Modular UBE2H-CTLH E2-E3 complexes regulate erythroid maturation
Figures
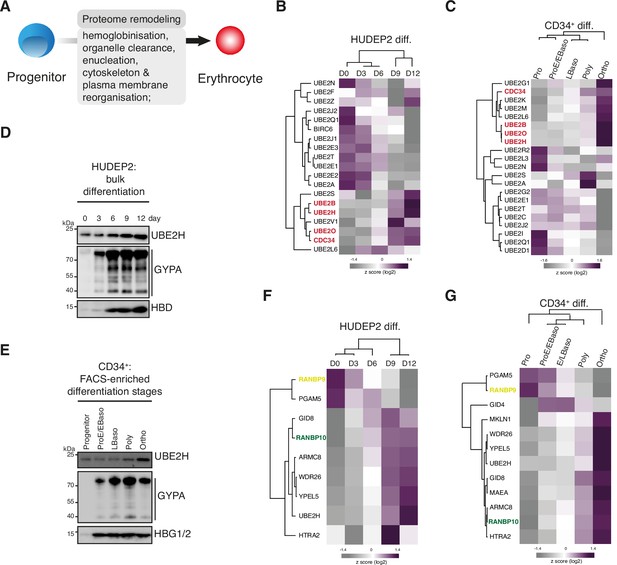
Stage-dependent expression of UBE2H and CTLH complex subunits during erythropoiesis.
(A) Cartoon indicating key features of mammalian erythropoiesis. (B) Heat map of z-scored protein abundance (log2 data-independent acquisition (DIA) intensity) of differentially expressed E2 enzymes in differentiated HUDEP2 cells. (C) Heat map of z-scored protein abundance (log2 DIA intensity) of differentially expressed E2 enzymes in differentiated CD34+ cells. (D) HUDEP2 cells were differentiated in vitro and analysed by immunoblotting with indicated antibodies. (E) CD34+ cells were differentiated in vitro, cell populations enriched by FACS, and analysed by immunoblotting with indicated antibodies. (F) Heat map of z-scored protein abundance (log2 DIA intensity) of differentially expressed CTLH complex subunits in differentiated HUDEP2 cells. (G) Heat map of z-scored protein abundance (log2 DIA intensity) of differentially expressed CTLH complex subunits in differentiated CD34+ cells.
-
Figure 1—source data 1
Original, uncropped scans of immunoblots.
- https://cdn.elifesciences.org/articles/77937/elife-77937-fig1-data1-v1.pdf
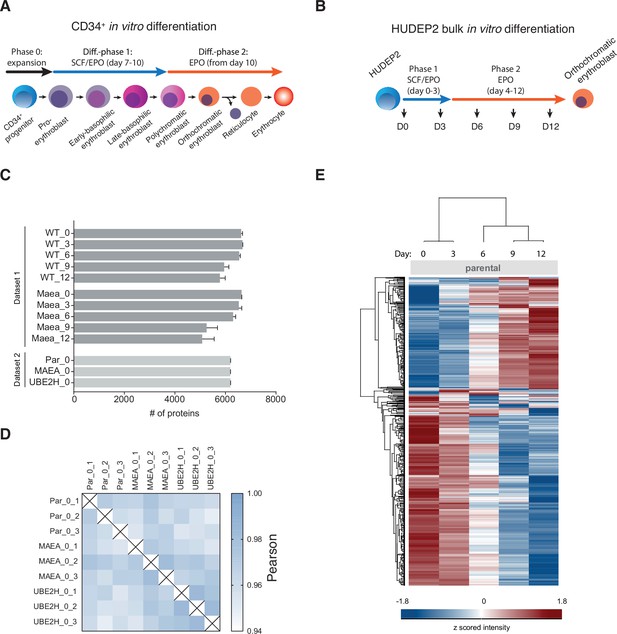
Determination of differentiation stage-dependent proteomes from in vitro differentiated HUDEP2 cells.
(A) Schematic with two-phase culture conditions of in vitro reconstituted erythropoiesis of CD34+ cells. Stem cell factor, SCF; erythropoietin, EPR. (B) Schematic with culture conditions of in vitro reconstituted erythropoiesis of HUDEP2 cells. (C) Number of different proteins quantified in each differentiation stage (days 0, 3, 6, 9, 12) of HUDEP2 parental (WT) and MAEA-/-cl3-1 cells (Dataset 1), and HUDEP2 parental, MAEA-/-cl3-1, and UBE2H-/-cl13 (Dataset 2). The mean value ± SD of three biological replicates is shown. (D) Correlation-based analysis illustrating reproducibility between biological replicates. High (1.0) and lower (0.94) Pearson correlation are indicated in blue and white, respectively. (E) Heat map of z-scored protein abundance (log2 data-independent acquisition (DIA) intensity) of 2771 differentially expressed proteins in differentiated HUDEP2 cells (ANOVA, false discovery rate [FDR] < 0.01). Differentiation time stages correspond to schematic in (B).
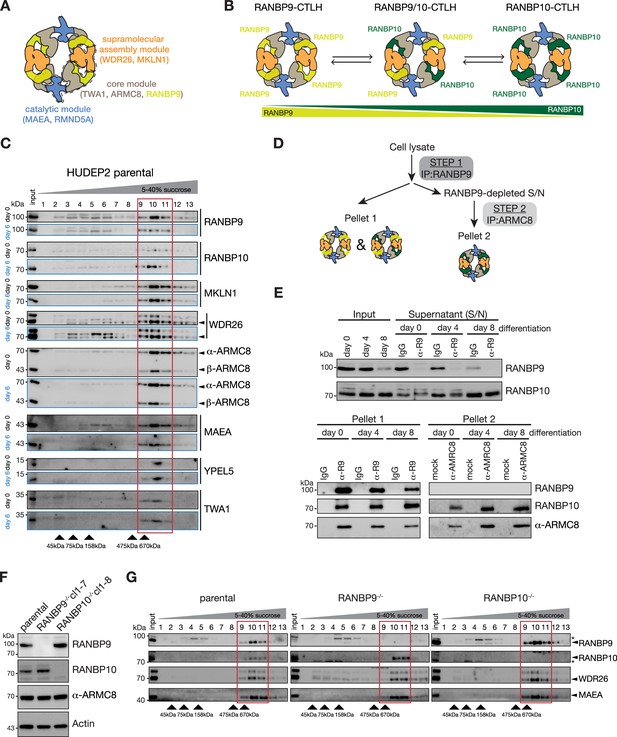
Erythroid maturation stage-dependent modulation of RANBP9- and RANBP10-assembled CTLH complexes.
(A) Cartoon of the supramolecular RANBP9-CTLH assembly indicating the catalytic (blue), core (grey/yellow), and supramolecular assembly (orange) modules. (B) Model of remodelling RANBP9- and RANBP10-CTLH complexes. (C) HUDEP2 cell lysates from differentiation days 0 and 6 were separated on sucrose gradients, and fractions analysed by immunoblotting with indicated antibodies. Fractions containing supramolecular CTLH assemblies are boxed in red. (D) Workflow of the sequential immunoprecipitation (IP) to determine RANBP9- and RANBP10-CTLH complexes. (E) HUDEP2 cell lysates from differentiation days 0, 4, and 8 were subjected to sequential IPs with RANBP9 antibody and ARMC8-specific nanobody as described in (D) followed by immunoblot analysis with indicated antibodies. IgG: unspecific antibody; mock: absence of nanobody. (F) Immunoblots of lysates of HUDEP2 parental, RANBP9-/-, and RANBP10-/- cells probing for RANBP9 and RANBP10. Actin serves as loading control. (G) Sucrose gradient fractionation of HUDEP2 cell lysates from RANBP9-/- or RANBP10-/- knock out lines, fractions were analysed by immunoblotting with indicated antibodies. Fractions containing supramolecular CTLH assemblies are boxed in red.
-
Figure 2—source data 1
Original, uncropped scans of immunoblots.
- https://cdn.elifesciences.org/articles/77937/elife-77937-fig2-data1-v1.pdf
-
Figure 2—source data 2
Original, uncropped scans of immunoblots.
- https://cdn.elifesciences.org/articles/77937/elife-77937-fig2-data2-v1.pdf
-
Figure 2—source data 3
Original, uncropped scans of immunoblots.
- https://cdn.elifesciences.org/articles/77937/elife-77937-fig2-data3-v1.pdf
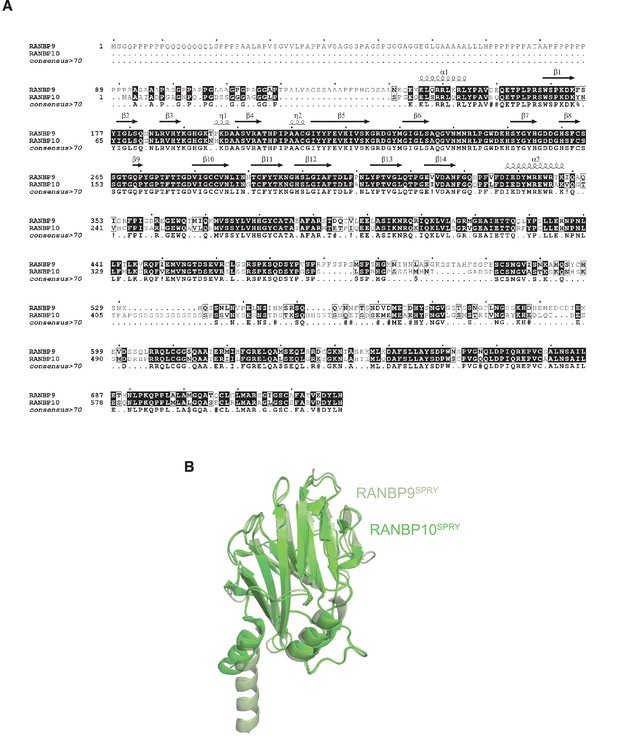
Sequence and structural alignments of human RANBP9 and RANBP10.
(A) Alignment of human RANBP9 and RANBP10 sequences, with identical sequences highlighted in black. (B) Structural alignments of SPRY domains of RANBP9 (PDB: 7NSC, light green) and RANBP10 (PDB:5JIA, dark green).
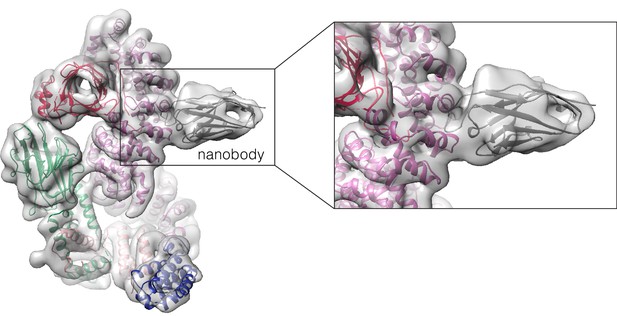
Cryo-EM structure of the ARMC8-specific nanobody bound to CTLH core (EMDB: EMD-16243).
The homology model of the nanobody (grey) and the structure of the CTLH core (colour-coded by subunits as in Figure 3C, PDB: 7NSC) was fitted in the cryo-EM density (transparent surface). The CDR loop of the nanobody contacts the C-terminal armadillo repeats of ARMC8 (purple) ranging from aa 420 to 470.
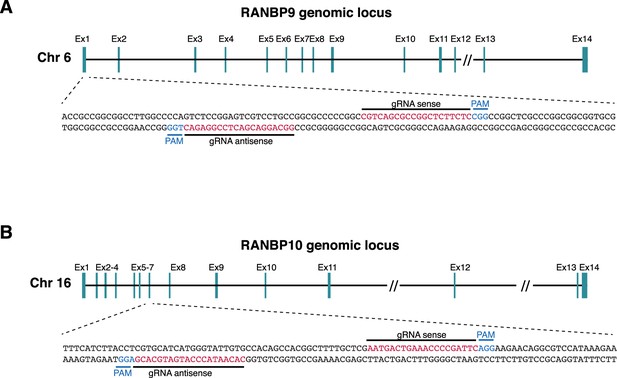
Schematics of CRISPR-Cas9 edited knockouts of RANBP9 and RANBP10.
(A) Schematics of CRISPR knockouts of RANBP9, showing exon structure and indicated guide RNAs and PAM sequences. (B) Schematics of CRISPR knockouts of RANBP10, showing exon structure and indicated guide RNAs and PAM sequences.
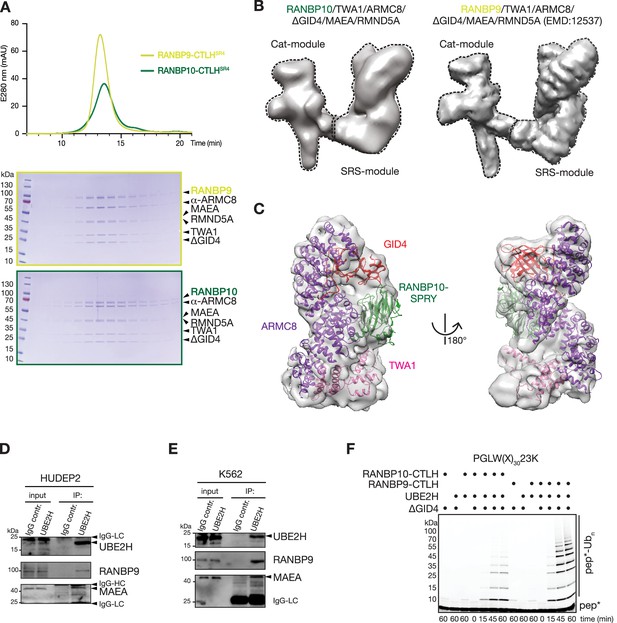
RANBP9 and RANBP10 form similar CTLH complex structures that cooperate with UBE2H to promote ubiquitin transfer.
(A) Chromatograms (top) and Coomassie-stained SDS-PAGE gels (bottom) from size-exclusion chromatography of recombinant RANBP10-CTLHSR4 and RANBP9-CTLHSR4 complexes. (B) Cryo-EM map of RANBP10-CTLHSR4 (EMDB: EMD-16242, left) and RANBP9-CTLHSR4 (EMDB: EMD-12537) (right) with Cat-module and SRS-module indicated. (C) Focused refined map of the RANBP10-CTLH SRS-module with coloured subunits: ARMC8, purple; TWA1, salmon;GID4(Δ1–99), red; RANBP10 SPRY-domain, green. (D) Immunoprecipitation (IP) from HUDEP2 cell lysates with IgG control and UBE2H-specific antibody and immunoblot analysis. (E) IP from K562 cell lysates with IgG control and UBE2H-specific antibody and immunoblot analysis. IgG light chain (IgG-LC), IgG heavy chain (IgG-HC). (F) Fluorescence scan of SDS-PAGE gels presenting time course of in vitro ubiquitylation assay with fluorescently labelled model substrate peptide PGLW(X)n-23K with lysine at position 23 (pep*) in the presence of UBE2H, RANBP10-CTLH or RANBP9-CTLH, and GID4.
-
Figure 3—source data 1
Original, uncropped scans of Coomassie-stained SDS-PAGEs and immunoblots.
- https://cdn.elifesciences.org/articles/77937/elife-77937-fig3-data1-v1.pdf
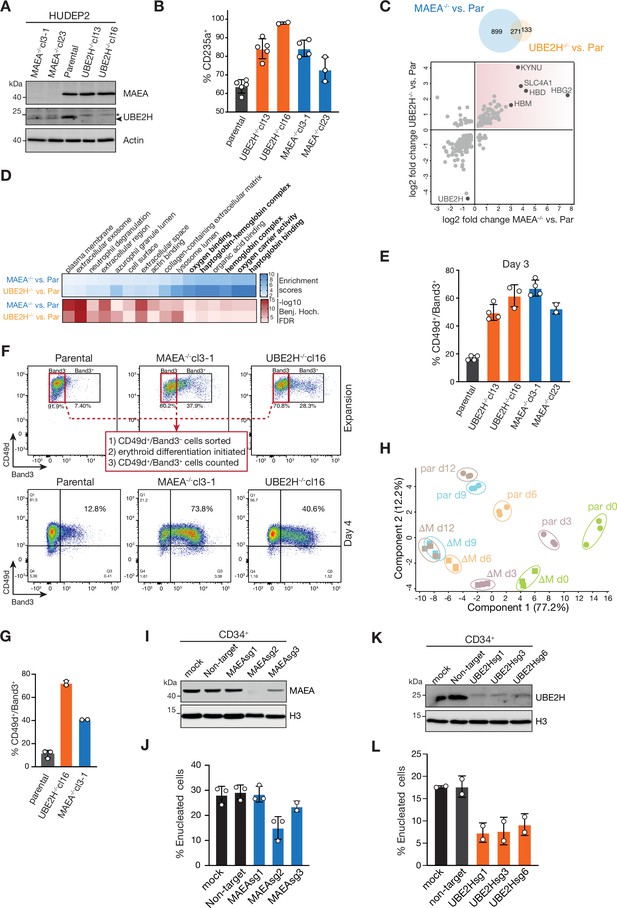
Catalytically inactive CTLH E3 complexes and UBE2H deficiency cause aberrant erythroid maturation.
(A) Immunoblots of lysates of HUDEP2 parental, MAEA-/-, and UBE2H-/- knockout clones probing for MAEA and UBE2H. Actin serves as loading control. (B) Quantitation of flow cytometry blots of indicated HUDEP2 cell lines assessing fraction of CD235a+ cells in expansion growing media condition. Error bars represent mean ± SD of n = 3–5 biological replicates. (C) Overlap between proteins with abundance differences of UBE2H-/- versus parental and MAEA-/- versus parental comparisons (top). Proteins with abundance differences (log2) of UBE2H-/- versus parental blotted against proteins with abundance differences (log2) MAEA-/- versus parental comparisons (bottom). Commonly enriched proteins are highlighted (top-right quadrant). (D) Gene Ontology (GO) enrichment analyses of upregulated protein in MAEA-/- versus parental and UBE2H-/- versus parental comparisons performed using Fisher’s exact test (Benj. Hoch. FDR 5%). (E) Quantitation of flow cytometry blots of indicated HUDEP2 cell lines assessing fraction of CD49d+/Band3+ cells at differentiation day 3. Error bars represent mean ± SD of n = 2–4 biological replicates. (F) Indicated HUDEP2 cell lines, cultured in expansion media, were sorted for CD49d+/Band3- (flow cytometry blots, top) followed by induction of erythroid maturation. Flow cytometry blots of indicated HUDEP2 cell lines showing CD49d/Band3 expression at day 4 after induced erythroid maturation. (G) Quantitation of (H) with graph showing fraction of CD49d+/Band3+ cells. Error bars represent mean ± SD of n = 2 biological replicates. (H) Principal component analysis (PCA) of erythroid differentiation stages (day 0, green; day 3, purple; day 6, orange; day 9, blue; day 12, brown) of HUDEP2 parental (par) and MAEA-/-cl3-1 (∆M) cell lines with their biological replicates based on expression profiles of selected erythroid marker proteins. (I) Immunoblot analysis of CRISPR-Cas9-mediated targeting of MAEA (MAEAsg1-3) in CD34+ cells. Histone H3 serves as loading control. (J) Quantitation of flow cytometry blots of MAEAsg-targeted cell lines assessing fraction of enucleated cells. Error bars represent mean ± SD of n = 2 biological replicates. (K) Immunoblot analysis of CRISPR-Cas9-mediated targeting of UBE2H (UBE2Hsg1-3) in CD34+ cells. Histone H3 serves as loading control. (L) Quantitation of flow cytometry blots of UBE2Hsg-targeted cell lines assessing fraction of enucleated cells. Error bars represent mean ± SD of n = 2 biological replicates.
-
Figure 4—source data 1
Original, uncropped scans of immunoblots.
- https://cdn.elifesciences.org/articles/77937/elife-77937-fig4-data1-v1.pdf
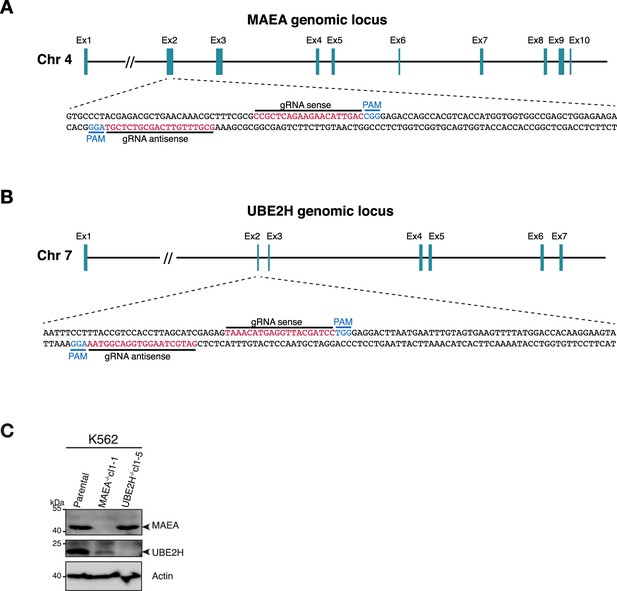
Generation of CRISPR-Cas9 edited knockouts of UBE2H and MAEA.
(A) Schematics of CRISPR knockouts of MAEA, showing exon structure and indicated guide RNAs and PAM sequences. (B) Schematics of CRISPR knockouts of UBE2H, showing exon structure and indicated guide RNAs and PAM sequences. (C) Immunoblots of lysates of K562 parental, MAEA-/-cl1-1, UBE2H-/-cl1-5 cells probing for MAEA and UBE2H. Actin serves as loading control.
-
Figure 4—figure supplement 1—source data 1
Original, uncropped scans of immunoblots.
- https://cdn.elifesciences.org/articles/77937/elife-77937-fig4-figsupp1-data1-v1.pdf
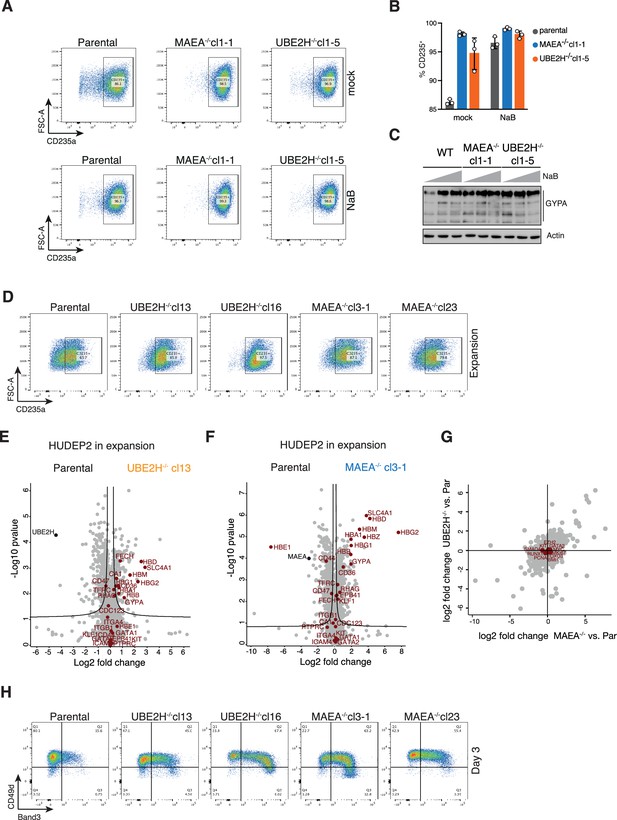
Erythroid maturation analysis of MAEA- or UBE2H-deficient K562 and HUDEP2 cells.
(A) Flow cytometry plots of mock (top) or NaB-treated (bottom) K562 parental, MAEA-/-cl1-1 and UBE2H-/-cl1-5 cell lines showing CD235a expression. (B) Quantitation of (A), with graph showing fraction of CD235a-expressing K562 parental, MAEA-/-cl1-1, UBE2H-/-cl1-5 cells in the presence of Na-butyrate (NaB) or mock treated. Error bars represent mean ± SD of n = 3 biological replicates. (C) Immunoblot analysis of cell lysates from K562 parental, MAEA-/-cl1-1, UBE2H-/-cl1-5 treated with 0, 0.3, or 0.6 mM NaB for 24 hr. Anti-GYPA/CD235a antibody detects variant forms of glycosylated GYPA. Actin serves as loading control. (D) Flow cytometry plots of indicated HUDEP2 cell lines showing CD235a expression in expansion growing media condition. (E) Volcano plots of p-values (-log10) versus protein abundance (log2) differences between parental and UBE2H-/-cl13 cells with erythroid marker proteins highlighted in red. (F) Volcano plots of p-values (-log10) versus protein abundance (log2) differences between parental and MAEA-/-cl3-1 cells with erythroid marker proteins highlighted in red. (G) Proteins with abundance difference (log2) of UBE2H-/- versus parental plotted against proteins with abundance difference (log2) of MAEA-/- versus parental comparisons. Proteins implicated in quiescence are highlighted. (H) Flow cytometry plots of indicated HUDEP2 cell lines showing CD49d/Band3 expression at day 3 after induced erythroid differentiation.
-
Figure 4—figure supplement 2—source data 1
Original, uncropped scans of immunoblots.
- https://cdn.elifesciences.org/articles/77937/elife-77937-fig4-figsupp2-data1-v1.pdf
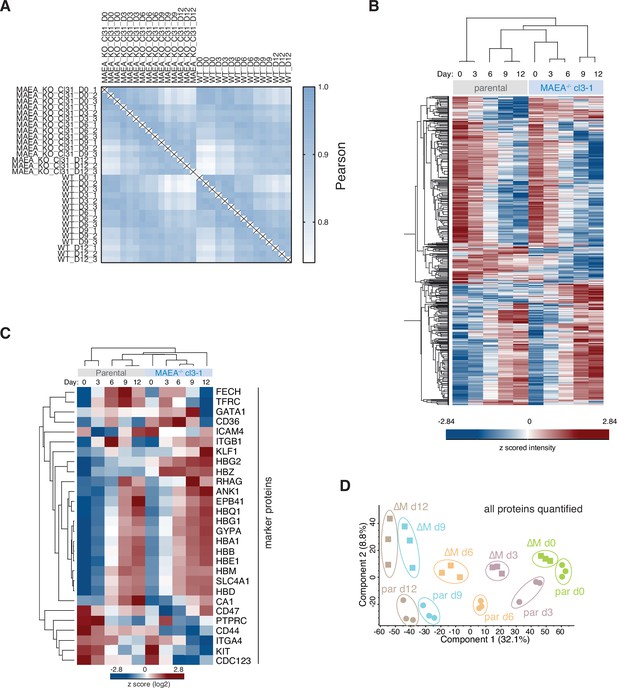
Proteome landscape of in vitro differentiated parental and MAEA-deficient HUDEP2 cells.
(A) Correlation-based analysis illustrating reproducibility between biological replicates. High (1.0) and lower (0.75) Pearson correlation are indicated in blue and white, respectively. (B) Heat map of z-scored protein abundance (log2 data-independent acquisition [DIA] intensity) of 3193 differentially expressed proteins in differentiated HUDEP2 parental and MAEA-/-cl3-1 cells (ANOVA, false discovery rate [FDR] < 0.01). (C) Heat map of z-scored protein abundance (log2 DIA intensity) of differentially expressed erythroid marker proteins in differentiating HUDEP2 parental and MAEA-/-cl3-1 cells. (D) Principal component analysis (PCA) of erythroid differentiation stages (day 0, green; day 3, purple; day 6, orange; day 9, blue; day 12, brown) of HUDEP2 parental (par) and MAEA-/-cl3-1 (∆M) cell lines with their biological replicates based on expression profiles of all quantified proteins.
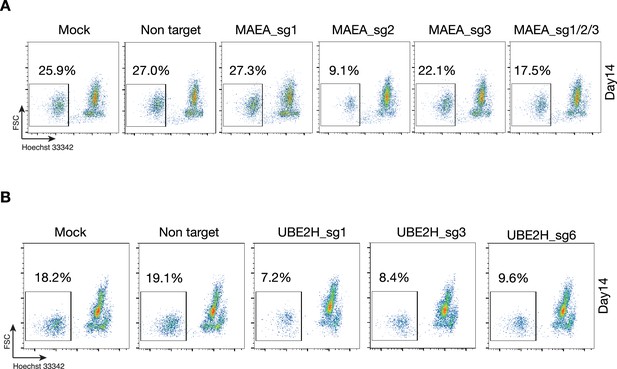
Enucleation analysis of MAEA- and UBE2H-deficient CD34+ cells during erythroid maturation.
(A) Flow cytometry plots of mock, non-target, and MAEA_short guide (sg) targeted CD34+ cell lines showing Hoechst 33342-negative cell populations (enucleated cells) of erythroid maturation at day 14. (B) Flow cytometry plots of mock, non-target, and UBE2H_sg targeted CD34+ cell lines showing Hoechst 33342-negative cell populations (enucleated cells) of erythroid maturation at day 14.
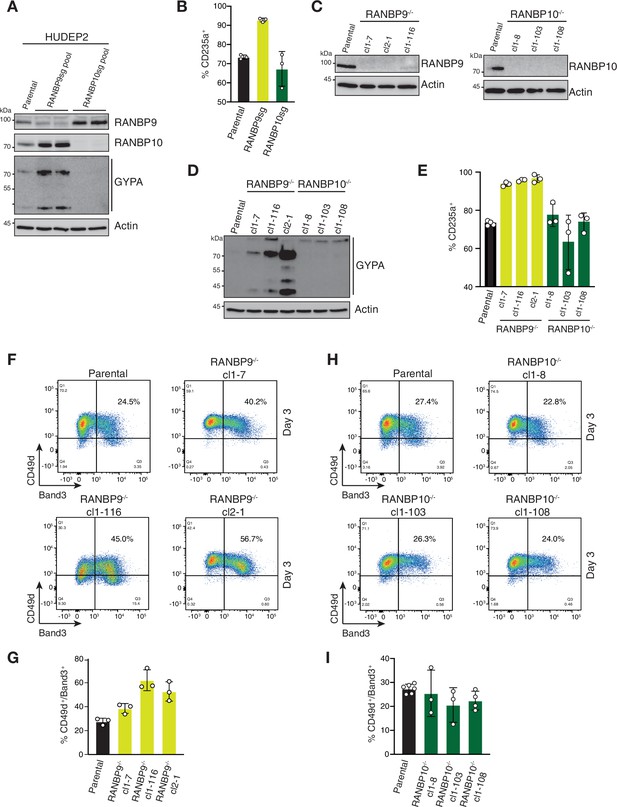
RANBP9 but not RANBP10 deficiency causes accelerated erythroid maturation.
(A) Immunoblot analysis of CRISPR-Cas9-edited (RANBP9 or RANBP10) HUDEP2 cell pools using indicated antibodies. (B) Quantitation of flow cytometry blots of indicated CRISPR-Cas9 HUDEP2 cell pools assessing fraction of CD235a+ cells in expansion growing media condition. Error bars represent mean ± SD of n = 3 biological replicates. (C, D) Immunoblot analysis of HUDEP2 RANBP9-/- or RANBP10-/- cell lines using indicated antibodies. (E) Quantitation of flow cytometry blots of indicated CRISPR-Cas9 HUDEP2 cell lines assessing fraction of CD235a+ cells in expansion growing media condition. Error bars represent mean ± SD of n = 3 biological replicates. (F, H) Flow cytometry blots of indicated HUDEP2 cell lines showing CD49d/Band3 expression at day 3 after induced erythroid maturation. (G, I) Quantitation of (F) and (H) with graphs showing fraction of CD49d+/Band3+ cells. Error bars represent mean ± SD of n = 3 biological replicates.
-
Figure 5—source data 1
Original, uncropped scans of immunoblots.
- https://cdn.elifesciences.org/articles/77937/elife-77937-fig5-data1-v1.pdf
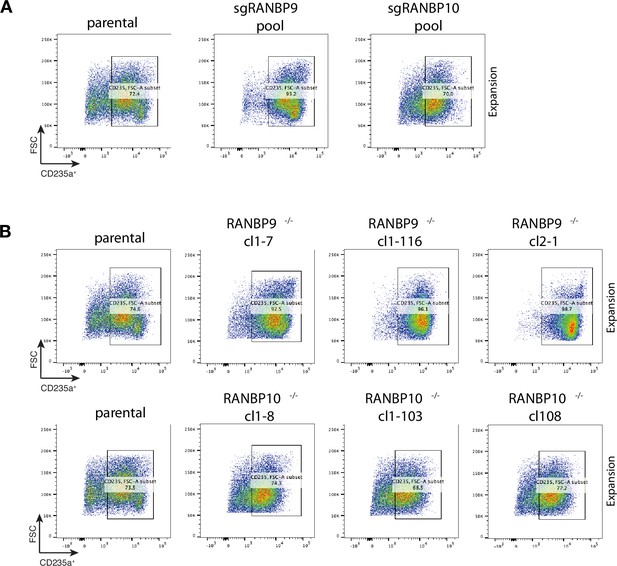
CD235a expression of RANBP9- and RANBP10-deficient HUDEP2 cells.
(A) Flow cytometry plots of expansion growing HUDEP2 cells comparing parental, sgRANBP9- and sgRANBP10 CRISPR-Cas9-targeted cells showing CD235a expression. (B) Flow cytometry plots of expansion growing parental HUDEP2, RANBP9-/- clones (cl1-7, cl1-116, cl2-1) and RANBP10-/- clones (cl1-8, cl1-103, cl1-108) showing CD235a expression.
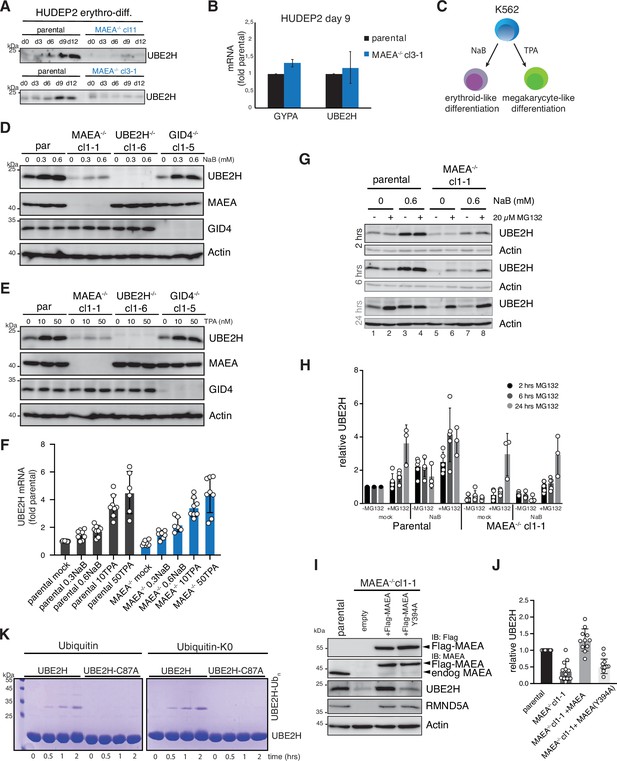
Cellular abundance of UBE2H is coupled to functional MAEA.
(A) HUDEP2 parental and MAEA-/- (clone 3-1 and 11) cells were differentiated in vitro and analysed by immunoblotting to detect UBE2H protein levels. (B) mRNA determination by RT-qPCR of HUDEP2 parental and MAEA-/-cl3-1 cells at differentiation stage day 9. Results (normalized to GAPDH) are mean ± SD of n = 2 experiments. (C) K562 cell can be either induced with Na-butyrate (NaB) for erythroid-like differentiation, or induced with 12-O-tetradodecanoyl-phorbol-13 acetate (TPA) for megakaryocyte-like differentiation. (D) K562 parental and knockout cell lines were treated with NaB for 24 hr and analysed by immunoblotting with indicated antibodies. (E) K562 parental and knockout cell lines were treated with TPA for 24 hr and analysed by immunoblotting with indicated antibodies. (F) K562 parental and MAEA-/-cl1-1 cell lines were treated with either NaB or TPA for 24 hr, and UBE2H mRNA levels determination by RT-qPCR. Results (normalized to GAPDH) are mean ± SD of n = 6–8 experiments. (G) K562 parental and MAEA-/-cl1-1 cell lines were mock- or 0.6 mM NaB-treated for 24 hr, followed by 2, 6, and 24 hr proteasome inhibition (MG132) and immunoblot analysis of cell lysates for UBE2H. Actin serves as protein loading control. (H) Quantitation of UBE2H immunoblot signals from (G) normalized to Actin and relative to parental mock values. Graph shows results by mean ± SD of n = 3–5 experiments. (I) Cell lysates of K562 parental and MAEA-/-cl1-1 cells stably expressing Flag-MAEA wildtype (WT) or mutated Flag-MAEA-Y394A (Y394A) were analysed for UBE2H protein levels by immunoblotting. (J) Quantitation of immunoblots in (I) normalized to Actin and relative to parental values. Graph shows results by mean ± SD of n = 10–12 experiments. (K) Coomassie-stained SDS-PAGE gels presenting time course of in vitro autoubiquitylation assay with either ubiquitin or lysine-less ubiquitin (ubiquitin-K0) comparing UBE2H with UBE2H-C87A.
-
Figure 6—source data 1
Original, uncropped scans of immunoblots.
- https://cdn.elifesciences.org/articles/77937/elife-77937-fig6-data1-v1.pdf
-
Figure 6—source data 2
Original, uncropped scans of immunoblots and Coomassie-stained SDS-PAGEs.
- https://cdn.elifesciences.org/articles/77937/elife-77937-fig6-data2-v1.pdf
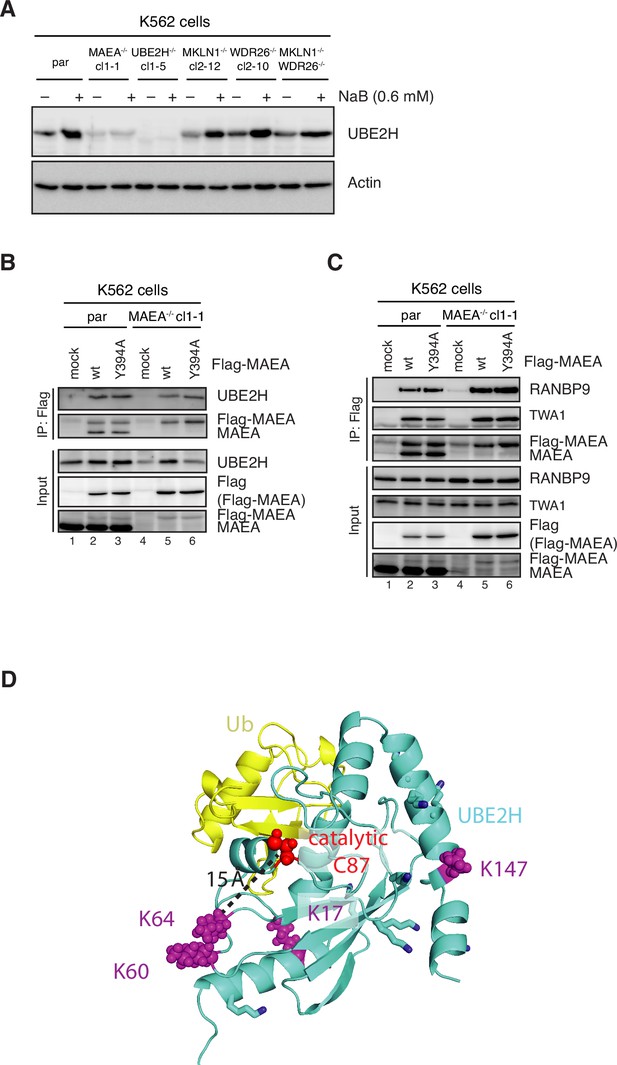
MAEA-dependent UBE2H amounts in K562 cells.
(A) K562 parental and knockout cell lines were treated with 0.6 mM NaB for 24 hr and analysed for UBE2H protein levels by immunoblotting. (B, C) K562 parental and MAEA-/-cl1-1 cells were mock, Flag-MAEA wildtype (WT), or mutated Flag-MAEA-Y394A (Y394A) transfected, and Flag-IPs from cell lysates were analysed by immunoblotting using indicated antibodies. (D) AlphaFold2 predicted structure of ubiquitin-conjugated UBE2H with catalytic Cys87 (shown in red), and potential autoubiquitylation lysine sites indicated in magenta spheres and remaining lysines indicated as sticks.
-
Figure 6—figure supplement 1—source data 1
Original, uncropped scans of immunoblots.
- https://cdn.elifesciences.org/articles/77937/elife-77937-fig6-figsupp1-data1-v1.pdf