centrinone-treated AKAP450/CAMSAP2/p53 knockout cells.
(A) Immunofluorescence images of metaphase AKAP450/CAMSAP2/p53 knockout cells treated with DMSO and centrinone (CentB) and stained for h microtubules (α-tubulin, magenta), PCM (PCNT, red), centrioles (Centrin, green) and DNA (DAPI, blue). (B–C) Phase-contrast time-lapse images of centrinone-treated AKAP450/CAMSAP2/p53 knockout RPE1 cells. Time is hh:mm. (B) The red and blue arrows show two cells with different mitosis duration. (C) Mitotic phases in a representative cell. (D) Quantification of the mitosis duration of centrinone-treated AKAP450/CAMSAP2/p53 knockout RPE1 cells. Thirty-six cells from 14 videos were analyzed. Values represent mean ± SD.
-
Figure 2—figure supplement 3—source data 1
An Excel sheet with numerical data on the quantifications shown in panel D.
- https://cdn.elifesciences.org/articles/77892/elife-77892-fig2-figsupp3-data1-v2.xlsx
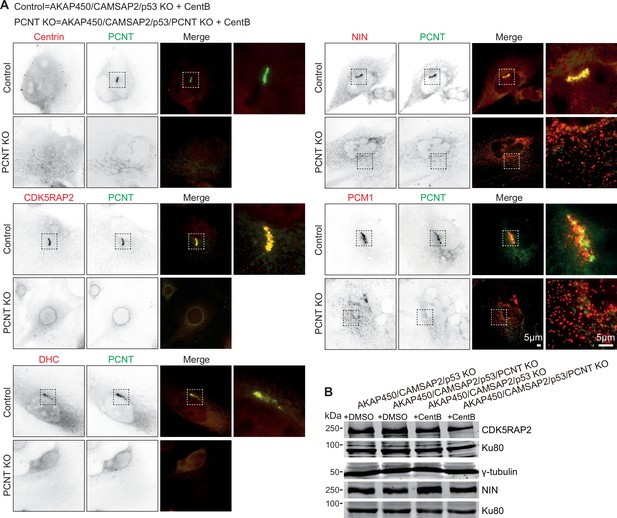
Characterization of PCM organization in AKAP450/CAMSAP2/p53/pericentrin knockout cells.
(A) Immunofluorescence images of centrinone-treated AKAP450/CAMSAP2/p53 knockout (Control) and AKAP450/CAMSAP2/p53/pericentrin knockout (PCNT KO) RPE1 cells showing staining for the indicated proteins. Zooms of the boxed areas show merged channels. (B) Western blots showing the expression levels of CDK5RAP2, γ-tub, and ninein in control (+DMSO) and centrinone-treated (+CentB) AKAP450/CAMSAP2/p53 knockout and AKAP450/CAMSAP2/p53/PCNT knockout RPE1 cells.
-
Figure 2—figure supplement 4—source data 1
Full raw unedited western blots shown in panel B.
- https://cdn.elifesciences.org/articles/77892/elife-77892-fig2-figsupp4-data1-v2.zip
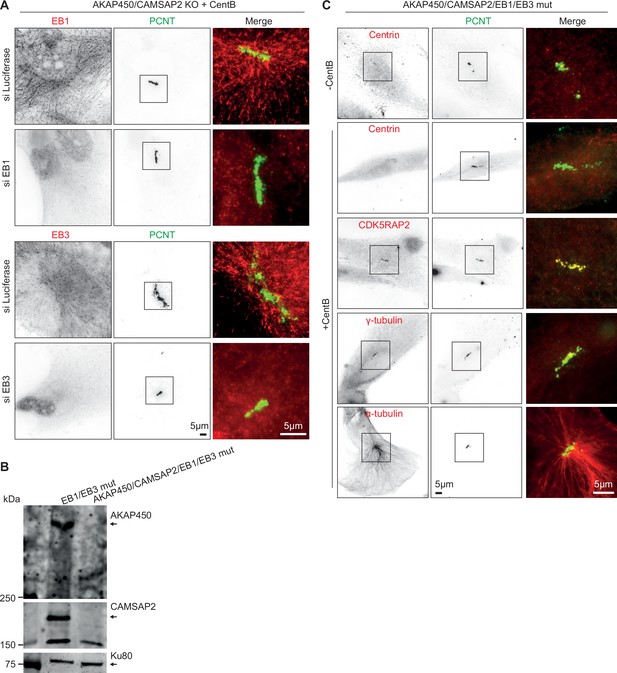
Effects of the depletion or knockout of EB1 and EB3 on caMTOC formation in AKAP450/CAMSAP2 knockout cells.
(A) Immunofluorescence images of centrinone-treated AKAP450/CAMSAP2 knockout cells stained for EB1 or EB3 and pericentrin showing effects of EB1 or EB3 depletion on caMTOCs. (B) Western blot confirming the loss of AKAP450 and CAMSAP2 in AKAP450/CAMSAP2/EB1/EB3 mutant cells. (C) Immunofluorescence images of control or centrinone-treated AKAP450/CASMSAP2/EB1/EB3 mutant RPE1 cells stained for the indicated proteins. Zooms of the boxed areas show merged channels.
-
Figure 2—figure supplement 5—source data 1
Full raw unedited western blots shown in panel B.
- https://cdn.elifesciences.org/articles/77892/elife-77892-fig2-figsupp5-data1-v2.zip

Microtubule organization in acentriolar cells missing different caMTOC components.
(A) Immunofluorescence images of centrinone-treated AKAP450/CAMSAP2 knockout RPE1 cells depleted of the indicated proteins and stained for microtubules (α-tubulin, red) and different PCM proteins (green). Insets show enlargements of the merged channels of the boxed areas and dashed lines show cell boundaries. (B) Quantification of the normalized overall microtubule intensity for the indicated conditions. The number of cells analyzed in three independent experiments: n=56 (siLuci), 45 (siPCNT), 33 (siCDK5RAP2), 36 (siNinein), 43 (siγ-tubulin), and 28 (siDHC). The statistical significance was determined by unpaired two-tailed Mann-Whitney test in Prism 9.1 (***P<0.001). Values represent mean ± SD. (C) Microtubule images were split into a radial and non-radial components (heat maps) based on microtubule orientation in relation to the PCM clusters or the brightest point, as described in Materials and methods. (D) Quantification of the proportion of the non-radial microtubules shown in panel C (see Materials and methods for details). The number of cells analyzed for each measurement in three independent experiments: n=25 (siLuci), 43 (siPCNT), 32 (siCDK5RAP2), 34 (siNinein), 37 (siγ-tubulin), and 25 (siDHC). The statistical significance was determined by unpaired two-tailed Mann-Whitney test in Prism 9.1 (***p<0.001). Values represent mean ± SD. (E) Diagram illustrating the distribution of PCM clusters and microtubule organization upon the depletion of the indicated proteins in centrinone-treated AKAP450/CAMSAP2 knockout cells.
-
Figure 3—source data 1
An Excel sheet with numerical data on the quantifications shown in panel B and D.
- https://cdn.elifesciences.org/articles/77892/elife-77892-fig3-data1-v2.xlsx

Microtubule- and dynein-dependent disassembly of caMTOCs.
(A) Diagram illustrating different order of cell treatments with nocodazole (Noco) and/or dynapyrazole A (Dyna, 5 µM) and the time points when the cells were fixed. (B) Immunofluorescence staining of centrinone-treated AKAP450/CAMSAP2 knockout cells treated as shown in panel A. Dashed red circles represent the areas occupied by PCM clusters in each condition. (C) Quantification of the area occupied by PCM clusters in each condition, as shown in panels A and B. n=35–53 cells analyzed for each measurement in three independent experiments. The statistical significance was determined by unpaired two-tailed Mann-Whitney test in Prism 9.1 (not significant (NS), p<0.12; *p<0.033; ***p<0.001). Values are represented as mean ± SD. (D) Western blot showing that 3 hr treatment with dynapyrazole A does not affect the expression of the endogenous dynein heavy chain and the dynactin large subunit p150Glued. (E) Time-lapse images of centrinone-treated AKAP450/CAMSAP2 knockout RPE1 cells stably expressing GFP-CDK5RAP2. Microtubules were visualized by treating cells with 100 nM SiR-tubulin overnight. Red arrows show the immobilized PCM clusters at indicated timepoints. Time is min: s. Time-lapse images of the same cell prior to the nocodazole treatment were shown in Figure 1—figure supplement 3B. (F) (Top) Kymograph illustrating the motility of PCM clusters during microtubule disassembly with nocodazole. (Bottom) Measurements of the normalized microtubule (SiR-tubulin) fluorescence intensity (red plot, left Y-axis) and the instantaneous velocity of GFP-CDK5RAP2 clusters (green plot, right Y-axis) during the movement of GFP-CDK5RAP2 clusters away from caMTOC. Microtubule density around each PCM cluster was determined by measuring mean fluorescence intensity of SiR-tubulin in a circular area with a 2 μm radius centered on the PCM cluster and normalizing it to the mean fluorescence intensity of 20 images prior to nocodazole addition (set as 100%). The moment when a PCM cluster started to move out of the caMTOC was set as the initial time point (0 min) for this cluster, and the subsequent PCM cluster motion velocity and the relative local microtubule density of 43 time points were calculated and averaged. n=12 clusters were analyzed in each condition. Values are represented as mean ± SD. (G) Motor-PAINT images of centrinone-treated AKAP450/CAMSAP2 knockout RPE1 cells before and after nocodazole treatment. Plus-end-out microtubules are shown in white whereas minus-end-out microtubules are shown in magenta. Asterisks represent the putative position of caMTOC. (H) Summarizing diagram illustrating microtubule organization and the motility of GFP-CDK5RAP2-positive PCM clusters during nocodazole treatment and dynapyrazole A (treat first) and nocodazole co-treatment. (I) Immunofluorescence images of centrinone-treated AKAP450/CAMSAP2 knockout RPE1 cells stained for microtubules (α-tubulin, red) and PCM (PCNT, green). Enlargements show the merged and single channels of the boxed areas.
-
Figure 4—source data 1
An Excel sheet with numerical data on the quantifications shown in panels C and F.
- https://cdn.elifesciences.org/articles/77892/elife-77892-fig4-data1-v2.xlsx
-
Figure 4—source data 2
Full raw unedited western blots shown in panel D.
- https://cdn.elifesciences.org/articles/77892/elife-77892-fig4-data2-v2.zip
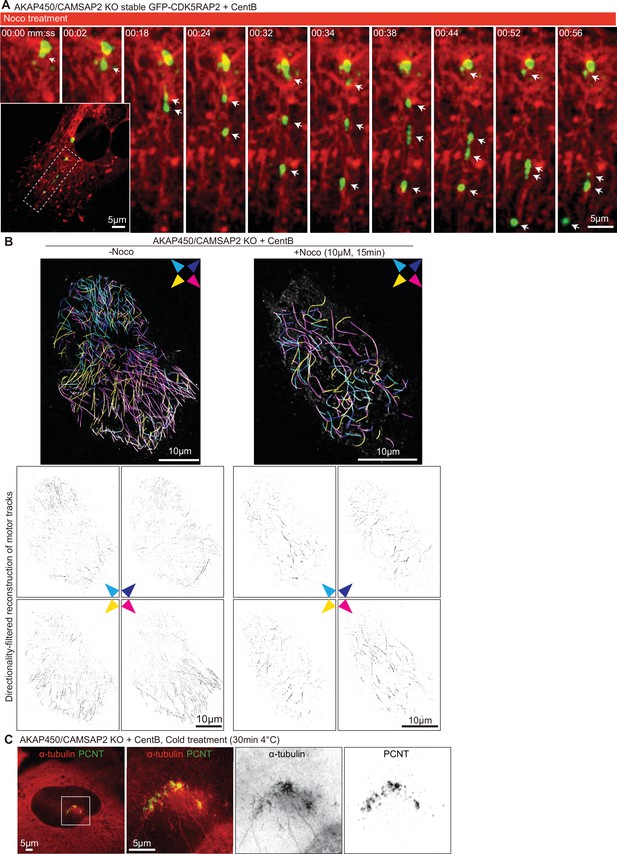
PCM dynamics during nocodazole treatment in centrinone-treated AKAP450/CAMSAP2 knockout cells.
(A) Time-lapse images illustrating the dynamics of GFP-CDK5RAP2 clusters (green, white arrows) and microtubules (visualized with an overnight treatment with 100 nM SiR-tubulin, red) during nocodazole treatment. A single frame of the whole cell is on the bottom left, with the boxed area indicating the location of the enlarged images. Time is min: s. (B) (Top) Motor-PAINT images of centrinone-treated AKAP450/CAMSAP2 knockout RPE1 cells before and after treatment with 10 μM nocodazole for 15 min (the same cells, colorized differently, are displayed in Figure 4G). Microtubule segments were assigned a color based on their absolute orientation, with their plus-ends pointing in the directions indicated with the compass. Asterisks indicate approximate assumed positions of caMTOCs. (Bottom) Directionality-filtered reconstruction of motor tracks used for generating the Motor-PAINT images. (C) Immunofluorescence images of centrinone-treated AKAP450/CAMSAP2 knockout RPE1 cells stained for microtubules (α-tubulin, red) and PCM (PCNT, green) after cold treatment for 30 min at 4 °C. Enlargements show the merged and single channels of the boxed areas.
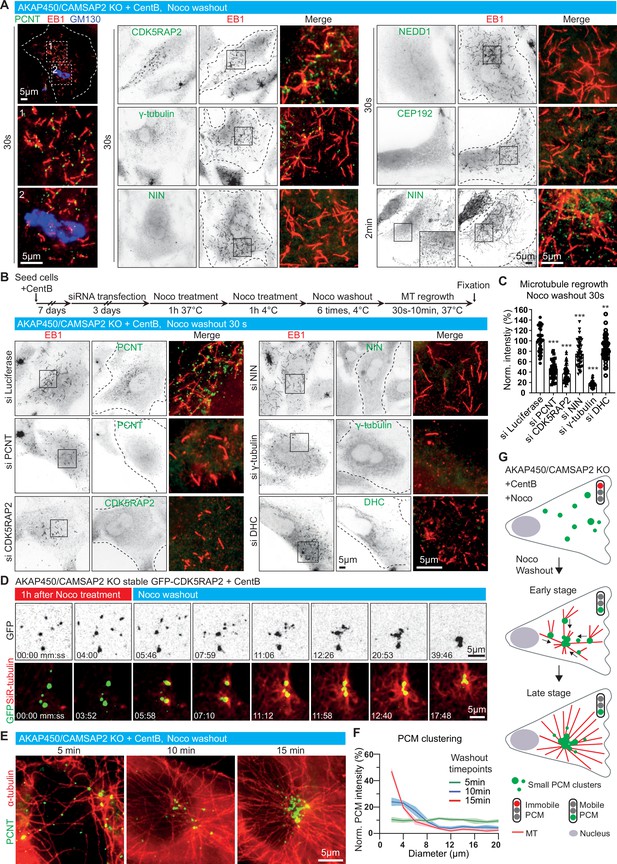
Dynamics of microtubule nucleation and caMTOC re-assembly in acentriolar cells.
(A) Immunofluorescence images of microtubule regrowth after nocodazole washout at the indicated timepoints in centrinone-treated AKAP450/CAMSAP2 knockout RPE1 cells stained for PCM components (green) and newly nucleated microtubules (EB1, red). A Golgi marker, GM130 (blue), is included in the left row, and zooms of the boxed regions (numbered 1 and 2) show that microtubules nucleate from PCM clusters but not from the Golgi membranes. Dashed lines show cell boundaries. (B) (Top) Timeline shows the time course of protein depletion (siRNA transfection), nocodazole treatment, nocodazole washout and microtubule regrowth. (Bottom) Immunofluorescence images of microtubule regrowth experiments after depletion of the indicated proteins in centrinone-treated AKAP450/CAMSAP2 knockout RPE1 cells stained for the indicated PCM markers (green) and EB1 as a marker of nascent microtubules (red). Cell outlines are indicated with dashed lines and enlargements of the merged channels of the boxed areas are shown on the right. (C) Quantification of normalized microtubule intensity at 30 s after nocodazole washout in control cells and cells depleted of the indicated PCM proteins. n=40 (siLuci, siPCNT), 57 (siCDK5RAP2), 48 (siNIN), 45 (siγ-tubulin), and 50 (siDHC) cells analyzed for each measurement in three independent experiments. The statistical significance was determined by unpaired two-tailed Mann-Whitney test in Prism 9.1 (**p<0.002; ***p<0.001). Values are represented as mean ± SD. (D) Time-lapse images of centrinone-treated AKAP450/CAMSAP2 knockout RPE1 cells stably expressing GFP-CDK5RAP2 before and after nocodazole washout. Dispersed GFP-CDK5RAP2-positive PCM clusters (GFP, green) serve as microtubule nucleation sites (SiR-tubulin, red) and coalesce into a big cluster after nocodazole washout. Time is min: s. (E) Immunofluorescence images of centrinone-treated AKAP450/CAMSAP2 knockout RPE1 cells stained for pericentrin (green) and microtubules (α-tubulin, red) at the indicated timepoints after nocodazole washout. (F) Measurements of normalized fluorescence intensity of PCM clusters at the indicated distances in relation to the brightest point, as described in Materials and methods. The biggest PCM cluster (which normally also had the highest fluorescence intensity) was selected as the center, around which 10 concentric circles with 2 μm width were drawn. Fluorescence intensity of PCM clusters in these concentric circles was measured automatically and normalized by the sum of the total PCM intensity in each cell per condition. n=12 cells per plot per timepoint. Values represent mean ± SD. (G) Summarizing diagram illustrating microtubule organization and motility of GFP-CDK5RAP2-positive PCM clusters during nocodazole washout.
-
Figure 5—source data 1
An Excel sheet with numerical data on the quantifications shown in panels C and F.
- https://cdn.elifesciences.org/articles/77892/elife-77892-fig5-data1-v2.xlsx
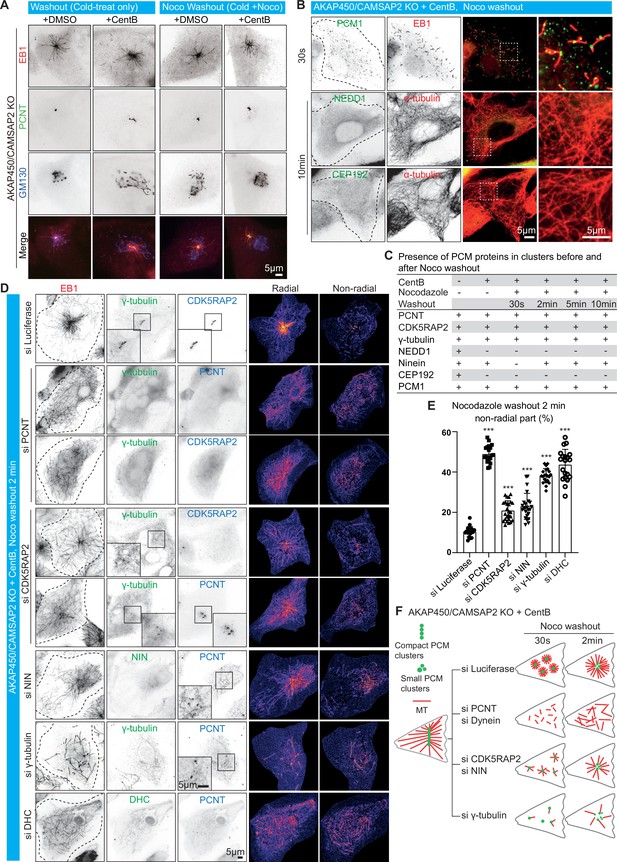
PCM dynamics and microtubule regrowth during nocodazole washout in AKAP450/CAMSAP2 knockout cells.
(A) Immunofluorescence images of control (+DMSO) and centrinone-treated AKAP450/CAMSAP2 knockout cells treated with cold alone or cold and nocodazole, and stained for nascent microtubules (EB1, red), PCM (PCNT, green) and the Golgi apparatus (GM130, blue) 30 s after nocodazole washout. (B) Immunofluorescence images of acentriolar AKAP450/CAMSAP2 knockout RPE1 cells fixed at 30 s and 10 min after nocodazole washout and stained for the indicated markers. Zooms show the enlargements of boxed areas. Dashed lines show the cell boundaries. (C) Summarizing table showing the presence of PCM components in clusters (including centrosome and caMTOC) before and after nocodazole washout in indicated conditions. (D) Immunofluorescence images of control acentriolar AKAP450/CAMSAP2 knockout RPE1 cells and cells lacking the indicated PCM proteins fixed 2 min after nocodazole washout and stained for EB1 (a marker of growing microtubules, red) and different PCM proteins (γ-tubulin, ninein (NIN) and dynein heavy chain (DHC), green; CDK5RAP2 and PCNT, blue). Insets show the enlargements of the boxed areas and the heat maps on the right show the radial and non-radial part of growing microtubules. Dashed lines show the cell boundaries. (E) Quantification of the proportion of non-radial microtubules 2 min after nocodazole washout. The radial and the non-radial microtubule intensities were measured and the non-radial microtubule intensity was normalized by dividing it by the sum of the radial and the non-radial microtubule intensities for each group. n=20–25 cells analyzed for each measurement in three independent experiments. The statistical significance was determined by unpaired two-tailed Mann-Whitney test in Prism 9.1 (***p<0.001). Values represent mean ± SD. (F) Diagram showing microtubule regrowth and PCM assembly in control acentriolar AKAP450/CAMSAP2 knockout RPE1 cells and cells depleted of the indicated PCM proteins at 30 s and 2 min after nocodazole washout.
-
Figure 5—figure supplement 1—source data 1
An Excel sheet with numerical data on the quantifications shown in panel E.
- https://cdn.elifesciences.org/articles/77892/elife-77892-fig5-figsupp1-data1-v2.xlsx
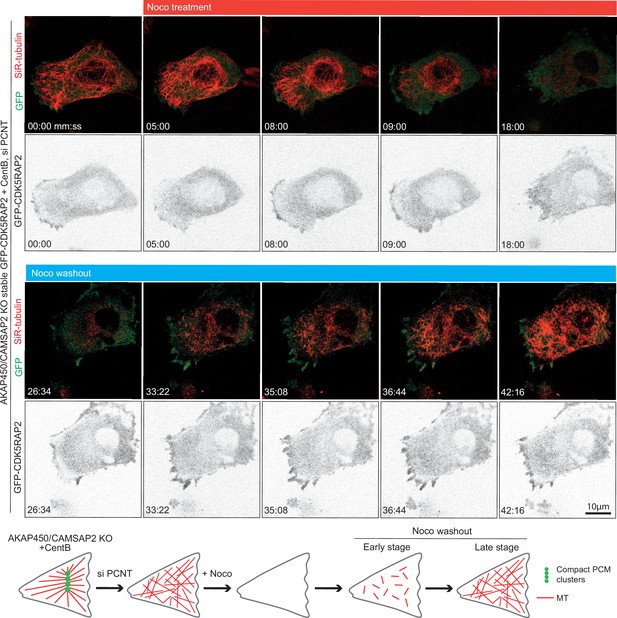
Pericentrin is required for PCM clustering in acentriolar AKAP450/CAMSAP2 knockout RPE1 cells.
Time-lapse images of centrinone-treated AKAP450/CAMSAP2 knockout RPE1 cells stably expressing GFP-CDK5RAP2 (green), which is diffusely distributed and does not form any clear clusters. Microtubule regrowth experiments show random nucleation of microtubules (labeled with 100 nM SiR-tubulin overnight) that remain disorganized. Summarizing diagram shows PCM clusters and microtubule network in the indicated conditions.
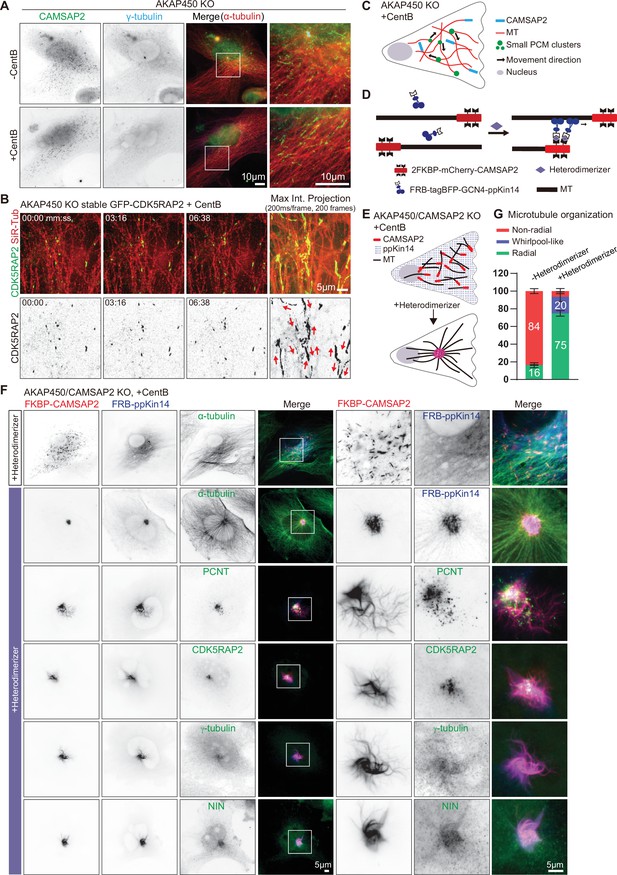
caMTOC formation in the presence of CAMSAP2 using inducible motor recruitment.
(A) Immunofluorescence images of control or centrinone treated AKAP450 knockout RPE1 cells stained for CAMSAP2 (green), PCM protein (γ-tubulin, cyan) and microtubules (α-tubulin, red). Enlargements show the boxed regions of the merged images. (B) Time lapse images of centrinone treated AKAP450 knockout RPE1 cells stably expressing GFP-CDK5RAP2 (green). Microtubules were labeled with 100 nM SiR-tubulin overnight (red, top row). The maximum intensity projection includes 200 frames, 200ms/frame. Red arrows show the motion directions of GFP-CDK5RAP2-positive PCM clusters. Time is min: s. (C) A diagram of microtubule organization and PCM motility in AKAP450 knockout cells. (D, E) Diagram of the inducible heterodimerization assay with ppKin14 and CAMSAP2. (D) CAMSAP2 was tagged with mCherry and fused to a tandemly repeated FKBP domain; tetramerized ppKin14 was tagged with TagBFP and fused to FRB. Heterodimerizer induces the binding of CAMSAP2 and ppKin14 by linking FKBP to FRB. (E) Heterodimerizer treatment induces the binding of CAMSAP2 (red) and ppKin14 (blue) and the formation of radial microtubule network. In this scheme, PCM-anchored microtubules are not shown. (F) Immunofluorescence images of centrinone-treated AKAP450/CAMSAP2 knockout RPE1 cells co-transfected with 2FKBP-mCherry-CAMSAP2 and FRB-TagBFP-GCN4-ppKin14 and stained for the indicated proteins in cells treated with DMSO or heterodimerizer. Zooms show the magnifications of boxed areas. (G) Quantification of the proportion of cells with a radial, whirlpool-like or non-radial microtubule organization with and without heterodimerizer treatment. Numbers on the histogram show the percentages. A total of 414 cells treated with DMSO (-Heterodimerizer) and 385 cells treated with heterodimerizer (+Heterodimerizer) analyzed for each measurement in three independent experiments (n=3). Values represent mean ± SD.
-
Figure 6—source data 1
An Excel sheet with numerical data on the quantifications shown in panel G.
- https://cdn.elifesciences.org/articles/77892/elife-77892-fig6-data1-v2.xlsx
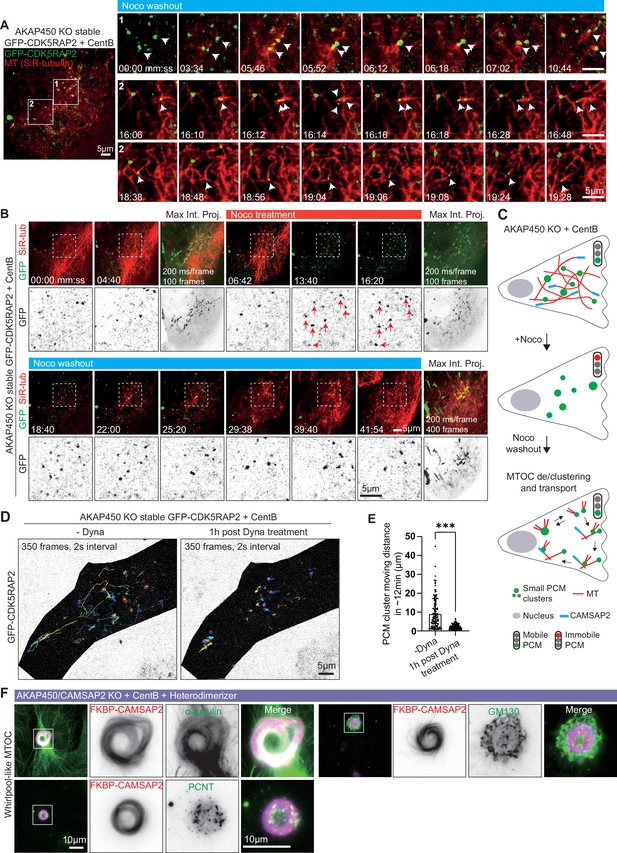
PCM dynamics and the effects of CAMSAP2 clustering on PCM organization in centrinone-treated RPE1 cells lacking AKAP450.
(A) Time-lapse images illustrating the dynamics of PCM clusters (green, white arrows) and microtubules (SiR-tubulin, red) after nocodazole washout in centrinone-treated AKAP450 knockout RPE1 cells stably expressing GFP-CDK5RAP2. A single frame of the whole cell with numbered boxed areas indicating the location of enlarged time-lapse images is shown on the left. White arrows indicate moving PCM clusters after microtubules re-grow. Time is min: s. (B) Time-lapse images of centrinone-treated AKAP450 knockout RPE1 cells stably expressing GFP-CDK5RAP2. GFP-CDK5RAP2 forms small clusters that are dynamic before microtubules (SiR-tubulin, red) are disassembled by nocodazole treatment. Red arrows show that these clusters become immobile when microtubules are completely depolymerized (enlargements of the boxed areas shown in the bottom rows). GFP-CDK5RAP2-positive clusters are sites of microtubule nucleation after nocodazole washout and become dynamic again once microtubules re-grow. The maximum intensity projections include 100 or 400 frames, 200ms/frame. Time is min: s. (C) Summarizing diagram illustrating microtubule organization and the motility of PCM clusters in acentriolar AKAP450 knockout RPE1 cells.(D) Movement trajectories of PCM clusters in acentriolar AKAP450 knockout cells before and after 1 hr treatment with 5 µM dynapyrazole A (Dyna). 350 frames with a 2 s interval from the same cell were analyzed. Shaded areas represent the cell body. (E) Quantification of the length of the trajectories of moving PCM clusters. n=132 (without dynapyrazole A, from 5 cells) and 111 (after 1 hr dynapyrazole A treatment, from 4 cells) trajectories were analyzed for each condition. The statistical significance was determined by unpaired two-tailed Mann-Whitney test in Prism 9.1 (***p<0.001). Values represent mean ± SD. (F) Immunofluorescence images of centrinone-treated AKAP450/CAMSAP2 knockout RPE1 cells transfected with 2FKBP-mCherry-CAMSAP2 and FRB-TagBFP-GCN4-ppKin14 and stained for the indicated proteins after overnight heterodimerizer treatment. Zooms show the magnification of boxed area.
-
Figure 6—figure supplement 1—source data 1
An Excel sheet with numerical data on the quantifications shown in panel E.
- https://cdn.elifesciences.org/articles/77892/elife-77892-fig6-figsupp1-data1-v2.xlsx
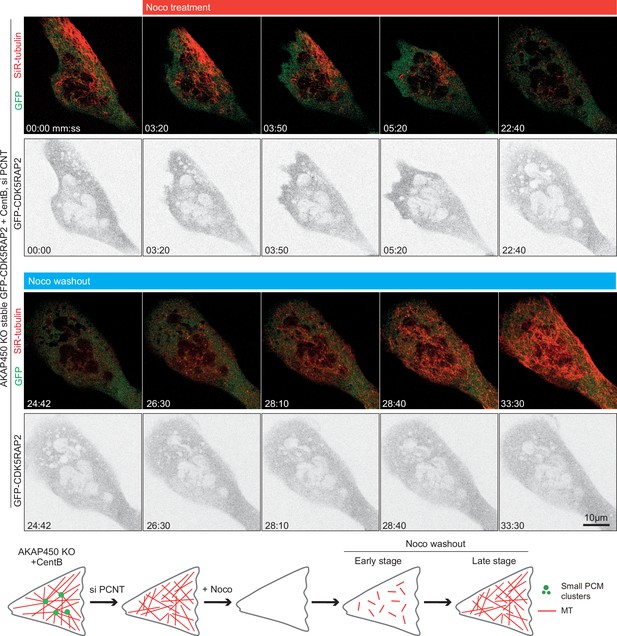
Pericentrin is required for PCM clustering in acentriolar AKAP450 knockout RPE1 cells.
Time-lapse images of centrinone-treated AKAP450 knockout RPE1 cells stably expressing GFP-CDK5RAP2 (green) depleted of pericentrin. GFP-CDK5RAP2 is diffusely distributed and does not form any clear clusters. Microtubule regrowth experiments show random nucleation of microtubules (labeled with 100 nM SiR-tubulin overnight) that remain disorganized. Summarizing diagram shows PCM clusters and microtubule network in the indicated conditions.
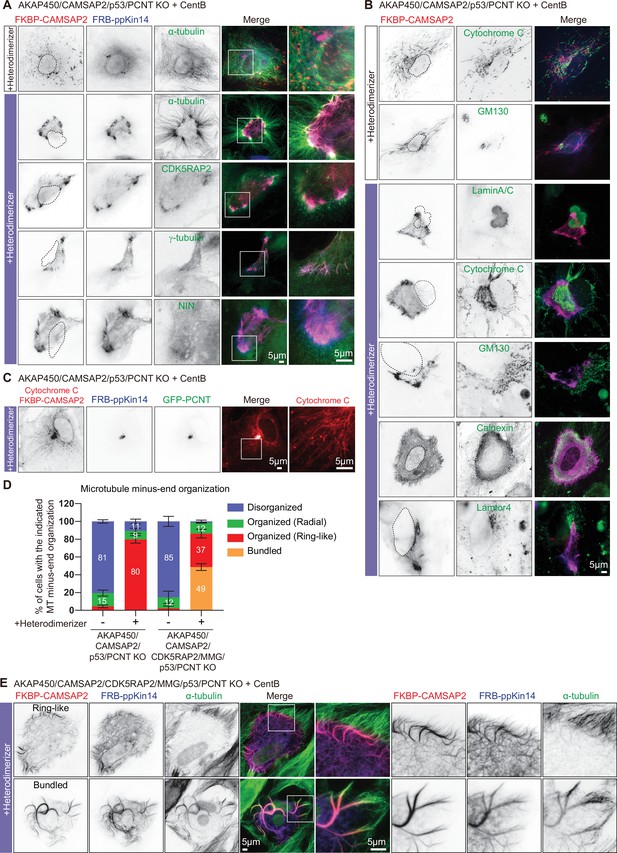
The role of the PCM in CAMSAP2-driven formation of caMTOCs.
(A,B) Immunofluorescence images of centrinone-treated AKAP450/CAMSAP2/p53/pericentrin knockout RPE1 cells transfected with 2FKBP-mCherry-CAMSAP2 and FRB-TagBFP-GCN4-ppKin14 and stained for the indicated components before (top) or after an overnight heterodimerizer treatment. Zooms show magnifications of boxed areas. Black dashed lines show the position of the nucleus. (C) Cells treated as described for panel A were co-transfected with GFP-pericentrin and stained for mitochondria (cytochrome C, red) and CAMSAP2 (red) in same channel overnight after heterodimerizer addition. Zooms show magnifications of boxed areas. (D) Quantification of the proportion of cells with different types of microtubule minus end organization before and after overnight heterodimerizer treatment. Numbers on the histogram show the percentages. 334 (-Heterodimerizer), 424(+Heterodimerizer) cells of AKAP450/CAMSAP2/p53/pericentrin knockout RPE1 cells, 206(-Heterodimerizer), and 239(+Heterodimerizer) of AKAP450/CAMSAP2/CDK5RAP2/MMG/p53/pericentrin knockout RPE1 cells analyzed for each measurement in three independent experiments (n=3). Values represent mean ± SD. (E) Immunofluorescence images of centrinone-treated AKAP450/CAMSAP2/CDK5RAP2/MMG/p53/pericentrin knockout RPE1 cells transfected with 2FKBP-mCherry-CAMSAP2 and FRB-TagBFP-GCN4-ppKin14 and stained for microtubules (α-tubulin, green) after an overnight heterodimerizer treatment. Zooms show magnifications of boxed areas.
-
Figure 7—source data 1
An Excel sheet with numerical data on the quantifications shown in panel D.
- https://cdn.elifesciences.org/articles/77892/elife-77892-fig7-data1-v2.xlsx
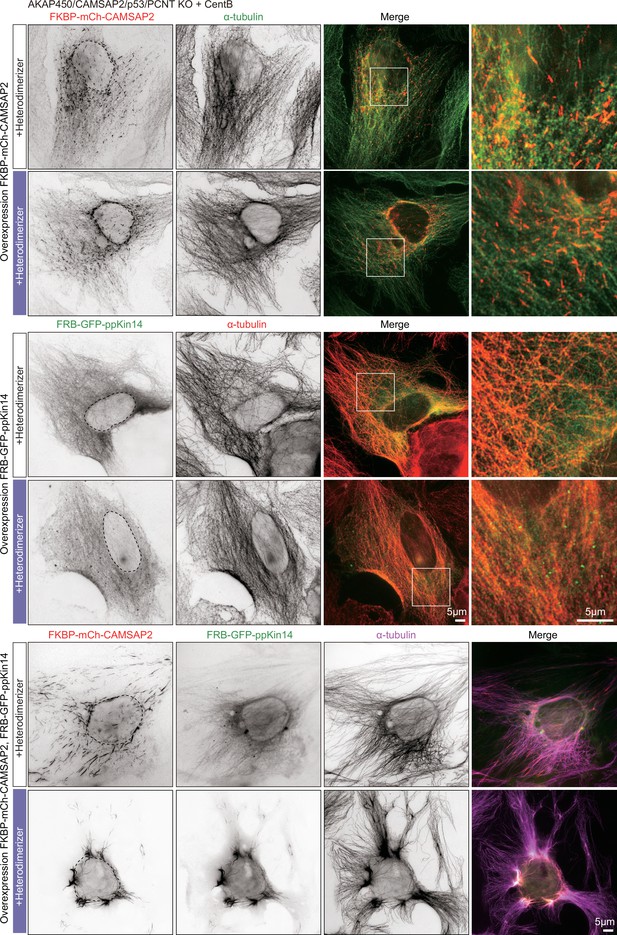
Inducible CAMSAP2-driven microtubule rearrangement in AKAP450/CAMSAP2/p53/pericentrin knockout cell.
Immunofluorescence images of centrinone-treated AKAP450/CAMSAP2/p53/pericentrin knockout cells expressing either FKBP-mCh-CAMSAP2 alone, FRB-GFP-ppKin14 alone or both, with or without heterodimerizer treatment, and stained for microtubules (α-tubulin). Enlargements show the merged channels of the boxed areas.
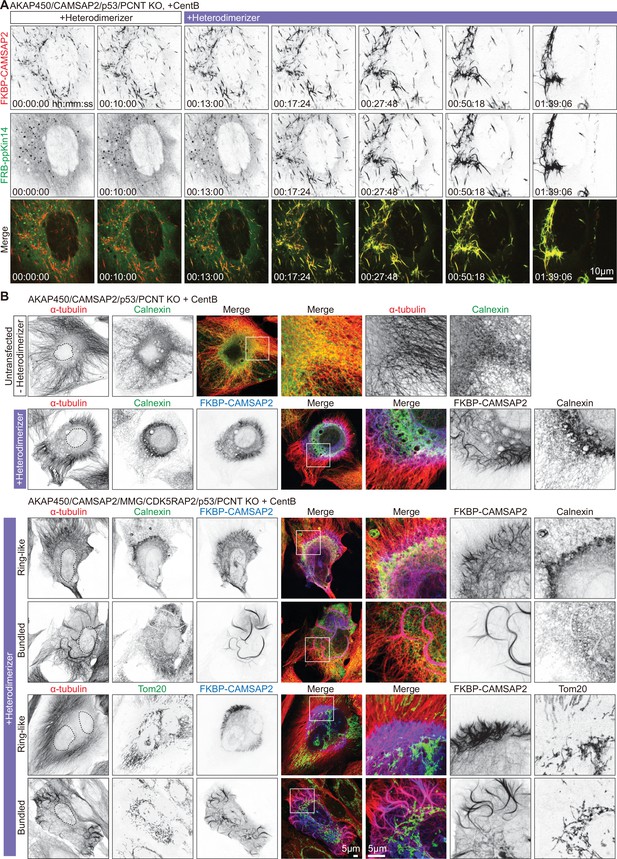
Inducible CAMSAP2-driven radial microtubule rearrangement in AKAP450/CAMSAP2/p53/pericentrin knockout cell.
(A) Time-lapse images of a centrinone-treated AKAP450/CAMSAP2/p53/pericentrin knockout cell transiently expressing 2FKBP-mCherry-CAMSAP2 and FRB-GFP-GCN4-ppKin14 imaged for 10 min (100 frames, 6 s interval) prior to treatment with 100 nM heterodimerizer and ~90 min after. Time is hr: min: s. (B) Immunofluorescence images of centrinone-treated AKAP450/CAMSAP2/p53/pericentrin knockout and AKAP450/CAMSAP2/MMG/CDK5RAP2/p53/pericentrin knockout RPE1 cells, stained for the indicated markers. In the upper row, the cells were neither transfected nor treated with heterodimerizer, whereas in the other panels, cells were transfected with 2FKBP-mCherry-CAMSAP2 and FRB-HA-GCN4-ppKin14 and treated with heterodimerizer overnight. Zooms show the magnification of boxed areas.
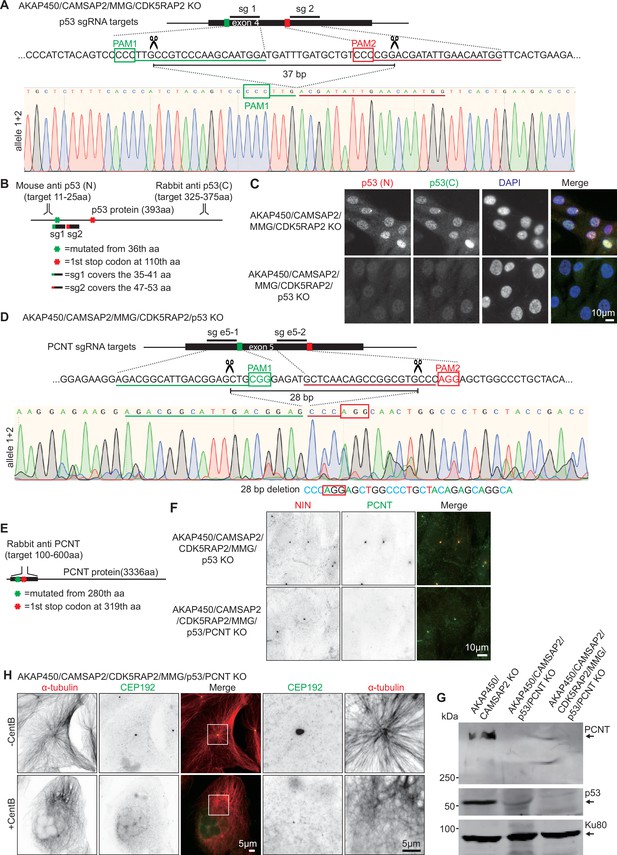
Generation of the AKAP450/CAMSAP2/CDK5RAP2/MMG/ p53/pericentrin knockout RPE1 cell line.
(A) Diagram illustrating two sgRNA sites targeting p53 exon 4. The green and red boxes indicate the position of the PAM sites, and the predicted Cas9 cut sites are indicated by scissors. Genotyping results of the genomic mutation using gel-purified PCR product which covers exon 4 of the p53-encoding gene in AKAP450/CAMSAP2/MMG/CDK5RAP2/p53 knockout cell line are shown below. (B) Diagram illustrating the binding sites of p53 antibodies and the position of the mutation induced in p53-encoding gene. (C) Immunofluorescence images showing p53 staining in control and AKAP450/CAMSAP2/MMG/CDK5RAP2/p53 knockout cells. (D) Diagram illustrating two sgRNA sites targeting pericentrin exon 5. The green and red boxes indicate the position of the PAM sites, and the predicted Cas9 cut sites are indicated by scissors. Genotyping results of the genomic mutation using gel-purified PCR product which covers exon 5 of the pericentrin-encoding gene in AKAP450/CAMSAP2/MMG/CDK5RAP2/p53/pericentrin knockout cell line are shown below. (E) Diagram illustrating the binding domain of the pericentrin antibody and the positions of the mutations induced downstream of the Cas9 cut sites. (F) Immunofluorescence images showing the confirmation of pericentrin loss in control and AKAP450/CAMSAP2/MMG/CDK5RAP2/p53/pericentrin knockout cells. (G) Western blot illustrating the p53 and pericentrin loss in control and AKAP450/CAMSAP2/MMG/CDK5RAP2/p53/pericentrin knockout cells. (H) Immunofluorescence images of control and centrinone-treated AKAP450/CAMSAP2/CDK5RAP2/MMG/p53/pericentrin knockout cells staining microtubules (α-tubulin, red) and centrosomes (CEP192, green). Zooms show magnification of the boxed area.
-
Figure 7—figure supplement 3—source data 1
Full raw unedited western blots shown in panels F and G.
- https://cdn.elifesciences.org/articles/77892/elife-77892-fig7-figsupp3-data1-v2.zip
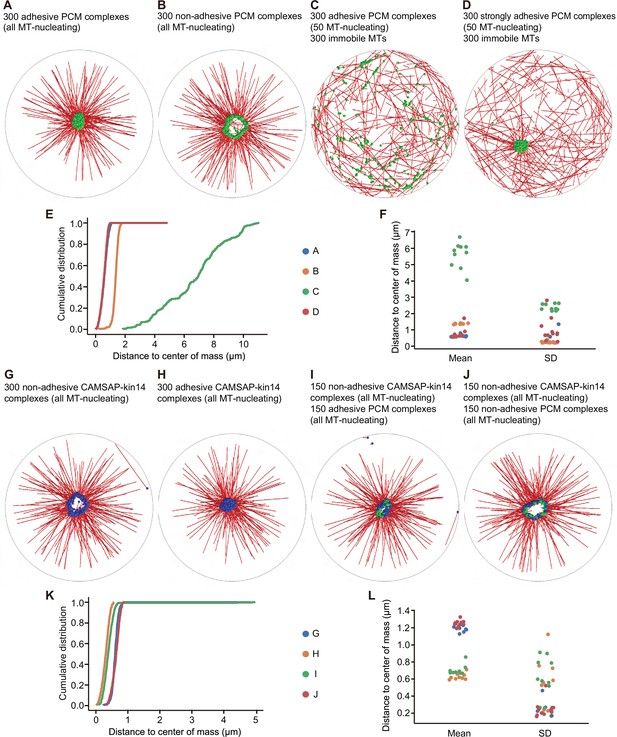
Cytosim simulations of PCM and microtubule self-organization.
In all simulations, we considered a circular cell with a radius of 10 µm (gray line), containing microtubules (red), PCM complexes (green), and CAMSAP-kin14 complexes (blue). (A) Simulation of 300 PCM complexes; each PCM complex is attached to a dynein motor, and from each complex, a MT can grow. A weak adhesive interaction is introduced between the PCM complexes. A compact PCM cluster is observed. (B) The same simulation as in (A), but with the adhesive interaction disabled; PCM is organized in a ring-like structure. (C) The simulation in (A) modified in two ways: a randomly organized, dynamic microtubule network with 300 filaments is added, and only 50 of the 300 PCM complexes can nucleate microtubules. PCM cluster formation is disrupted. (D) The same simulation as in (C), but the strength of the adhesive interaction is increased. The formation of a compact cluster is recovered. (E) Cumulative distributions of distances of the PCM complexes to the average position (the center of mass), determined from the last frame of the simulations. (F) The mean and standard deviation (SD) of the distances of the PCM complexes to the center of mass from different repetitions of the simulations. Each system was simulated 10 times. Small values for the mean and for the standard deviation indicate a compact cluster. For a ring-like structure, the mean values are larger and describe the radius of the ring. A dispersed localization of PCM complexes is characterized by large values for the mean and the standard deviation. (G) Simulation of 300 CAMSAP-kin14 complexes, which consist of a microtubule nucleation site and five kinesin-14 motors, but do not adhere to each other. The complexes form a ring-like structure. (H) The same simulation as in (G), but with complexes that have the same weak adhesive interaction as for the PCM complexes in (A). The complexes form a central compact cluster. (I) Simulation of a mixed system with 150 CAMSAP-kin14 complexes that do not adhere to each other and 150 weakly adhesive PCM complexes. All complexes associate in a central compact cluster. (J) The same simulation as in (I), but with non-adhesive PCM complexes. A ring-like structure is formed. (K) Cumulative distributions of the distances from all complexes to the center of mass of the last frame of the simulation. (L) The mean and standard deviation of the distances from all complexes to the center of mass from different repetitions of the simulations. Each system was simulated 10 times. A compact cluster is characterized by a small value for the mean and for the standard deviation. A ring-like structure has a larger mean value.
-
Figure 8—source data 1
An Excel sheet with numerical data of the quantifications shown in panels E, F, K, and L.
- https://cdn.elifesciences.org/articles/77892/elife-77892-fig8-data1-v2.xlsx
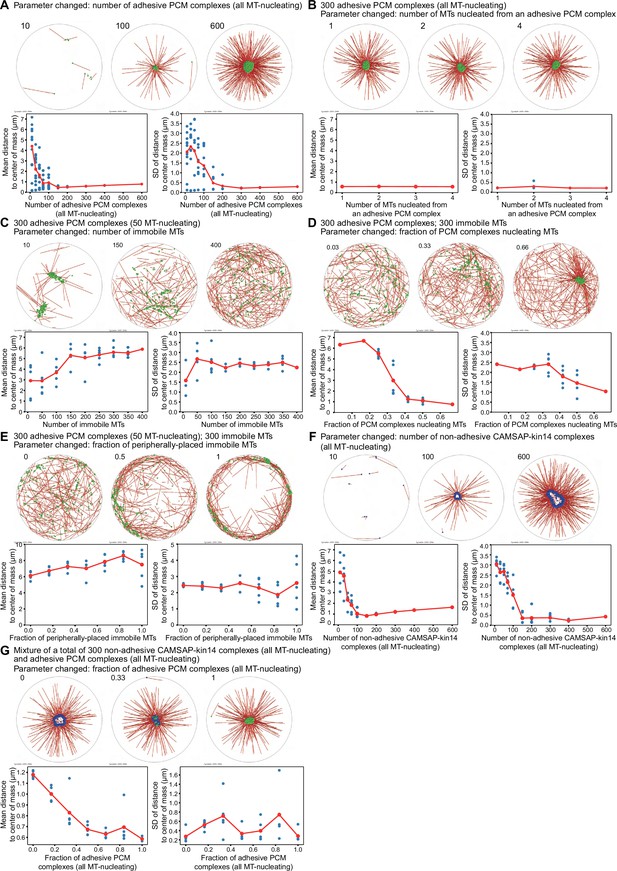
Systematic alteration of the composition of the Cytosim simulations of PCM and microtubule self-organization.
Each panel consists of five subpanels. The top row displays three examples of the end state of a simulation for different parameters. The specific parameter that was varied is given in the title, and the numerical value is indicated on the upper left on each panel. The two panels in the bottom row show the mean distance to the center of mass (left) and the standard deviation of the distance to the center of mass (right) as a function of the varied parameter. Each blue dot represents an individual simulation. The red dots connected with a thin line indicate the respective averaged value. All biophysical parameters for the simulations are given in Table 1, and only the number of the system components is varied. (A) The number of adhesive PCM complexes (all microtubule-nucleating) is varied from 10 to 600. For an increasing number of PCM complexes, the mean distance and its standard deviation are decreasing, indicating a cluster formation. In most of the simulations, clusters are formed if there are more than 150 PCM complexes in the system. (B) Increasing the number of microtubules that nucleate from an adhesive PCM complex does not affect the outcome of the simulations. One microtubule nucleated from an adhesive PCM complex is sufficient to observe cluster formation. (C) 300 adhesive PCM complexes of which 50 nucleate microtubules are placed in a system with varied number of immobile microtubules. By increasing the number of immobile microtubules, the cluster formation of the adhesive PCM complexes is disrupted. This randomization is displayed in an increasing mean distance to the center of mass and its standard deviation. With approximately 150–200 immobile microtubules, the system switches to a randomized organization of the adhesive PCM complexes. (D) In a system with 300 immobile microtubules and a fixed total number of 300 adhesive PCM complexes, the fraction of PCM complexes that nucleate microtubules is varied. When the fraction of PCM complexes nucleating microtubules increases, 300 immobile microtubules are not sufficient anymore to randomize the motion of PCM complexes, and a cluster forms. This cluster formation is reflected in a decreasing mean distance to the center of mass and its standard deviation. A threshold value can be identified at a fraction of about 0.33. Interestingly, at this value, the system seems to be bistable. (E) 300 adhesive PCM complexes, from which 50 nucleate microtubules, interact with a total of 300 immobile microtubules. The nucleation sites for the immobile microtubules are placed randomly within the cell with a radius of 10 µm. A subset of these microtubule nucleation sites is placed randomly in a peripheral area defined by a disc with an inner radius of 9 µm and an outer radius of 10 µm. The fraction of peripherally placed immobile microtubules is varied. Increasing this fraction results in an enrichment of the PCM complexes on the periphery. (F) Changing the number of non-adhesive CAMSAP-kin14 complexes (all microtubule-nucleating) from 10 to 600 indicates that a ring-like structure reliably forms if there are more than ~150 complexes in the system. This value for the threshold is estimated from the decreased mean distance to the center of mass and its standard deviation. Above this threshold, the mean distance to the center of mass is slightly increasing again, reflecting an increased radius of the ring-like structure. (G) By changing the number of adhesive PCM complexes (all microtubule-nucleating) in a mixture with non-adhesive CAMSAP-kin14 complex (all microtubule-nucleating), the fraction of PCM complexes is systematically altered. For all simulations, the total number of complexes is set to be 300. By increasing the fraction of adhesive PCM complexes, the ring-like structure is transformed into a cluster. This transition is indicated by a decrease of the mean distance to the center of mass and a threshold value can be estimated at a fraction of 0.5.
-
Figure 8—figure supplement 1—source data 1
An Excel sheet with numerical data of the shown quantifications.
- https://cdn.elifesciences.org/articles/77892/elife-77892-fig8-figsupp1-data1-v2.xlsx
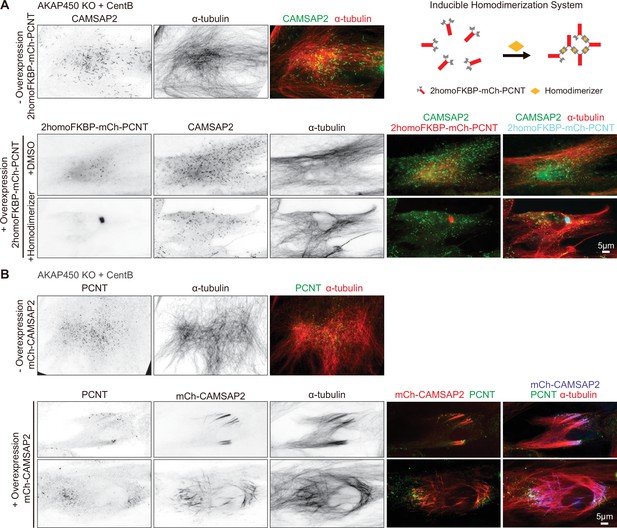
Manipulation of pericentrin localization in acentriolar AKAP450 knockout RPE1 cells through pericentrin homodimerization and CAMSAP2 overexpression.
(A) Immunofluorescence images of centrinone-treated AKAP450 knockout cells with or without overexpression of 2homoFKBP-mCherry-ericentrin (PCNT) and treated with DMSO or B/B homodimerizer, stained for CAMSAP2 (green) and microtubules (α-tubulin, red). (Top right) Scheme showing inducible self-association of pericentrin. (B) Immunofluorescence images of centrinone-treated AKAP450 knockout cells with or without overexpression of mCherry-CAMSAP2 and stained for pericentrin (green) and microtubules (α-tubulin, red).
Videos
Cell cycle progression in acentriolar AKAP450/CAMSAP2/p53 knockout cells.
Cell cycle progression visualized by phase-contrast imaging of centrinone-treated AKAP450/CAMSAP2/p53 knockout RPE1 cells. The cells were imaged for ~15 hr with 1 min interval. Time is hh:mm.
caMTOC disassembly during nocodazole treatment of acentriolar AKAP450/CAMSAP2 knockout cells.
PCM dynamics visualized by stable expression of GFP-CDK5RAP2 (green) in centrinone-treated AKAP450/CAMSAP2 knockout RPE1 cells. Microtubules were labeled overnight with 100 nM SiR-tubulin (red). The cell was imaged for ~3.5 min (100 frames, 2 s interval) prior to the addition of 10 μM nocodazole. Time is min: s.
Depletion of pericentrin inhibits PCM clustering in acentriolar AKAP450/CAMSAP2 knockout cells.
A pericentrin-depleted acentriolar AKAP450/CAMSAP2 knockout cell stably expressing GFP-CDK5RAP2 (green) and labeled overnight with 100 nM SiR-tubulin (red) was imaged for ~4.5 min (140 frames, 2 s time interval) prior to treatment with 10 μM nocodazole. Nocodazole was washed out at ~20 min (frame 591), when all microtubules were depolymerized. Time is hr: min:s.
CAMSAP2 distribution and dynamics in acentriolar AKAP450 knockout cells.
An acentriolar AKAP450 knockout RPE1 cell transiently expressing mCherry-CAMSAP2 was imaged for 15 hrs (900 frames, 1 min interval). Time is hr:min.
PCM dynamics during nocodazole treatment and washout in acentriolar AKAP450 knockout cells.
An acentriolar AKAP450 knockout RPE1 cell stably expressing GFP-CDK5RAP2 (green) and labeled overnight with 100 nM SiR-tubulin (red) was imaged for ~7 min (200 frames, 2 s interval) prior to treatment with 10 μM nocodazole. Nocodazole was washed out at ~27 min (frame 801) when all microtubules were depolymerized. Time is min: s.
PCM dynamics in acentriolar AKAP450 knockout cells are inhibited by dynapyrazole.
An acentriolar AKAP450 knockout RPE1 cell stably expressing GFP-CDK5RAP2 (green) and labeled overnight with 100 nM SiR-tubulin (red) was imaged for ~12 min (350 frames, 2 s interval) prior to the dynapyrazole treatment, treated with 5 μM Dynapyrazole A for 1 hr, and then the same cell was imaged for ~12 min. Time is min: s.
Depletion of pericentrin inhibits PCM clustering in acentriolar AKAP450 knockout cells.
A pericentrin-depleted acentriolar AKAP450 knockout cell stably expressing GFP-CDK5RAP2 (green) and labeled overnight with 100 nM SiR-tubulin (red) was imaged for ~3 min (90 frames, 2 s time interval) prior to treatment with 10 μM nocodazole. Nocodazole was washed out at ~23 min (frame 701) when all microtubules were depolymerized. Time is hr: min:s.
Inducible CAMSAP2-driven radial microtubule rearrangement in an AKAP450/CAMSAP2/p53/pericentrin knockout cell.
An acentriolar AKAP450/CAMSAP2/p53/pericentrin knockout cell transiently expressing 2FKBP-mCherry-CAMSAP2 (red) and FRB-GFP-GCN4-ppKin14 (green) was imaged for 10 min (100 frames, 6 s interval) prior to treatment with 100 nM heterodimerizer. Subsequently, the cell was imaged for ~1 hr and 35 min after heterodimerizer addition. Time is hr: min: s.
Cytosim simulations of PCM and microtubule self-organization.
Example videos of our Cytosim simulations. Each simulation represents 1800 seconds of real time, and the last frame is shown in the panels of Figure 8. In all simulations, we considered a circular cell with a radius of 10 µm (gray line), containing microtubules (red), PCM complexes (green), and CAMSAP-kin14 complexes (blue). The videos correspond to panels A, B,C, D, I, and J of Figure 8, as indicated.
Tables
Parameters with numerical values used in the Cytosim simulations.
Symbol | Value | Comment | Reference | |
---|---|---|---|---|
Cell | ||||
Cell radius | 10 µm | A typical RPE cell is ~50 µm wide. Therefore, the radius that we use is by a factor of ~2 smaller. We chose a smaller size to reduce the computational costs. | Letort et al., 2016 | |
Viscosity | 1 pN s/ µm2 | Typical value for Cytosim simulations based on measurements in C. elegans embryos. | Kole et al., 2005; Letort et al., 2016; Daniels et al., 2006 | |
Thermal energy | kT | 4.2 pN nm | Thermal energy at room temperature | |
Dynein | To parametrize dynein motors, we use the consensus numerical parameters discussed in Ohashi et al. | |||
Binding rate | kon | 5 s–1 | Ohashi et al., 2019 | |
Force-free unbinding rate | k0off | 0.1 s–1 | Ohashi et al., 2019 | |
Detachment force | Fd | 2 | Ohashi et al., 2019 | |
Binding range | 75 nm | Estimated value based on the length of the motor, typically used in Cytosim simulations. | Letort et al., 2016 | |
Force-free velocity | v0 | 500 nm/s | Brenner et al., 2020 | |
Stall force | Fs | 4 pN | Belyy et al., 2016 | |
Kin14 | Because the biophysical parameters that describe a single kinesin-14 molecule are unknown, we assume numerical values as typical for kinesin-1 | |||
Binding rate | kon | 1 s–1 | Klumpp et al., 2015 | |
Force-free unbinding rate | k0off | 1 s–1 | Berger et al., 2019 | |
Detachment force | Fd | 3 pN | Pyrpassopoulos et al., 2020 | |
Binding range | 75 nm | Typical length of a molecular motor | Letort et al., 2016 | |
Force-free velocity | v0 | 800 nm/s | Carter and Cross, 2005 | |
Stall force | Fs | 7 pN | Carter and Cross, 2005 | |
Microtubules | The numerical values that we use to describe microtubule dynamics are typically used in Cytosim simulations and based on experimental measurements. The only new parameter that we introduced is the maximum length of a microtubule. | |||
Rigidity | 20 pN µm2 | Typical value used for Cytosim simulations based on experiments. | Gibeaux et al., 2017; Gittes et al., 1993 | |
Catastrophe rate | kcat | 0.026 s–1 | Published value | Gibeaux et al., 2017 |
Rescue rate | 0 | We ignore rescues for simplicity | ||
Growing force | 1.7 pN | Dogterom and Yurke, 1997 | ||
Growing speed | vg | 0.13 µm/s | Burakov et al., 2003; Letort et al., 2016 | |
Shrinkage speed | 0.272 µm/s | Burakov et al., 2003; Letort et al., 2016 | ||
Maximum length | 7.5 µm | To avoid boundary effects exerted by long microtubules pushing, we restrict microtubules to a maximum length. | ||
Adhesive interactions of PCM complexes | The biophysical parameters describing the adhesive interaction of PCM complexes are unknown. We introduced an effective model based on the implemented agents in Cytosim, and therefore the used values don’t have a physical meaning. Overall, they generate an adhesive interaction between the PCM complexes which is not too weak and not too strong compared to the other forces that arise in the system. | |||
Binding rate | 10 s–1 | |||
Binding range | 100 nm | |||
Unbinding rate | 0.01 s–1 | |||
Detachment force | 3 pN | |||
General parameters | ||||
Stiffness of all linking elements | 100 pN/ µm | Typical value for molecular motors | Gros et al., 2021; Letort et al., 2016 | |
Total simulated time | 18,000 s | Typical time scale of the experiments |
Reagent type (species) or resource | Designation | Source or reference | Identifiers | Additional information |
---|---|---|---|---|
Antibody | anti-Pericentrin (mouse monoclonal) | Abcam | Abcam Cat# ab28144, RRID:AB_2160664 | (1:500) for IF |
Antibody | anti-Pericentrin (rabbit polyclonal) | Abcam | Abcam Cat# ab4448, RRID:AB_304461 | (1:500) for IF; (1:1000) for WB |
Antibody | anti-CDK5RAP2 (rabbit polyclonal) | Bethyl Laboratories | Bethyl Cat# A300-554A, RRID:AB_477974 | (1:300) for IF; (1:1000) for WB |
Antibody | anti-γ-tubulin (mouse monoclonal) | Sigma-Aldrich | Sigma-Aldrich: T6557; RRID:AB_477584 | (1:300) for IF; (1:2000) for WB |
Antibody | anti-γ-tubulin (rabbit polyclonal) | Sigma-Aldrich | Sigma-Aldrich:T3559, RRID:AB_477575 | (1:300) for IF |
Antibody | anti-NEDD1 (mouse monoclonal) | Abnova | Abnova Corporation Cat# H00121441-M05, RRID:AB_534956 | (1:300) for IF |
Antibody | anti-NEDD1 (rabbit polyclonal) | Rockland | Rockland Cat# 109–401 C38S, RRID:AB_10893219 | (1:1000) for WB |
Antibody | anti-Ninein (rabbit polyclonal) | BETHYL | Bethyl Cat# A301-504A, RRID:AB_999627 | (1:300) for IF; (1:2000) for WB |
Antibody | anti-Ninein (mouse monoclonal) | Santa Cruz Biotechnology | Santa Cruz Biotechnology Cat# sc-376420, RRID:AB_11151570 | (1:300) for IF |
Antibody | anti-Dynein HC (rabbit polyclonal) | Santa Cruz Biotechnology | Santa Cruz Biotechnology Cat# sc-9115, RRID:AB_2093483 | (1:300) for IF; (1:500) for WB |
Antibody | anti-p150Glued (mouse monoclonal) | BD Biosciences | BD Biosciences Cat# 610473, RRID:AB_397845 | (1:100) for IF; (1:500) for WB |
Antibody | anti-PCM1 (mouse monoclonal) | Santa Cruz Biotechnology | Santa Cruz Biotechnology Cat# sc-398365, RRID:AB_2827155 | (1:300) for IF |
Antibody | anti-PCM1 (rabbit polyclonal) | Bethyl Laboratories | Bethyl Cat# A301-150A, RRID:AB_873100 | (1:300) for IF |
Antibody | anti-AKAP450 (mouse monoclonal) | BD Biosciences | BD Biosciences Cat# 611518, RRID:AB_398978 | (1:500) for WB |
Antibody | anti-CAMSAP2 (rabbit polyclonal) | Proteintech | Proteintech Cat# 17880–1-AP, RRID:AB_2068826 | (1:200) for IF; (1:1000) for WB |
Antibody | anti-p53 (mouse monoclonal) | Santa Cruz Biotechnology | Santa Cruz Biotechnology Cat# sc-126, RRID:AB_628082 | (1:300) for IF; (1:1000) for WB |
Antibody | anti-p53 (rabbit polyclonal) | BETHYL | Bethyl Cat# A300-248A, RRID:AB_263349 | (1:300) for IF |
Antibody | anti-EB1 (mouse monoclonal) | BD Biosciences | BD Biosciences:610535; RRID:AB_397892 | (1:400) for IF |
Antibody | anti-EB3 (rabbit polyclonal) | Martin et al., 2018; | (1:300) for IF | |
Antibody | anti-Centrin (mouse monoclonal) | Millipore | Millipore Cat# 04–1624, RRID:AB_10563501 | (1:500) for IF |
Antibody | anti-CEP120 (rabbit polyclonal) | Thermo Fisher Scientific | Thermo Fisher Scientific Cat# PA5-55985, RRID:AB_2639665 | (1:300) for IF |
Antibody | anti-CEP135 (rabbit polyclonal) | Sigma-Aldrich | Sigma-Aldrich:SAB4503685; RRID:AB_10746232 | (1:300) for IF |
Antibody | anti-CEP152 (rabbit polyclonal) | Abcam | Abcam, Cat # ab183911 | (1:300) for IF; (1:1000) for WB |
Antibody | anti-CEP170 (mouse monoclonal) | Thermo Fisher Scientific | Thermo Fisher Scientific Cat# 41–3200, RRID:AB_2533502 | (1:200) for IF |
Antibody | anti-CEP192 (rabbit polyclonal) | Bethyl Laboratories | Bethyl Cat# A302-324A, RRID:AB_1850234 | (1:300) for IF; (1:1000) for WB |
Antibody | anti-GM130 (mouse monoclonal) | BD Biosciences | BD Biosciences:610823; RRID:AB_398142 | (1:300) for IF; (1:2000) for WB |
Antibody | anti-α-tubulin YL1/2 (rat monoclonal) | Pierce | Pierce: MA1-80017; RRID:AB_2210201 | (1:300) for IF |
Antibody | anti-α-tubulin (mouse monoclonal) | Sigma-Aldrich | Sigma-Aldrich:T5168; RRID:AB_477579 | (1:400) for IF |
Antibody | anti-α-tubulin (rabbit monoclonal antibody) | Abcam | Abcam Cat# ab52866, RRID:AB_869989 | (1:800) for IF |
Antibody | Anti-β-tubulin (mouse monoclonal) | Sigma-Aldrich | Sigma-Aldrich Cat# T8660, RRID:AB_477590 | (1:2000) for WB |
Antibody | anti-CLASP1 (rabbit polyclonal) | Akhmanova et al., 2001 | (1:400) for IF | |
Antibody | anti-CLASP2 (rabbit polyclonal) | Akhmanova et al., 2001 | (1:400) for IF | |
Antibody | anti-CLIP-115 #2,238 (rabbit polyclonal) | Akhmanova et al., 2001 | (1:300) for IF | |
Antibody | anti-CLIP-170 #2,360 (rabbit polyclonal) | Akhmanova et al., 2001 | (1:300) for IF | |
Antibody | anti-ch-TOG (rabbit polyclonal) | Charrasse et al., 1998 | Dr. Lynne Cassimeris (Lehigh University, USA) | (1:200) for IF |
Antibody | anti-CPAP (rabbit polyclonal) | Kohlmaier et al., 2009 | Dr. Pierre Gönczy (EPFL, Switzerland) | (1:200) for IF |
Antibody | anti-CP110 (rabbit monoclonal) | Proteintech | Proteintech Cat# 12780–1-AP, RRID:AB_10638480 | (1:300) for IF |
Antibody | anti-KIF1C (rabbit polyclonal) | Cytoskeleton | Cytoskeleton Cat# AKIN11-A, RRID:AB_10708792 | (1:300) for IF |
Antibody | anti-KIF2A (rabbit polyclonal) | Ganem and Compton, 2004 | Dr. Duane Compton (Geisel School of Medicine at Dartmouth, USA) | (1:300) for IF |
Antibody | anti-HAUS2 (rabbit polyclonal) | Lawo et al., 2009 | Dr. Laurence Pelletier (Lunenfeld-Tanenbaum Research Institute, Canada) | (1:200) for IF |
Antibody | anti-BICD2 (rabbit polyclonal) | Hoogenraad et al., 2003 | (1:2500) for WB | |
Antibody | anti-Actin (mouse monoclonal) | Millipore | Millipore Cat# MAB1501, RRID:AB_2223041 | (1:4000) for WB |
Antibody | anti-Ku80 (mouse monoclonal) | BD Biosciences | BD Biosciences Cat# 611360, RRID:AB_398882 | (1:2000) for WB |
Antibody | anti-LaminA/C (mouse monoclonal) | BD Biosciences | BD Biosciences Cat# 612162, RRID:AB_399533 | (1:400) for IF |
Antibody | anti-Cytochrome C (mouse monoclonal) | BD Biosciences | BD Biosciences Cat# 556432, RRID:AB_396416 | (1:300) for IF |
Antibody | anti-Calnexin (rabbit polyclonal) | Abcam | Abcam Cat# ab22595, RRID:AB_2069006 | (1:300) for IF |
Antibody | Anti-Lamtor4 (rabbit monoclonal) | Cell Signaling (CST)/Bioke | Cell Signaling Technology Cat# 12284, RRID:AB_2797870 | (1:800) for IF |
Antibody | Anti-Tom20 (mouse monoclonal) | BD Biosciences | BD Biosciences Cat# 612278, RRID:AB_399595 | (1:200) for IF |
Antibody | IRDye 800CW/680LT secondaries | Li-Cor Biosciences | LI-COR Biosciences Cat# 926–32219, RRID:AB_1850025’ LI-COR Biosciences Cat# 926–68020, RRID:AB_10706161; LI-COR Biosciences Cat# 926–32211, RRID:AB_621843; LI-COR Biosciences Cat# 926–68021, RRID:AB_10706309 | (1:5000) for WB |
Antibody | Alexa Fluor 405–, 488–, and 594– secondaries | Molecular Probes/ Thermo Fisher Scientific | Molecular Probes Cat# A-11007, RRID:AB_141374; Cat# A-11034, RRID:AB_2576217; Cat# A32723, RRID:AB_2633275; Cat# A-31553, RRID:AB_221604; Cat# A-11029, RRID:AB_138404; Cat# A-11032, RRID:AB_2534091; Cat# A-11006, RRID:AB_141373; Thermo Fisher Scientific Cat# A-11012, RRID:AB_2534079 | (1:500) for IF |
Sequence-based reagent | siRNA against PCNT #1 | Gavilan et al., 2018 | 5’-AAAAGCUCUGAUU UAUCAAAAGAAG-3’ | |
Sequence-based reagent | siRNA against PCNT #2 | Gavilan et al., 2018 | 5’-UGAUUGGACGUCA UCCAAUGAGAAA-3’ | |
Sequence-based reagent | siRNA against PCNT #3 | Tibelius et al., 2009 | 5’-GCAGCUGAGCUGAAGGAGA-3’ | |
Sequence-based reagent | siRNA against CDK5RAP2 | Fong et al., 2008 | 5’-UGGAAGAUCUCCUAACUAA-3’ | |
Sequence-based reagent | siRNA against γ-tubulin #1 | Lüders et al., 2006 | 5’-GGAGGACAUGUUCAAGGAA-3’ | |
Sequence-based reagent | siRNA against γ-tubulin #2 | Vinopal et al., 2012 | 5’-CGCAUCUCUUUCUCAUAU-3’ | |
Sequence-based reagent | siRNA against Ninein | Goldspink et al., 2017 | 5’-CGGUACAAUGAGUGUAGAAUU-3’ | |
Sequence-based reagent | siRNA against PCM1 | Wang et al., 2013 | 5’-UCAGCUUCGUGAUUCUCAG-3’ | |
Sequence-based reagent | siRNA against CEP152 | Cizmecioglu et al., 2010; Komarova et al., 2005 | 5’-GCGGAUCCA ACUGGAAAUCUA-3’ | |
Sequence-based reagent | siRNA against CEP120 | Ganem et al., 2005; Lin et al., 2013 | 5’-AAUAUAUCUUCU UGCAUCUCCUUCC-3’ | |
Sequence-based reagent | siRNA against CEP192 | Sonnen et al., 2013 | 5’-CAGAGGAAUCAAUAAUAAA –3’ | |
Sequence-based reagent | siRNA against NEDD1 #1 | Lüders et al., 2006 | 5’-GCAGACAUGUGUCAAUUUA-3’ | |
Sequence-based reagent | siRNA against NEDD1 #2 | Haren et al., 2006 | 5’-GGGCAAAAGCAGACAUGUG-3’ | |
Sequence-based reagent | siRNA against DHC #1 | Splinter et al., 2010 | 5’-CGUACUCCCGUGAUUGAUG-3’ | |
Sequence-based reagent | siRNA against DHC #2 | Splinter et al., 2010 | 5’-GCCAAAAGUUACAGACUUU-3’ | |
Sequence-based reagent | siRNA against CAMSAP2 | Jiang et al., 2014 | 5’-GUACUGGAUAAAUAAGGUA-3’ | |
Sequence-based reagent | siRNA against CEP170 | Stolz et al., 2015 | 5’-GAAGGAAUCCUCCAAGUCA-3’ | |
Sequence-based reagent | siRNA against CPAP | Tang et al., 2009 | 5’-AGAAUUAGCUCGAAUAGAA-3’ | |
Sequence-based reagent | siRNA against CLIP170 #1 | Lansbergen et al., 2004; Mimori-Kiyosue et al., 2005 | 5’-GGAGAAGCAGCAGCACAUU-3’ | |
Sequence-based reagent | siRNA against CLIP170 #2 | Lansbergen et al., 2004; Mimori-Kiyosue et al., 2005 | 5’-UGAAGAUGUCAGGAGAUAA-3’ | |
Sequence-based reagent | siRNA against CLIP115 #1 | Lansbergen et al., 2004 | 5’-GGCACAGCAUGAGCAGUAU-3’ | |
Sequence-based reagent | siRNA against CLIP115 #2 | Lansbergen et al., 2004 | 5’-CUGGAAAUCCAAGCUGGAC-3’ | |
Sequence-based reagent | siRNA against ch-TOG: | Cassimeris and Morabito, 2004; Lansbergen et al., 2004 | 5’-GAGCCCAGAGUGGUCCAAA-3’ | |
Sequence-based reagent | siRNA against EB1 | Grigoriev et al., 2008; Lansbergen et al., 2004 | 5’-AUUCCAAGCUAAGCUAGAA-3’ | |
Sequence-based reagent | siRNA against EB3 | Cassimeris and Morabito, 2004; Komarova et al., 2005 | 5’-CUAUGAUGGAAAGGAUUAC-3’ | |
Sequence-based reagent | siRNA against KIF2A | Ganem et al., 2005; Grigoriev et al., 2008 | 5’-GGCAAAGAGAUUGACCUGG-3’ | |
Sequence-based reagent | siRNA against CP110 | Cizmecioglu et al., 2010; Spektor et al., 2007 | 5'-AAGCAGCAUGAGUAUGCCAGU-3' | |
Sequence-based reagent | siRNA against Luciferase | Lansbergen et al., 2004; Lin et al., 2013 | 5’-CGUACGCGGAAUACUUCGA-3’ | |
Sequence-based reagent | sgRNA target CAMSAP2 | Lansbergen et al., 2004; Wu et al., 2016 | 5’-gCATGATCGATACCCTCATGA-3 | |
Sequence-based reagent | sgRNA target p53 e2 #1 | This study | 5′-gCGTCGAGCCCCCTCTGAGTC-3′; | |
Sequence-based reagent | sgRNA target p53 e4 #2 | This study | 5′-gCCATTGTTCAATATCGTCCG-3′; | |
Sequence-based reagent | sgRNA target PCNT e5-1 #1 | This study | 5′-gAGACGGCATTGACGGAGCTG-3′; | |
Sequence-based reagent | sgRNA target PCNT e5-2 #2 | This study | 5′-GCTCAACAGCCGGCGTGCCC-3′; | |
Sequence-based reagent | p53 KO sequencing primer F | This study | 5′-TCAGACACTGGCATGGTGTT-3′; | |
Sequence-based reagent | p53 KO sequencing primer R | This study | 5′-AGAAATGCAGGGGGATACGG-3′; | |
Sequence-based reagent | PCNT KO sequencing primer F | This study | 5′-ATACAGCGAGGGAATTCGGG-3′; | |
Sequence-based reagent | PCNT KO sequencing primer R | This study | 5′-TAGAATGCCCACACCGAGC-3′; | |
Sequence-based reagent | Forward primer for PCR of tagBFP to generate pB80-FRB-TagBFP-GCN4-ppKin14 | This study | 5′-TCTCAAAGCAATTGT CGACAGGATCCGC TGGCTCCGCTGCTG GTTCTGGCGAATTCA GCGAGCTGATTA AGGAGAACA-3′; | |
Sequence-based reagent | Reverse primer for PCR of tagBFP to generate pB80-FRB-TagBFP-GCN4-ppKin14 | This study | 5′-ATAGCGGAGCC TGCTTTTTTGTACA CATTAAGCTTGTG CCCCAGTTTG-3′; | |
Sequence-based reagent | Forward primer for PCR of tagBFP to generate pB80-FRB-HA-GCN4-ppKin14 | This study | 5′-TCTCAAAGCAAT TGTCGACATACCCATA CGATGTTCCAGAT TACGCTGTGTAC AAAAAAGCAGGCTCC-3′; | |
Sequence-based reagent | Reverse primer for PCR of tagBFP to generate pB80-FRB-HA-GCN4-ppKin14 | This study | 5′-GGAGCCTGCTTT TTTGTACACAG CGTAATCTGG AACATCGTATGG GTATGTCGACAA TTGCTTTGAGA-3′; | |
Chemical compound | Centrinone B | Tocris Bioscience | Tocris Bioscience Cat # 5,690 | 125 nM |
Chemical compound | Nocodazole | Sigma-Aldrich | Sigma-Aldrich, Cat # M1404-10MG | 10 μM |
Chemical compound | Rapalog (A/C Heterodimerizer) | Takara | Takara, Cat # 635,056 | 50 nM (fixation), 100 nM (live imaging). |
Chemical compound | Rapalog (B/B Homodimerizer) | Takara | Takara, Cat # 635,060 | 500 nM (fixation) |
Chemical compound | Dynapyrazole A | Sigma-Aldrich | Sigma-Aldrich, Cat # SML2127-25MG | 5 μM |
Chemical compound | BI2536 | Selleckchem | Selleckchem, Cat # S1109 | 500 nM |
Chemical compound | Thymidine | Sigma-Aldrich | Sigma-Aldrich, Cat # T9250-25G | 5 mM |
Chemical compound | proTAME | Boston Biochem | Boston Biochem, Cat # I-440 | 5 μM |
Chemical compound | Brefeldin A | Peptrotech | Peptrotech, Cat # 2031560 | 5 µg/ml |
Chemical compound | SiR-tubulin | Tebu-bio | Tebu-bio, Cat # SC002 | 100 nM |
Software, algorithm | ImageJ radiality plugin | Katrukha, 2019; https://github.com/ekatrukha/radialitymap | ||
Recombinant DNA reagent | pLVX-IRES-puro (plasmid) | Clontech | ||
Recombinant DNA reagent | pLVX-GFP- CDK5RAP2-IRES-puro (plasmid) | This work | ||
Recombinant DNA reagent | pB80-FRB-TagBFP-GCN4-ppKin14 (plasmid) | This work | ||
Recombinant DNA reagent | pB80-FRB-GFP-GCN4-ppKin14 (plasmid) | This work | ||
Recombinant DNA reagent | pB80-FRB-HA-GCN4-ppKin14 (plasmid) | This work | ||
Recombinant DNA reagent | 2FKBP-mCherry-CAMSAP2 (plasmid) | This work | ||
Recombinant DNA reagent | GFP-PCNT (plasmid) | This work | ||
Recombinant DNA reagent | 2homoFKBP-mCherry-PCNT (plasmid) | This work | ||
Recombinant DNA reagent | GST-DmKHC(1-421)-mNeonGreen (plasmid) | This work | ||
Cell line (Homo sapiens) | hTERT-RPE-1 | ATCC | CRL-4000 | |
Cell line (Homo sapiens) | hTERT-RPE-1 AKAP450 knockout | Wu et al., 2016 | ||
Cell line (Homo sapiens) | hTERT-RPE-1 AKAP450/ CAMSAP2 knockout | Wu et al., 2016 | ||
Cell line (Homo sapiens) | hTERT-RPE-1 AKAP450/CAMSAP2/p53 knockout | This work | ||
Cell line (Homo sapiens) | hTERT-RPE-1 AKAP450/CAMSAP2/ p53/Pericentrin knockout | This work | ||
Cell line (Homo sapiens) | hTERT-RPE-1 AKAP450/ CAMSAP2/EB1/EB3 mutant | This work | ||
Cell line (Homo sapiens) | hTERT-RPE-1 AKAP450/CAMSAP2/ CDK5RAP2/MMG/ p53/Pericentrin knockout | This work | ||
Cell line (Homo sapiens) | HEK 293T | ATCC | CRL-11268 |
Additional files
-
Supplementary file 1
Cytosim configuration file for the simulations.
The configuration file was executed with a compiled Cytosim (https://www.cytosim.org) version June 2019.
- https://cdn.elifesciences.org/articles/77892/elife-77892-supp1-v2.txt
-
Transparent reporting form
- https://cdn.elifesciences.org/articles/77892/elife-77892-transrepform1-v2.docx
-
Source data 1
Uncropped Western blots shown in this manuscript.
(A,B) Western blots showing that NEDD1 and CEP192 are present in centrinone-treated AKAP450/CAMSAP2 knockout cells shown in Figure 2—figure supplement 1A. (C-J) Western blots showing the depletion of indicated proteins in centrinone-treated AKAP450/CAMSAP2 knockout cells shown in Figure 2—figure supplement 1B. White dashed lines indicate where the blots were cut before incubation. (K) Western blot showing that 3 hrs treatment with dynapyrazole A does not affect the expression of the endogenous dynein heavy chain and the dynactin large subunit p150Glued in centrinone-treated AKAP450/CAMSAP2 knockout cells shown in Figure 4D.
- https://cdn.elifesciences.org/articles/77892/elife-77892-data1-v2.pdf
-
Source data 2
Uncropped Western blots shown in this manuscript.
(A) Western blots showing the knockout of p53 from AKAP450/CAMSAP2 knockout cell line shown in Figure 2—figure supplement 2F. (B) Western blots showing the knockout of pericentrin from AKAP450/CAMSAP2/p53 knockout cell line shown in Figure 2—figure supplement 2K. (C-D) Western blots showing expression levels of CDK5RAP2, γ-tub, and ninein (NIN) in control (-CentB) and centrinone-treated AKAP450/CAMSAP2/p53 knockout and AKAP450/CAMSAP2/p53/PCNT knockout cell lines shown in Figure 2—figure supplement 4B. (E) Western blots showing the knockout of AKAP450 and CAMSAP2 from EB1/EB3 mutant RPE1 cell line shown in Figure 2—figure supplement 5B. (F) Western blots showing the knockout of pericentrin in AKAP450/CAMSAP2/CDK5RAP2/MMG/p53 /knockout cell line shown in Figure 7—figure supplement 3G. White dashed lines indicate where the blots were cut before incubation.
- https://cdn.elifesciences.org/articles/77892/elife-77892-data2-v2.pdf