Human muscle spindles are wired to function as controllable signal-processing devices
Figures
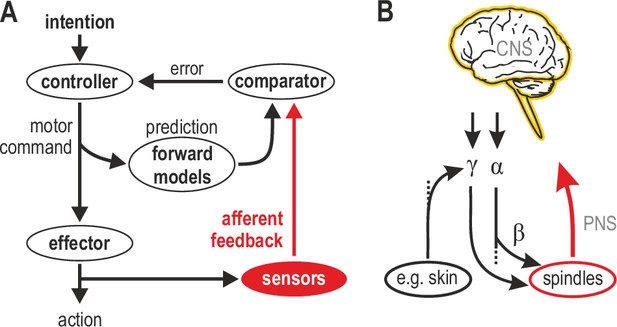
Human sensorimotor control and muscle spindle innervation.
(A) One prevalent model of human sensorimotor control. Proprioceptors in muscle and skin are viewed as basic sensors, reliably encoding actual mechanical state in unimodal coordinates. Advanced (e.g. selective) processing of sensory signals is thought to occur only in the CNS. (B) The role of muscle spindles under naturalistic efferent control has remained unclear. Mammalian muscle spindles can be powerfully controlled by γ motor neurons. These lower motor neurons are subject to both top-down and peripheral control, including from cutaneous afferents. I propose that spindles and their control enable advanced processing of sensorimotor information, giving rise to flexible coordinate representations at the level of the peripheral nervous system (PNS).
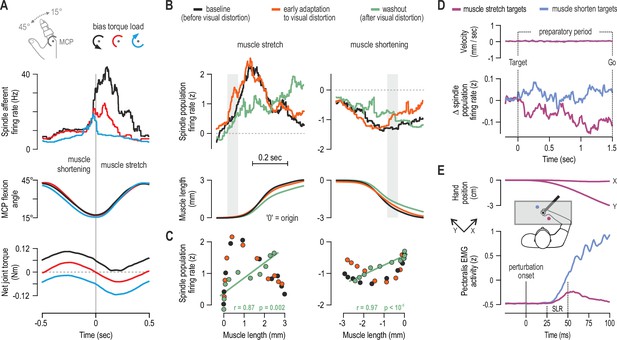
Human muscle spindle organs are not basic kinematic sensors.
(A) Averaged responses of a representative spindle afferent from the common digit extensor muscle, during active sinusoidal movements of a single finger at 1Hz (adapted from Figure 3 of Dimitriou, 2014). Movement was constrained to the metacarpophalangeal joint (MCP) and occurred under a flexion resistive or assistive torque load, or no external load. Standard classification tests identified the afferent as a typical spindle primary (i.e. ‘type Ia’; see Fig 2 in Dimitriou, 2014). Despite virtually identical finger flexion, spindle responses to stretch varied according to joint dynamics. (B) Averaged spindle afferent population responses and equivalent muscle length changes during the classic visuomotor rotation task (both ‘B’ and ‘C’ adapted from Figure 4A and 4E of Dimitriou, 2016). Grey background bars highlight phases in early adaptation (orange) that differ substantially from baseline (black). (C) Correlating the signals shown in ‘B’ (down-sampled at 50ms) confirmed a significant relationship in the washout stage. (D) Muscle velocity (null) and changes in spindle Ia responses before movement initiation in the classic instructed-delay reaching task with the hand. Ia firing rates from extensor muscles were lower when preparing movement to visual targets associated with stretch of the spindle-bearing muscle (purple). ‘D’ and ‘E’ are adapted from Figure 2B and 6A of Papaioannou and Dimitriou, 2021. (E) Averaged signals across participants; experiments using a robotic manipulandum showed a congruent goal-directed tuning of stretch reflexes, including at the short-latency epoch (‘SLR’) in cases where the homonymous muscle was not heavily loaded before perturbation. Color coding as in ‘D’.
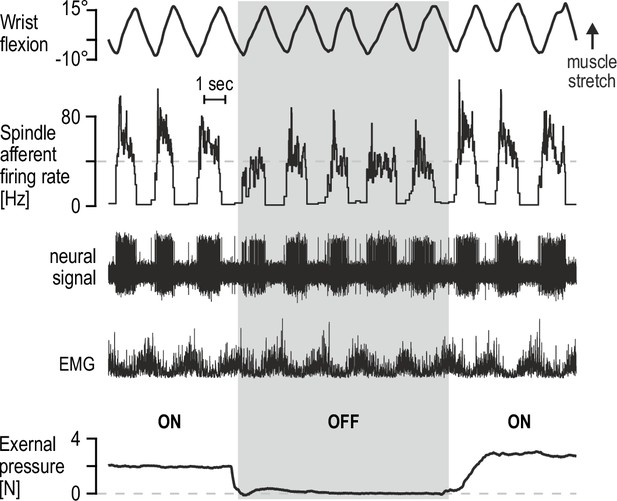
Percutaneous mechanical pressure near the spindle capsule affects encoding of active movement.
Responses of a spindle afferent from a wrist extensor muscle while the participant continuously moved their right semipronated hand about the wrist (flexion-extension; 0° denotes alignment with forearm). A hand-held probe was used by the experimenter for applying and measuring mechanical pressure over a small area of skin on the forearm (5 mm probe tip diameter), near the spindle capsule, during some movement cycles only (grey vertical bar denotes stimulus removal). Throughout, the participant’s gaze was directed at a monitor displaying a cursor that tracked hand movement. Despite very similar hand movement and activation patterns of the spindle-bearing muscle (‘EMG’), spindle responses to hand flexion were markedly stronger during percutaneous pressure.
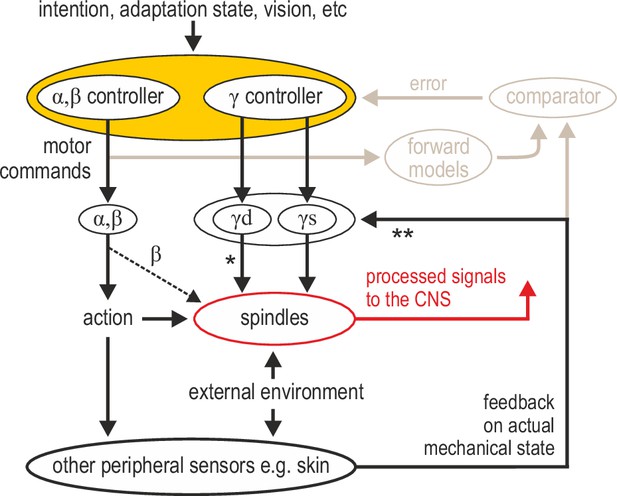
Advanced signal-processing at the level of muscle spindle organs.
In addition to descending commands to skeletal muscles and an efferent copy to forward models (Figure 1A), there can be independent descending control of γ dynamic (‘γd’) and γ static (‘γs’) spinal motor neurons. The vast majority of efferent projections to spindles are from γ motor (‘fusimotor’) neurons, but there is also some β supply (indicated by the thinner dashed line). Fusimotor control can affect spindle output in the absence of mechanical stimulation (e.g. muscle stretch), but fusimotor activity can also shape spindle responses to direct mechanical stimulation arising from own action or the external environment. ‘*’: γd project only to primary muscle spindles, allowing for differential control of primary and secondary muscle spindles. Electrophysiological studies in mammals have also demonstrated multisensory afferent convergence onto fusimotor neurons. ‘**’: The specific impact of afferent control of fusimotor neurons has not been determined yet in the active human, and may well vary across body segments e.g., stronger in the hand and/or the foot. In this model, joint and cutaneous receptors (and vision) provide consistent/reliable information about actual bodily state, and potentially so do spindles, e.g., if they are predominantly affected by direct mechanical stimulation (as in the case of the passive, unengaged individual). But here, fusimotor activity represents an intermediate coordinate transformation that allows multimodal information to converge on spindles, creating flexible representations at the periphery. So far, spindle tuning has been shown to facilitate load compensation in joint space, the selective extraction of information during motor adaptation, and the independent preparatory adjustment of reflexive muscle stiffness before goal-directed reaching (Figure 2).