Two enhancer binding proteins activate σ54-dependent transcription of a quorum regulatory RNA in a bacterial symbiont
Figures
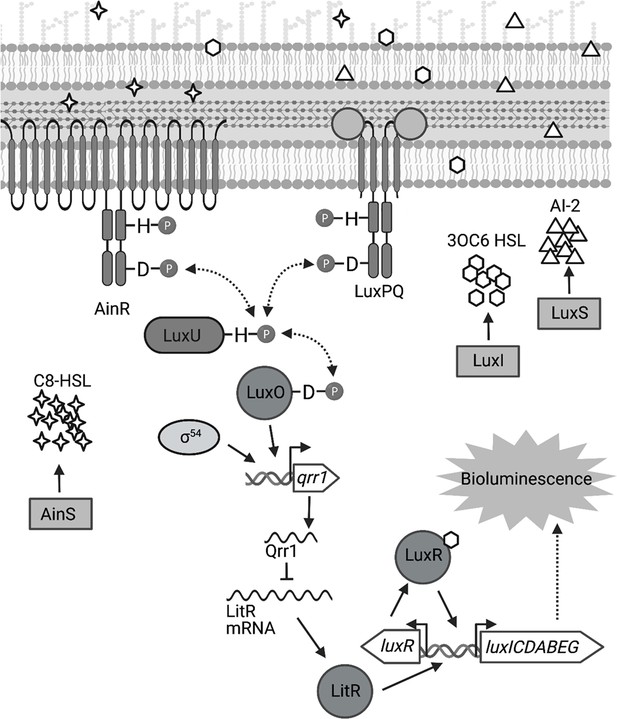
Signal transduction network underlying quorum sensing in Vibrio fischeri.
Quorum sensing in V. fischeri depends on autoinducers 3-oxo-C6 HSL (3OC6 HSL), AI-2, and C8 HSL, which are synthesized by LuxI, LuxS, and AinS, respectively. Interaction of C8 HSL or AI-2 with their cognate sensors (AinR and LuxPQ, respectively), results in lower levels of phosphorylated LuxO. Phosphorylated LuxO promotes σ54-dependent transcription of qrr1, which encodes the sRNA Qrr1. Qrr1 post-transcriptionally represses LitR, which is a positive regulator of luxR. Consequently, quorum sensing inhibits Qrr1 expression, thereby promoting bioluminescence production. Figure generated with BioRender.com.
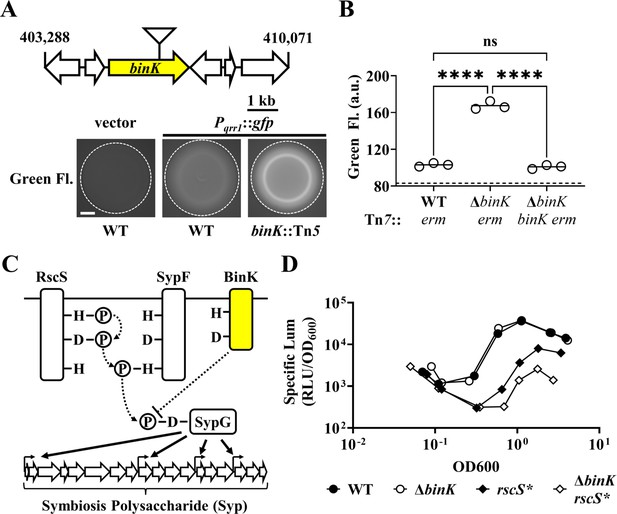
BinK inhibits the expression of the sRNA Qrr1.
(A) Top, Tn5 insertion location within VF_A0360 (binK). Below, Green fluorescence images associated with ES114 (WT) and DRO22 (binK::Tn5) harboring pTM268 (Pqrr1::gfp) or pVSV105 (vector). Dotted circle indicates border of the spot of bacterial growth resulting from placing a cell suspension on the surface of solid rich medium and incubating the sample at 28°C for 24 hr. Scale bar = 1 mm. (B) Green fluorescence levels of TIM313 (WT Tn7::erm), MJM2481 (ΔbinK Tn7::erm), and TIM412 (ΔbinK Tn7::[binK erm]) harboring pTM268. Point = green fluorescence of a spot (N = 3), bar = group mean. Dotted line = autofluorescence cutoff. One-way analysis of variance (ANOVA; F2,6 = 466.9, p < 0.0001); Tukey’s post hoc test with p-values corrected for multiple comparisons (n.s. = not significant, ****p < 0.0001). (C) Signaling pathway for Syp-dependent biofilm formation in V. fischeri ES114. Phosphoryl groups are relayed (dotted arrows) from RscS to the HPT domain of SypF for phosphotransfer to SypG. SypG activates σ54-dependent transcription of the syp locus to promote biofilm formation. BinK negatively regulates this process and likely changes the phosphorylation of SypG (directly or indirectly). (D) Top, Bioluminescence assay of ES114 (WT), MJM2251 (ΔbinK), MJM1198 (rscS*), and MJM2255 (ΔbinK rscS*). Point = specific luminescence (RLU/OD600) of indicated strain at the indicated turbidity (OD600). Shown are points derived from a representative culture (N = 3). Experimental trials: 2.
-
Figure 2—source data 1
Source data for Figure 2B, D.
- https://cdn.elifesciences.org/articles/78544/elife-78544-fig2-data1-v1.xlsx
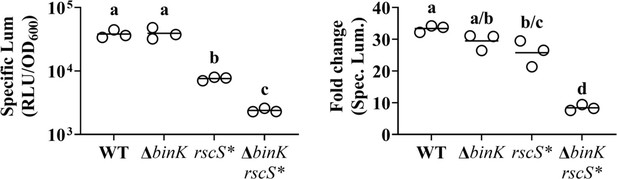
Analyses of bioluminescence assay described in Figure 2D.
Left, Point = peak specific bioluminescence derived from each culture, with bar = group mean. One-way analysis of variance (ANOVA) of log-transformed data revealed statistical significance among group means (F3,8 = 306.8, p < 0.0001); Tukey’s post hoc test with p-values corrected for multiple comparisons (same letter = not significant, different letters = p < 0.0001). Right, Point = fold change in specific bioluminescence from min to max, with bar = group mean. One-way ANOVA revealed statistical significance among group means (F3,8 = 56.23, p < 0.0001); Tukey’s post hoc test with p-values corrected for multiple comparisons (same letter = not significant, a/c = p < 0.05, c/d = p < 0.001, a/d = p < 0.0001).
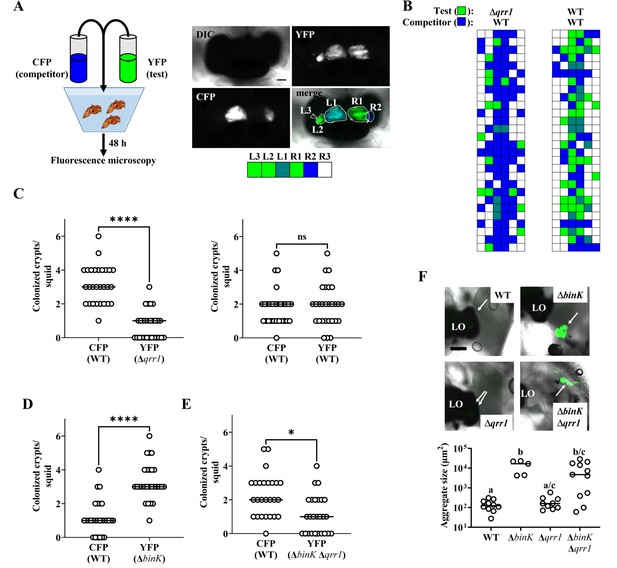
Qrr1 enhances the ability of V. fischeri to access crypt spaces.
(A) Left, experimental design of squid co-inoculation assays with YFP-labeled test strain and CFP-labeled wild-type competitor strain. Right, example image montage illustrating a light organ featuring populations comprised of cells expressing YFP or CFP. Dotted line = boundary of an individual population. Scale bar = 100 µm. Row of boxes below the image indicate the strain type(s) present within each predicted crypt space of the light organ; blue = CFP+ YFP−, green = YFP+ CFP−, hatched = CFP+ YFP+, white = CFP− YFP−. For panels B–E, experimental trials = 2. (B) Left, TIM305 (Δqrr1) as test strain. Right, ES114 (WT) as test strain. Each row represents an individual animal (N = 28). (C) Number of crypts colonized by indicated strains per squid in panel B. Wilcoxon test (****p < 0.0001, n.s. = not significant). (D) ΔbinK. Number of crypts colonized by MJM2251 (ΔbinK) as test strain. N = 27. Wilcoxon test (****p < 0.0001). (E) ΔbinK Δqrr1. Number of crypts colonized by EDR010 (ΔbinK Δqrr1) as test strain. N = 26. Wilcoxon test (*p < 0.05). (F) Aggregation assay with ES114 (WT), MJM2251 (ΔbinK), and EDR010 (ΔbinK Δqrr1) labeled with YFP. Top, merged brightfield and yellow fluorescence (green) images of aggregates (arrows) formed by indicated strains. LO = light organ. Scale bar = 200 µm. Bottom, quantification of aggregate size. Kruskal–Wallis (H = 16.79, d.f. = 3, p = 0.0008); Dunn’s post hoc test with p-values corrected for multiple comparisons (same letter = not significant, a/c and b/c = p < 0.05, a/b = p < 0.01). Experimental trials: 2.
-
Figure 3—source data 1
Source data for Figure 3B–F.
- https://cdn.elifesciences.org/articles/78544/elife-78544-fig3-data1-v1.xlsx
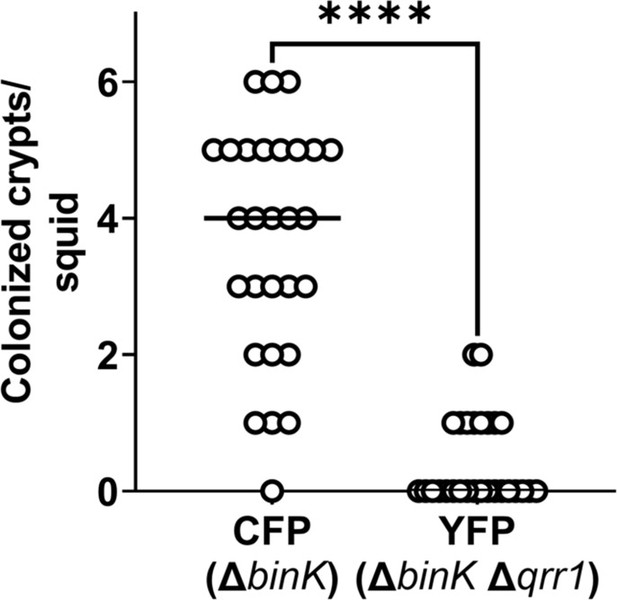
Impact of Qrr1 on crypt colonization is independent of BinK.
Number of crypts colonized by MJM2251 (ΔbinK) as CFP-labeled strain and EDR010 (ΔbinK Δqrr1) as YFP-labeled strain. N = 28. Wilcoxon test (****p < 0.0001).
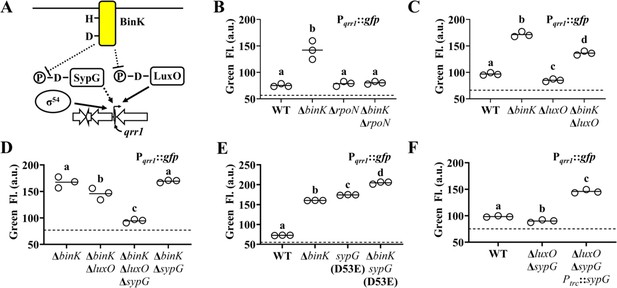
SypG activates σ54-dependent transcription of qrr1.
(A) Proposed model of BinK-dependent regulation of Qrr1 expression. (B) Green fluorescence levels of ES114 (WT), MJM2251 (ΔbinK), KRG004 (ΔrpoN), and KRG011 (ΔbinK ΔrpoN) harboring pTM268 (Pqrr1::gfp). Dotted line = autofluorescence cutoff. One-way analysis of variance (ANOVA; F3,8 = 35.69, p < 0.0001); Tukey’s post hoc test with p-values corrected for multiple comparisons (same letter = not significant, different letters = p < 0.001). (C) Green fluorescence levels of ES114 (WT), MJM2251 (ΔbinK), TIM306 (ΔluxO), and (ΔbinK ΔluxO) harboring pTM268 (Pqrr1::gfp). Dotted line = autofluorescence cutoff. One-way ANOVA (F3,8 = 367.4, p < 0.0001). (D) Green fluorescence levels of MJM2251 (ΔbinK), EDR009 (ΔbinK ΔluxO), EDR014 (ΔbinK ΔsypG), and EDR013 (ΔbinK ΔluxO ΔsypG) harboring pTM268 (Pqrr1::gfp). Dotted line = autofluorescence cutoff. One-way ANOVA (F3,8 = 60.66, p < 0.0001). (E) Green fluorescence levels of ES114 (WT), MJM2251 (ΔbinK), MJM4982 [sypG(D53E)], and MJM4983 [ΔbinK sypG(D53E)] harboring pTM268 (Pqrr1::gfp). Dotted line = autofluorescence cutoff. One-way ANOVA (F3,8 = 3921, p < 0.0001). (F) Green fluorescence levels of TIM313 (WT), EDS008 (ΔluxO ΔsypG), and EDS010 (ΔluxO ΔsypG Ptrc::sypG) harboring pEDR003 (Pqrr1::gfp) and grown on 150 µM IPTG. Dotted line = autofluorescence cutoff. One-way ANOVA (F2,6 = 438.8, p < 0.0001).
-
Figure 4—source data 1
Source data for Figure 4B–F.
- https://cdn.elifesciences.org/articles/78544/elife-78544-fig4-data1-v1.xlsx
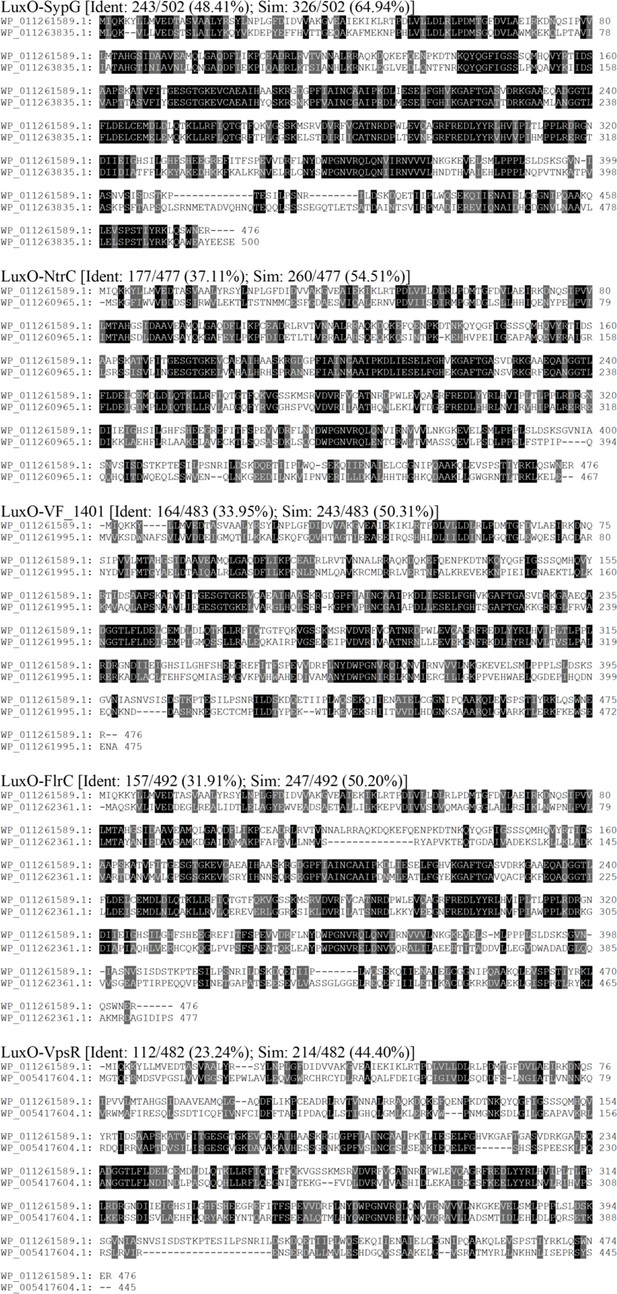
Comparisons of LuxO with other Class I bacterial enhancer binding proteins (bEBPs) encoded by V. fischeri.
Pairwise alignments of LuxO (top sequence) with indicated bEBP (bottom sequence) encoded by V. fischeri strain ES114. Amino acids highlighted in black or gray indicate identity and similarity, respectively.
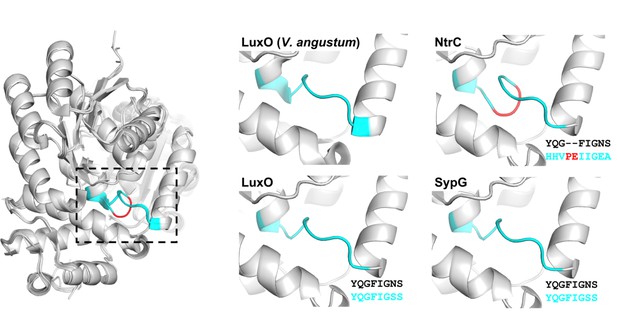
Alignment of SypG with LuxO crystal structure.
Left, overlay of homology models generated by MODELLER for the REC and AAA+ domains of LuxO (WP_011261589.1), SypG (WP_011263835.1), and NtrC (WP_011260965.1) with the crystal structure of the corresponding regions in the LuxO homolog of V. angustum (PDB 5EP0). Dotted box indicates the eight residues of the linker region between REC and AAA+ domains (cyan). The deviation within the corresponding region of NtrC is shown in red. Right, the region indicated by the box for the individual models of the indicated bacterial enhancer binding protein (bEBP). Sequences are the pairwise alignments of the eight amino acids associated with the V. angustum reference (top) and indicated bEBP (bottom).
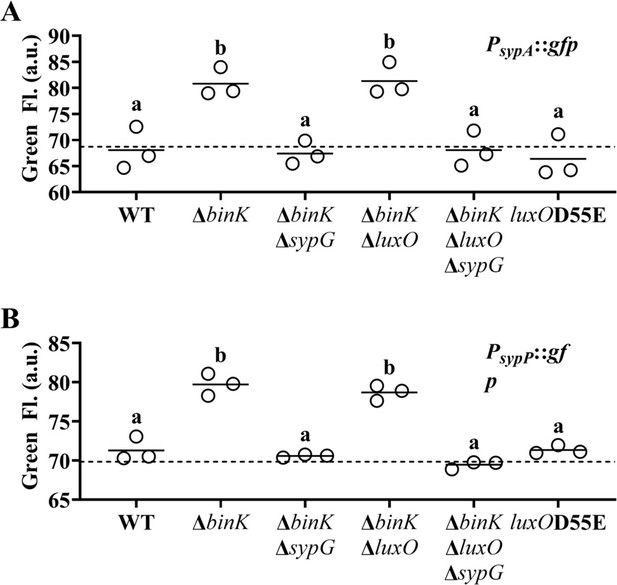
LuxO does not activate promoters of the syp locus in V. fischeri.
(A) Green fluorescence levels of ES114 (WT), MJM2251 (ΔbinK), EDR014 (ΔbinK ΔsypG), EDR009 (ΔbinK ΔluxO), EDR013 (ΔbinK ΔluxO ΔsypG), and CL59 (luxOD55E) harboring PsypA::gfp reporter plasmid pVF_A1020P. Dotted line = autofluorescence cutoff. One-way analysis of variance (ANOVA; F5,12 = 13.20, p = 0.0002). (B) Green fluorescence levels of ES114 (WT), MJM2251 (ΔbinK), EDR014 (ΔbinK ΔsypG), EDR009 (ΔbinK ΔluxO), EDR013 (ΔbinK ΔluxO ΔsypG), and CL59 (luxOD55E) harboring PsypP::gfp reporter plasmid pVF_A1035P. Dotted line = autofluorescence cutoff. One-way ANOVA (F5,12 = 61.10, p < 0.0001).
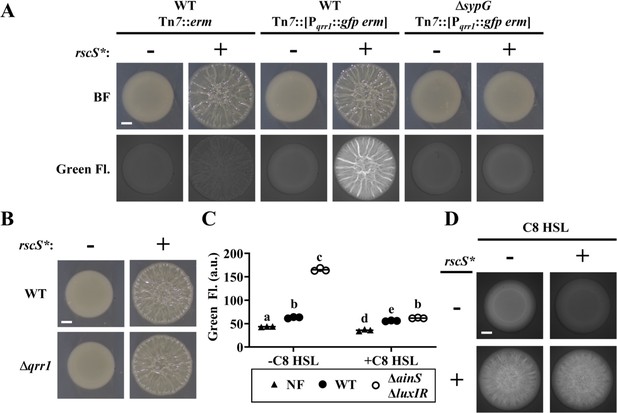
SypG activity overrides inhibition of Pqrr1 activity by quorum sensing.
(A) Brightfield (top) and green fluorescence (bottom) images of representative spots of growth (N = 3) containing TIM313 (WT Tn7::erm), TIM303 (WT Tn7::[Pqrr1::gfp erm]), or EDS015 (ΔsypG Tn7::[Pqrr1::gfp erm]) harboring plasmid pKV69 (rscS* = −) or pKG11 (rscS* = +). Scale bar = 1 mm. (B) Brightfield images of representative spots of growth (N = 3) containing TIM303 (WT) or SSC005 (Δqrr1) harboring plasmid pKV69 (rscS* = −) or pKG11 (rscS* = +). Scale bar = 1 mm. (C) Green fluorescence of ES114 (WT) and JHK007 (ΔainS ΔluxIR) harboring Pqrr1::gfp reporter pTM268 (circles) and grown ± 100 nM C8 HSL. ES114 harboring pVSV105 was used as a non-fluorescent control (NF). Two-way analysis of variance (ANOVA) revealed statistical significance for strain (F2,12 = 2809, p < 0.0001), C8 treatment (F1,12 = 2233, p < 0.0001), and their interaction (F2,12 = 1480, p < 0.0001); Tukey’s post hoc test with p-values corrected for multiple comparisons (same letter = not significant, b/e = p < 0.05, a/d = p < 0.01, other combinations of different letters = p < 0.0001). (D) Green fluorescence images of representative spots of growth (N = 3) containing KRG016 (ΔainS ΔluxIR Tn7::Pqrr1::gfp) harboring plasmid pKG11 (rscS* = +) or pKV69 (rscS* = −) on medium ± 100 nM C8 HSL. Scale bar = 1 mm.
-
Figure 5—source data 1
Source data for Figure 5C.
- https://cdn.elifesciences.org/articles/78544/elife-78544-fig5-data1-v1.xlsx
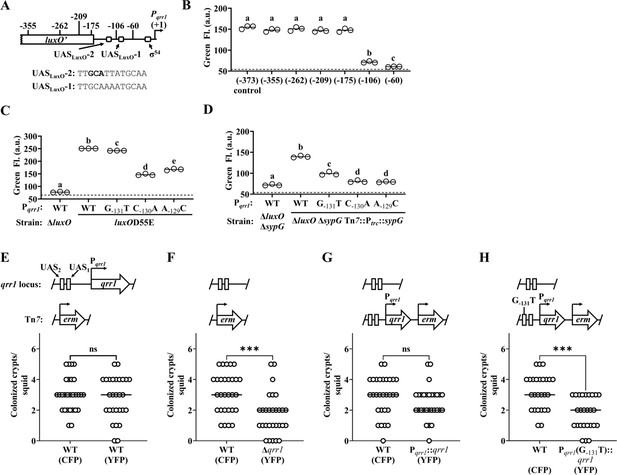
SypG activates σ54-dependent transcription of qrr1.
(A) Elements within Pqrr1 that facilitate σ54-dependent transcriptional activation. Sequence corresponds to 13 bp UASLuxO-2, with nucleotides (−131)–(−129) that were individually mutated by site-directed mutagenesis shown in bold. (B) Green fluorescence levels of EDS010 (ΔluxO ΔsypG Tn7::[Ptrc::sypG erm]) harboring Pqrr1::gfp reporter plasmids pEDR003, pEDR011 (−355), pEDR010 (−262), pEDR012 (−209), pEDR006 (−175), pEDR009 (−106), and pEDR008 (−60). Dotted line = autofluorescence cutoff. One-way analysis of variance (ANOVA; F6,14 = 411.7, p < 0.0001). (C) Green fluorescence levels of TIM306 (ΔluxO) and CL59 (luxOD55E) harboring Pqrr1::gfp reporter plasmids pEDR003, pEDS004 (G−131T), pEDS005 (C−130A), and pEDS006 (A−129C). The luxOD55E encodes a variant of LuxO that exhibits high activity in colonies. Dotted line = autofluorescence cutoff. One-way ANOVA (F4,10 = 2998, p < 0.0001). (D) Green fluorescence levels of EDS008 (ΔluxO ΔsypG) and EDS010 (ΔluxO ΔsypG Tn7::Ptrc::sypG) harboring Pqrr1::gfp reporter plasmids pEDR003 (WT), pEDS004 (G−131T), pEDS005 (C−130A), and pEDS006 (A−129C). Dotted line = autofluorescence cutoff. One-way ANOVA (F4,10 = 712.5, p < 0.0001). (E–H) Squid colonization assays, with each graph showing the number of crypts colonized by CFP-labeled TIM313 (WT) and the indicated YFP-labeled test strain. The diagram above each graph illustrates the genetic composition of the qrr1 locus (top) and Tn7 integration site (bottom) in the corresponding test strain. Test strains are TIM313 (WT), KRG021 (Δqrr1), KRG018 (Pqrr1::qrr1), and (D) KRG019 (Pqrr1 (G−131T)::qrr1). Wilcoxon test (***p < 0.001, n.s. = not significant).
-
Figure 6—source data 1
Source data for Figure 6B–H.
- https://cdn.elifesciences.org/articles/78544/elife-78544-fig6-data1-v1.xlsx
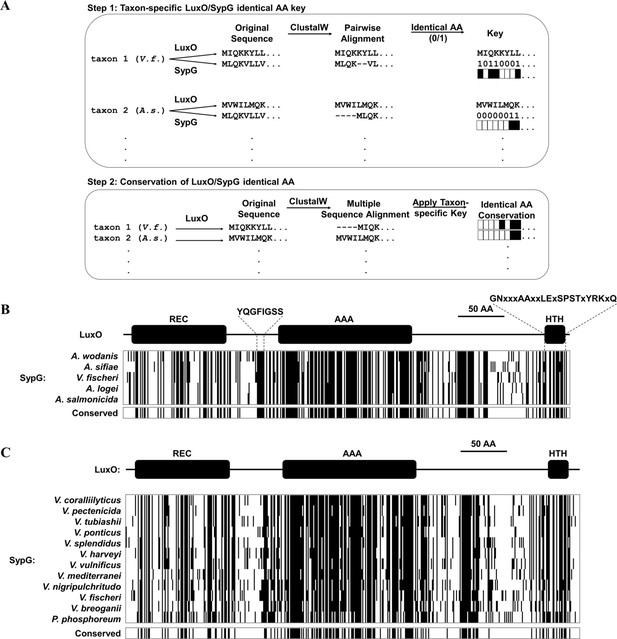
Conservation of SypG-LuxO structural features in the Vibrionaceae family.
(A) Experimental design for visualizing the extent of identity conserved between the primary structures of LuxO and SypG homologs among different taxa. (B) Each block represents a multiple sequence alignment of LuxO homologs encoded within the indicated Fischeri clade members that has 481 amino acid positions including gaps. Positions marked by a black line indicate that the corresponding amino acid of the LuxO homolog is identical to that of SypG based on pairwise alignments. Shown below each block are the positions of amino acid identity that are conserved among the indicated taxa. (C) Each block represents a multiple sequence alignment of LuxO homologs encoded within the indicated Vibrionaceae members that has 489 amino acid positions including gaps. Positions marked by a black line indicate that the corresponding amino acid of the LuxO homolog is identical to that of SypG based on pairwise alignments. Shown below each block are the positions of amino acid identity that are conserved among the indicated taxa.
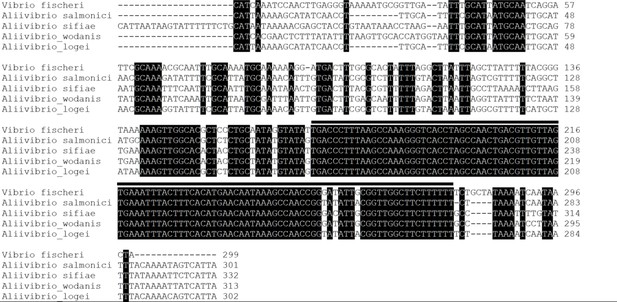
Conservation of Qrr1 within the Fischeri clade.
Region between uvrB and luxO genes in indicated taxa were aligned by MEGA X. Highlighted positions indicate 100% identity across sequences. Line above alignment indicates positions predicted to encode a Qrr1 homolog. Accessions and regions analyzed: Vibrio fischeri = NC_006840.2 [complement(1033214.1033512)], Aliivibrio salmonicida = NC_011312.1 [complement(2009689.2009989)], Aliivibrio sifiae = NZ_MSCP01000001.1 [1631724.1632055], Aliivibrio wodanis = LN554846.1 [complement(1083567.1083879)], and Aliivibrio logei = NZ_MAJU01000008.1 [810639.810940].
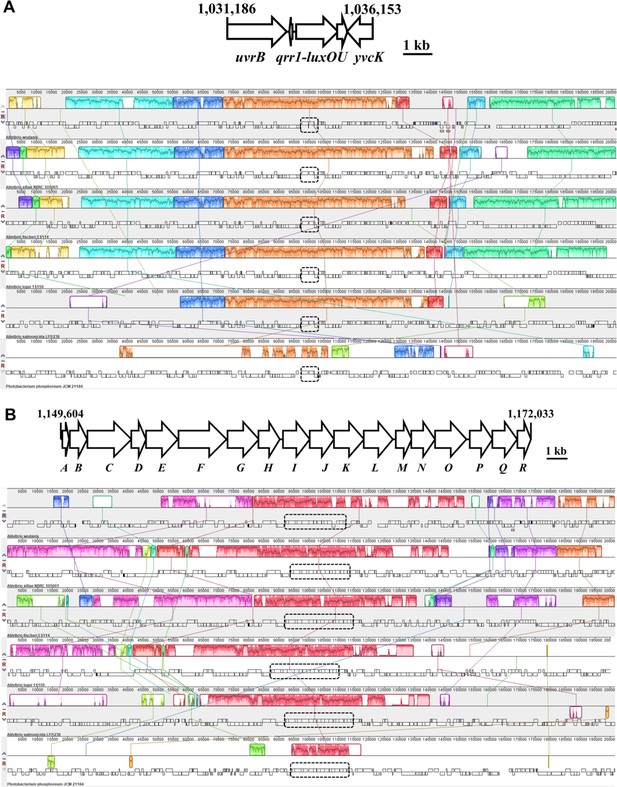
Conservation of gene synteny for luxO and sypG within the Fisheri clade.
(A) Gene synteny associated with luxO in the Fisheri clade. Top, region featuring luxO on chromosome 1 (NC_006840.2) of V. fischeri strain ES114. Bottom, progressiveMauve analysis of gene synteny associated with luxO for Fischeri clade members. Outlined regions indicate genomic blocks that are present in other taxa, with the interior plot indicating nucleotide similarity. Photobacterium phosphoreum was included as an outgroup. Accessions and regions analyzed: LN554846.1 [983877.1185307], NZ_MSCP01000001.1 [complement(1530329.1731726)], NC_006840.2 [933510.1134940], NZ_MAJU01000008.1 [complement(709196.910641)], NC_011312.1 [1909987.2111432], and NZ_MSCQ01000001.1 [complement(1671280.1872728)]. (B) Gene synteny associated with sypG in the Fisheri clade. Top, region featuring sypG on chromosome 2 (NC_006841.2) of V. fischeri strain ES114. Bottom, progressiveMauve analysis of gene synteny associated with sypG for Fischeri clade members. Outlined regions indicate genomic blocks that are present in other taxa, with the interior plot indicating nucleotide similarity. Photobacterium phosphoreum was included as an outgroup. Accessions and regions analyzed: LN554847.1 [1266858.1468330], NZ_MSCP01000002.1 [1390955.1592439], NC_006841.2 [1057508.1259010], NZ_MAJU01000009.1 [1.200000], NC_011313.1 [complement(241212.442708)], and NZ_MSCQ01000001.1 [complement(845650.1047125)].
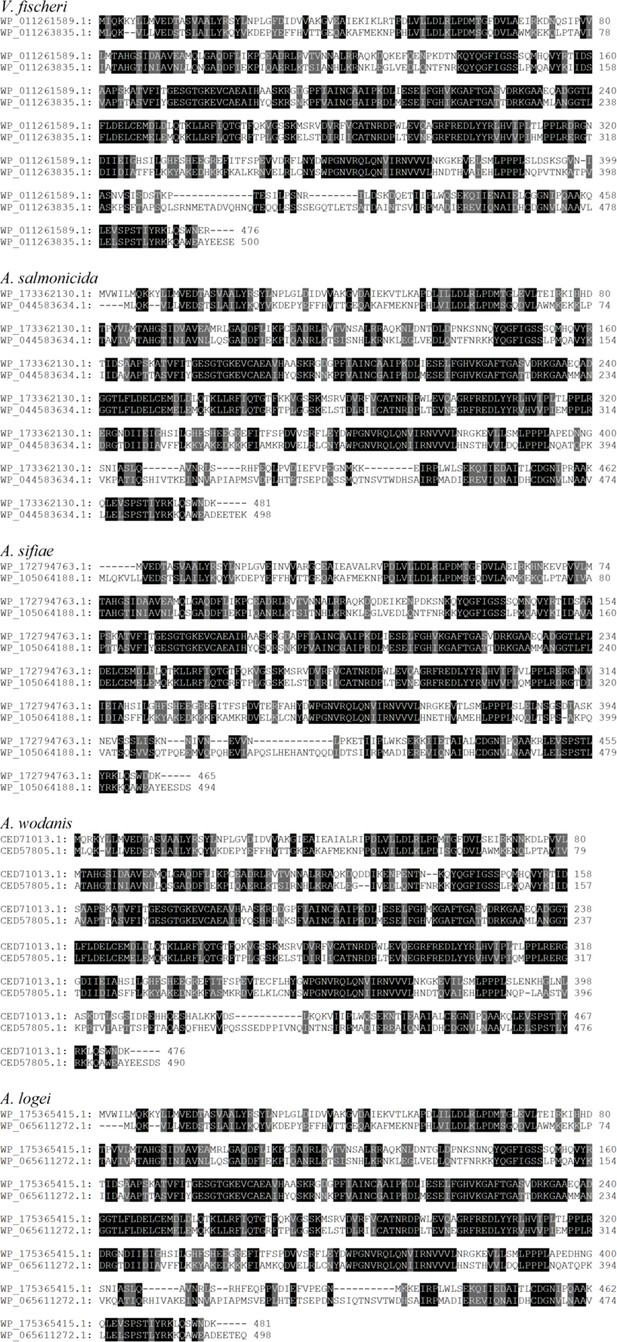
Pairwise alignments of LuxO (top sequence) and SypG (bottom sequence) homologs encoded by indicated Fischeri clade members.
Amino acids highlighted in black (gray) indicate identity (similarity).
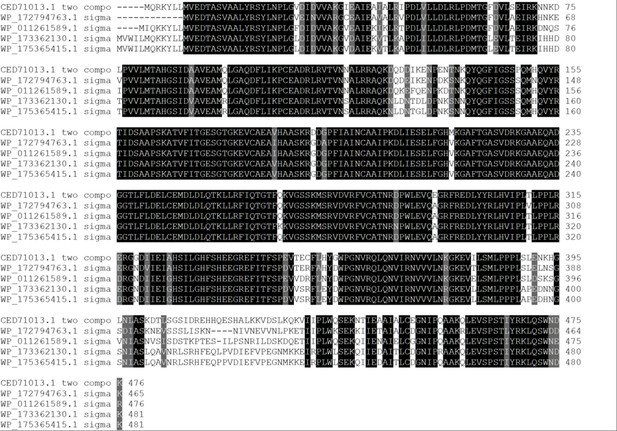
Multiple sequence alignment of LuxO homologs encoded by Fischeri clade members.
Amino acids highlighted in black or gray indicate identity and similarity, respectively, among 100% of sequences. Top to bottom: A. wodanis, A. sifiae, V. fischeri, A. logei, and A. salmonicida.
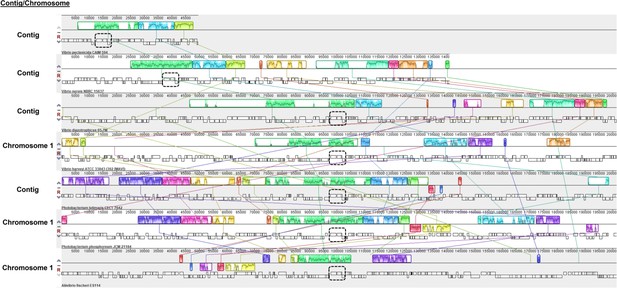
Gene synteny associated with luxO in Vibrionaceae taxa with incomplete genomes.
Analysis of gene synteny by progressiveMauve for luxO encoded by indicated Vibrionaceae members. Outlined regions indicate genomic blocks that are present in other taxa, with the interior plot indicating nucleotide similarity. The gene cluster spanning uvrB-yvcK that contains luxO is indicated by the dashed outline. The regions linked to luxO for the fully assembled genomes of V. harveyi, P. phosphoreum, and V. fischeri are included for comparisons. Accessions and regions analyzed: NZ_RSFA01000020.1 [1.49638], NZ_BCUD01000001.1 [1.140875], NZ_POSL01000002.1 [complement(144684.346084)], NZ_CP009467.1 [complement(2563561.2764964)], NZ_SNZO01000002.1 [complement(542785.744227)], NZ_MSCQ01000001.1 [complement(1671280.1872728)], and NZ_MSCQ01000001.1 [complement(1671280.1872728)].
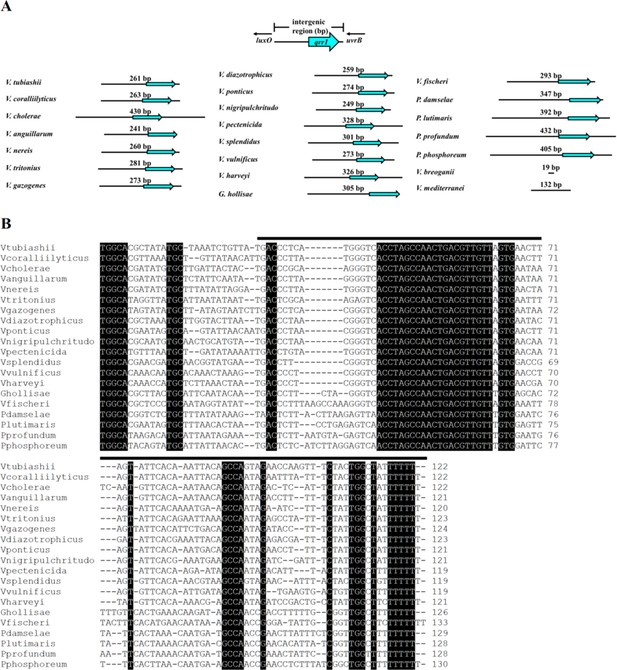
Conservation of qrr gene within uvrB-luxO intergenic region among Vibrionaceae.
(A) Cartoon indicating length in bp of uvrB-luxO intergenic region for indicated taxa. Location of putative gene encoding a Qrr is denoted by the cyan arrow. (B) Sequences of homologs of Qrr1 (denoted by the black lines) encoded within the uvrB-luxO intergenic region of each indicated taxon. Each homolog was identified by first using the locations of the −24 (GGC) and −12 (GC) sites corresponding to σ54 binding sites to determine the putative transcriptional start site and then locating the thymidine repeat corresponding to the likely terminator sequence.
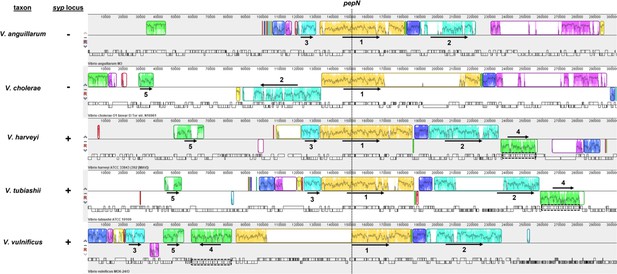
Gene synteny associated with pepN among select Vibrionaceae.
Analysis of gene synteny by progressiveMauve for PepN homolog (identified by BLAST using WP_069687131.1) encoded by indicated Vibrionaceae members. Dotted line indicates pepN gene. Outlined regions indicate locally colinear blocks (LCBs) that are present in at least two taxa, with the interior plot indicating nucleotide similarity. Numbers identify the LCBs that were used to interpret gene synteny, with the arrows indicating the relative LCB orientation. The syp locus is indicated by the dashed outline and associated with LCB 4. V. harveyi and V. tubiashii feature the syp locus downstream of LCB 2. In contrast, the syp locus of V. vulnificus is in an opposite orientation and located between LCBs 3 and 1. Neither V. anguillarum nor V. cholerae feature a syp locus. Accessions and regions analyzed: NC_022223.1 [complement(1659437.1962043)], NC_002505.1 [complement(1452881.1755487)], NZ_CP009467.1 [complement(1843797.2146403)], NZ_CP009354.1 [1461021.1763627], and NC_014965.1 [complement(1499333.1801960)].
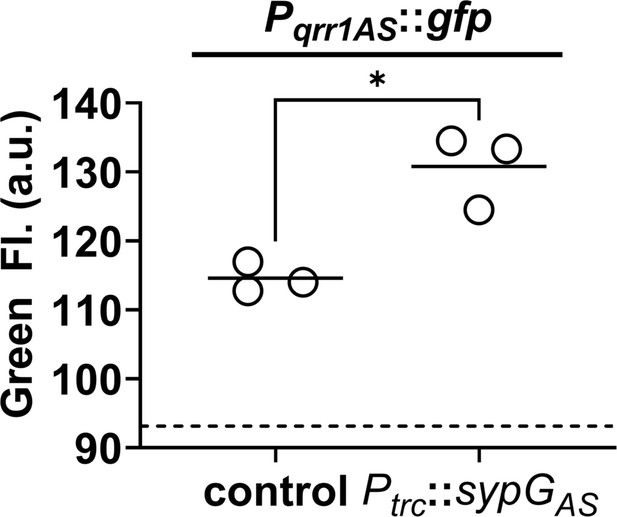
SypG-dependent transcription of qrr1 for A. salmonicida.
Green fluorescence of EDS008 (control) and EDS021 (Tn7::sypGAS) harboring pAGC003 (Pqrr1AS::gfp). N = 3. Genotypes of both strains include ΔluxO and ΔsypG alleles, as well as erm integrated at the Tn7 site. EDS008 harboring pVSV105 was used as a non-fluorescent control (dotted line). A paired t-test revealed significance between groups (*p = 0.0325). Experimental trials: 2.
-
Figure 8—source data 1
Source data for Figure 8.
- https://cdn.elifesciences.org/articles/78544/elife-78544-fig8-data1-v1.xlsx
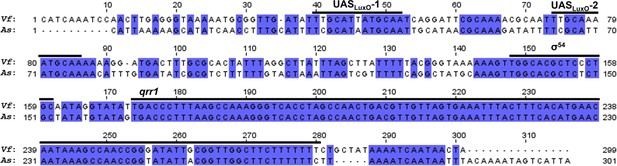
Conservation of the qrr1 locus among V. fischeri and A. salmonicida.
Pairwise alignment of luxO-uvrB intergenic region in V. fischeri (Vf) and A. salmonicida (As) generated by ClustalW in MEGA X, with identical nucleotides highlighted. Regions relevant to the expression of Qrr1 are labeled.
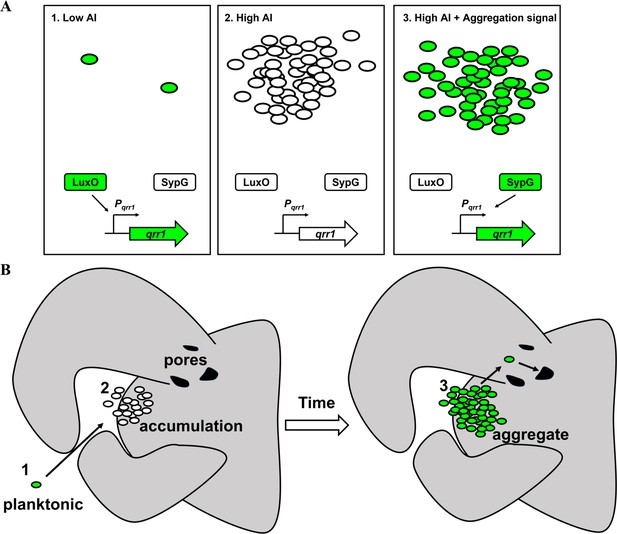
Model of dual bacterial enhancer binding protein (bEBP) control over Qrr1 expression in V. fischeri.
(A) Left, cells in an environment with low autoinducer concentration, for example, low cell density, will express Qrr1 by activating LuxO through the quorum-sensing pathway. Middle, cells in an environment with high autoinducer concentration, for example, high cell density, will have low Qrr1 levels due to the inactive state of LuxO. Right, even under conditions of high autoinducer concentration, expression of Qrr1 can occur if SypG is activated by the aggregation pathway. (B) Model of initial entry of V. fischeri into the light organ. Planktonic cells within the environment express Qrr1 due to low autoinducer levels (panel A, box 1). Motion of the cilia associated with the appendages sweep bacteria into a stagnant zone, where they locally accumulate (panel A, box 2), which has the potential to lower Qrr1 expression. Within a few hours, the cells have formed aggregates that depend on SypG (panel A, box 3), which induces Qrr1 expression to prime cells for entry into the pores.
Tables
LuxO and SypG homologs in Fischeri clade.
Taxon | Strain | LuxO homolog | Accession | SypG homolog | Accession | Identity | Similarity |
---|---|---|---|---|---|---|---|
V. fischeri | ES114 | WP_011261589.1 | NC_006840.2 | WP_011263835.1 | NC_006841.2 | 243/502 (48.41%) | 326/502 (64.94%) |
A. salmonicida | LFI1238 | WP_173362130.1 | NC_011312.1 | WP_044583634.1 | NC_011313.1 | 236/504 (46.83%) | 320/504 (63.49%) |
A. sifiae | NBRC 105001 | WP_172794763.1 | NZ_MSCP01000001.1 | WP_105064188.1 | NZ_MSCP01000002.1 | 238/495 (48.08%) | 316/495 (63.84%) |
A. wodanis | AWOD1 | CED71013.1 | LN554846.1 | CED57805.1 | LN554847.1 | 238/494 (48.18%) | 321/494 (64.98%) |
A. logei | 1S159 | WP_175365415.1 | NZ_MAJU01000008.1 | WP_065611272.1 | NZ_MAJU01000009.1 | 238/504 (47.22%) | 319/504 (63.29%) |
LuxO and SypG homologs in Vibrionaceae clades.
Clade | Taxon | LuxO homolog* | Accession | SypG homolog† | Accession | Identity‡ | Similarity‡ |
---|---|---|---|---|---|---|---|
Salinivibrio-Grimontia-Enterovibrio§ | G. hollisae | WP_005503370.1 | NZ_CP014056 | N.D. | — | N.A. | N.A. |
Rosenbergii | P. lutimaris | WP_107348500.1 | NZ_SNZO01000002 | N.D. | — | N.A. | N.A |
Profundum | P. profundum | WP_065814467.1 | NC_006370 | N.D. | — | N.A | N.A |
Damselae | Photobacterium damselae subsp. piscicida | WP_086957069.1 | NZ_AP018045 | N.D. | — | N.A | N.A |
Phosphoreum | P. phosphoreum | WP_045027808.1 | NZ_MSCQ01000001 | WP_105026695.1 | NZ_MSCQ01000001 | 237/499 (47.49%) | 310/499 (62.93%) |
Fischeri | V. fischeri | WP_011261589.1 | NC_006840 | WP_011263835.1 | NC_006841 | 243/502 (48.41%) | 326/502 (64.94%) |
Anguillarum | V. anguillarum | WP_026028983.1 | NC_022223 | N.D. | — | N.A | N.A |
Rumoiensis | V. rumoiensis | N.D. | — | N.D. | — | N.A | N.A |
Vulnificus | V. vulnificus | WP_011149911.1 | NC_014965 | WP_013571858.1 | NC_014965 | 247/508 (48.62%) | 320/508 (62.99%) |
Diazotrophicus | V. diazotrophicus | WP_042486207.1 | NZ_POSL01000002 | N.D. | — | N.A | N.A |
Gazogenes | V. gazogenes | WP_021019492.1 | NZ_CP018835 | N.D. | — | N.A | N.A |
Porteresiae | V. tritonius | WP_068714228.1 | NZ_AP014635 | N.D. | — | N.A | N.A |
Cholerae | V. cholerae | WP_001888250.1 | NC_002505 | N.D. | — | N.A | N.A |
Halioticoli | V. breoganii | WP_065209630.1 | NZ_CP016177 | WP_065210697.1 | NZ_CP016178 | 228/513 (44.44%) | 298/513 (57.70%) |
Splendidus | V. splendidus | WP_004734031.1 | NZ_CP031055 | WP_065205220.1 | NZ_CP031055 | 237/511 (46.38%) | 314/511 (61.45%) |
Pectenicida | V. pectenicida | WP_125320437.1 | NZ_RSFA01000020 | WP_125322971.1 | NZ_RSFA01000107 | 237/501 (47.31%) | 321/501 (64.07%) |
Scopthalmi | V. ponticus | WP_075650093.1 | NZ_AP019657 | WP_075649540.1 | NZ_AP019657 | 229/506 (45.26%) | 319/506 (63.04%) |
Nereis | V. nereis | WP_061781622.1 | NZ_BCUD01000001 | N.D. | — | N.A | N.A |
Orientalis | V. tubiashii | WP_038550519.1 | NZ_CP009354 | WP_004748949.1 | NZ_CP009354 | 242/497 (48.69%) | 319/497 (64.19%) |
Coralliilyticus | V. coralliilyticus | WP_019275536.1 | NZ_CP048693 | WP_021455926.1 | NZ_CP048693 | 242/503 (48.11%) | 322/503 (64.02%) |
Harveyi | V. harveyi | WP_005444697.1 | NZ_CP009467 | WP_050907635.1 | NZ_CP009467 | 244/522 (46.74%) | 320/522 (61.30%) |
Nigripulchritudo | V. nigripulchritudo | WP_022603175.1 | NC_022528 | WP_022550524.1 | NC_022528 | 247/508 (48.62%) | 331/508 (65.16%) |
Mediterranei | V. mediterranei | WP_062462808.1 | NZ_CP018308 | WP_088875891.1 | NZ_CP018308 | 236/503 (46.92%) | 318/503 (63.22%) |
-
*
N.D. (not detected) indicates that the top hit from BLAST was a bEBP other than LuxO.
-
†
N.D. (not detected) indicates that the top hit from BLAST was a bEBP other than SypG.
-
‡
N.A. (not applicable) due to SypG homolog not detected.
-
§
The Salinivibrio-Grimontia-Enterovibrio group is ancestrally related to the Vibrionaceae family and is included as an outgroup in this analysis.
Strains and plasmids used in this study.
Strain name | Genotype | Reference |
---|---|---|
ES114 | Wild-type V. fischeri | Mandel et al., 2008 |
DRO22 | ES114 Tn5::binK | This work |
MJM2481 | ES114 ΔbinK Tn7::erm | This work |
TIM303 | ES114 Tn7::(Pqrr1::gfp erm) | Miyashiro et al., 2010 |
TIM313 | ES114 Tn7::erm | Miyashiro et al., 2010 |
TIM412 | ES114 ΔbinK Tn7::(binK erm) | This work |
MJM2251 | ES114 ΔbinK | Brooks and Mandel, 2016 |
KRG004 | ES114 ΔrpoN | This work |
KRG011 | ES114 ΔbinK ΔrpoN | This work |
EDR009 | ES114 ΔbinK ΔluxO | This work |
EDR013 | ES114 ΔbinK ΔluxO ΔsypG | This work |
EDR014 | ES114 ΔbinK ΔsypG | This work |
MJM4982 | ES114 sypG(D53E) | This work |
MJM4983 | ES114 ΔbinK sypG(D53E) | This work |
EDS008 | ES114 ΔluxO ΔsypG Tn7::erm | This work |
EDS010 | ES114 ΔluxO ΔsypG Tn7::(lacIq Ptrc::sypG erm) | This work |
EDS015 | ES114 ΔsypG Tn7::(Pqrr1::gfp erm) | This work |
SSC009 | ES114 ΔsypK Tn7::(Pqrr1::gfp erm) | This work |
JHK007 | ES114 ΔainS ΔluxIR Plux-luxCDABEG | Kimbrough and Stabb, 2013 |
LFI1238 | Wild-type Aliivibrio salmonicida | Hjerde et al., 2008 |
EDS021 | ES114 ΔluxO ΔsypG Tn7::(lacIq Ptrc-sypGAS erm) | This work |
MJM2255 | ES114 rscS* ΔbinK | Brooks and Mandel, 2016 |
MJM1198 | MJM1100 rscS* | Singh et al., 2015 |
EDR010 | ES114 ΔbinK Δqrr1 | This work |
TIM305 | ES114 Δqrr1 | Miyashiro et al., 2010 |
SSC005 | ES114 Δqrr1 Tn7::erm | This work |
TIM306 | ES114 ΔluxO | Miyashiro et al., 2010 |
TIM311 | ES114 ΔluxO Tn7::erm | Miyashiro et al., 2010 |
KRG016 | ES114 ΔainS ΔluxIR Tn7::(Ptrc::gfp erm) | This work |
KRG018 | ES114 Δqrr1 Tn7::Pqrr1::qrr1 erm | This work |
KRG019 | ES114 Δqrr1 Tn7::Pqrr1(G−131T)::qrr1 erm | This work |
KRG021 | ES114 Δqrr1 Tn7::erm | This work |
Plasmid name | Relevant genotype | Source |
pVSV105 | R6Kori ori(pES213) RP4 oriT cat | Dunn et al., 2006 |
pTM267 | pVSV105 kan-gfp PtetA-mCherry | Miyashiro et al., 2010 |
pTM268 | pVSV105 Pqrr1-gfp PtetA-mCherry | Miyashiro et al., 2010 |
pSCV38 | pVSV105 PtetA-yfp PtetA-mCherry | Verma and Miyashiro, 2016 |
pYS112 | pVSV105 PproD-cfp PtetA-mCherry | Sun et al., 2016 |
pEDR003 | Region [(−373)–(+5)] of Pqrr1 cloned upstream of gfp reporter in pTM267 | This work |
pEDR011 | Region [(−357)–(+5)] of Pqrr1 cloned upstream of gfp reporter | This work |
pEDR012 | Region [(−262)–(+5)] of Pqrr1 cloned upstream of gfp reporter | This work |
pEDR006 | Region [(−209)–(+5)] of Pqrr1 cloned upstream of gfp reporter | This work |
pEDR009 | Region [(−106)–(+5)] of Pqrr1 cloned upstream of gfp reporter | This work |
pEDR008 | Region [(−60)–(+5)] of Pqrr1 cloned upstream of gfp reporter | This work |
pEDS007 | Region [(−373)–(+5)] of Pqrr1 with G−97T substitution cloned upstream of gfp reporter | This work |
pEDS008 | Region [(−373)–(+5)] of Pqrr1 with C−96A substitution cloned upstream of gfp reporter | This work |
pEDS009 | Region [(−373)–(+5)] of Pqrr1 with A−95C substitution cloned upstream of gfp reporter | This work |
pEDS004 | Region [(−373)–(+5)] of Pqrr1 with G−131T substitution cloned upstream of gfp reporter | This work |
pEDS005 | Region [(−373)–(+5)] of Pqrr1 with C−130A substitution cloned upstream of gfp reporter | This work |
pEDS006 | Region [(−373)–(+5)] of Pqrr1 with A−129C substitution cloned upstream of gfp reporter | This work |
pTM235 | pEVS79 ΔluxO | Miyashiro et al., 2010 |
pTM238 | pEVS79 Δqrr1 | Miyashiro et al., 2010 |
pDAT05 | pEVS79 sypG(D53E) | Ludvik et al., 2021 |
pEDR007 | pEVS79 ΔsypG | This work |
pEVS79 | pBC SK (+) oriT cat | Stabb and Ruby, 2002 |
pEVS104 | Stabb and Ruby, 2002 | |
pEVS107 | R6Kori oriT mini-Tn7 mob erm kan | McCann et al., 2003 |
pTn7BinK | pEVS107 binK | Brooks and Mandel, 2016 |
pTM239 | pEVS107 Pqrr1-gfp erm | Miyashiro et al., 2014 |
pAGC003 | pEVS107 lacIq Ptrc-sypGAs | This work |
pAGC004 | pTM267 Pqrr1AS-gfp | This work |
pKG11 | pKV69 rscS* | Yip et al., 2006 |
pKV69 | Mobilizable vector; tetR cat | Visick and Skoufos, 2001 |
pKRG040 | pEVS107 Pqrr1::qrr1 | This work |
pKRG041 | pEVS107 Pqrr1(G-131T)::qrr1 | This work |
pVF_A1020P | pTM267 PsypA::gfp | This work |
pVF_A1035P | pTM267 PsypP::gfp | This work |
Primers used in this study.
Primers | 5′ → 3′ |
---|---|
ΔsypG | |
sypG-del-XbaI-l1 | CGGTCTAGATGTGGTGGATTCTTTTCCATAAATGCC |
sypG-del-XbaI-u1 | GGCTCTAGAGTTAAGCCCGTCAACACTCT |
sypF-KpnI-u1 | GGTACCGTTCTGGTTTAGGGTTAGCTATTTGTCA |
sypH-SacI-l1 | GAGCTCCAGACAATAAAGAGGGGATGATAGC |
ΔrpoN | |
ES_rpoN Del Up F | CCTCAAGAAGCTTCTATTTTTAGAA |
ES_rpoN Del Up R | TAGGCGGCCGCACTAAGTATGGTATTTAGCGATACCTTTTGTACATT |
ES_rpoN Del Down F | GGATAGGCCTAGAAGGCCATGGTTAATGAAAAGGAAGTGTTATGCAA |
ES_rpoN Del Down R | GATAGCTATCCCATTACCTATACCA |
sypGD53E sequencing | |
DAT_095 sypG fwd | CTACAGCAAGCCAGAAATGAAGCAG |
DAT_096 sypG rev | GGGTGCCTTTTGATTGAATTAAGTTC |
pEDS003 | |
sypG-pTrc-KpnI-u1 | GGTACCTTCGCTAGGTAAAACAGGATGTTA |
sypG-pTrc-BsrGI-l1 | GGTGTACAGTAACCATATTTCATCATTCCGAT |
pAGC003 | |
AS-KpnI-SypG-U1 | GGTACCTGCACAAGGCTTCACTA |
AS-BsrGI-SypG-L1 | TGTACACAAAAGCCATACCTCAAAAG |
pEDR003 | |
qrr1-prom-XmaI-u2 | GGCCCGGGCAGCCAACACATCAAAACCTGTCA |
qrr1-prom-XbaI-l2 | GGTCTAGAACTAGTGGTCAATATACCTATTGCAGGGAG |
pEDR006 | |
qrr1-prom-XmaI-u3 | GGCCCGGGGTATCATCAAATCCAACTTGAGGG |
qrr1-prom-XbaI-l2 | GGTCTAGAACTAGTGGTCAATATACCTATTGCAGGGAG |
pEDR008 | |
qrr1-XmaI-reg1-u1 | GCGCCCGGGGGCTTATTTAGCTTATTTTTACG |
gfp-XhoI-l1 | TACTCGAGTTTGTGTCCGAGAATGTTTCCATC |
pEDR009 | |
qrr1-XmaI-reg2-u1 | CCGCCCGGGACGCAATTTGCAAAATGC |
gfp-XhoI-l1 | TACTCGAGTTTGTGTCCGAGAATGTTTCCATC |
pEDR010 | |
qrr1-XmaI-reg5-u1 | GGCCCCGGGCAATATCAAAACCTAACGGG |
gfp-XhoI-l1 | TACTCGAGTTTGTGTCCGAGAATGTTTCCATC |
pEDR011 | |
qrr1-XmaI-reg7-u1 | GGCCCCGGGACCTGTCATGTCAGGC |
gfp-XhoI-l1 | TACTCGAGTTTGTGTCCGAGAATGTTTCCATC |
pEDR012 | |
qrr1-XmaI-reg4-u1 | CCGCCCGGGGCAGTATCTTCTACCATTAATAAA |
gfp-XhoI-l1 | TACTCGAGTTTGTGTCCGAGAATGTTTCCATC |
pEDS004, pKRG041 | |
qrr1-prom-SDM-G243T-u1 | TAAAAATGCGGTTGATATTTTCATTATGCAATCAGGATTCG |
qrr1-prom-SDM-G243T-l1 | CGAATCCTGATTGCATAATGAAAATATCAACCGCATTTTTA |
pEDS005 | |
qrr1-prom-SDM-C244A-u1 | AAAAATGCGGTTGATATTTGAATTATGCAATCAGGATTCGC |
qrr1-prom-SDM-C244A-l1 | GCGAATCCTGATTGCATAATTCAAATATCAACCGCATTTTT |
pEDS006 | |
qrr1-prom-SDM-A245C-u1 | AAAATGCGGTTGATATTTGCCTTATGCAATCAGGATTCGCA |
qrr1-prom-SDM-A245C-l1 | TGCGAATCCTGATTGCATAAGGCAAATATCAACCGCATTTT |
pEDS007 | |
qrr1prom-mut_G277T_u1 | GGATTCGCAAAACGCAATTTTCAAAATGCAAAAAAGGATG |
qrr1prom-mut_G277T_l1 | CATCCTTTTTTGCATTTTGAAAATTGCGTTTTGCGAATCC |
pEDS008 | |
qrr1prom-mut_G278A_u1 | GATTCGCAAAACGCAATTTGAAAAATGCAAAAAAGGATGAC |
qrr1prom-mut_G278A_l1 | GTCATCCTTTTTTGCATTTTTCAAATTGCGTTTTGCGAATC |
pEDS009 | |
qrr1prom-mut_G279C_u1 | CGCAAAACGCAATTTGCCAAATGCAAAAAAGGATG |
qrr1prom-mut_G279C_l1 | CATCCTTTTTTGCATTTGGCAAATTGCGTTTTGCG |
pKRG040 | |
Qrr1-SpeI-u1 | CCGGACTAGTTAGTTAGTTATTGATTTTAA |
Qrr1-KpnI-l1 | CCGGGGTACCCAGCCAACACATCAAAACCT |
pAGC003 | |
AS-KpnI-SypG-U1 | GGTACCTGCACAAGGCTTCACTA |
AS-BsrGI-SypG-L1 | TGTACACAAAAGCCATACCTCAAAAG |
pAGC004 | |
AS-Qrr1-XmaI-U1 | CCCGGGGTCCAGTCATATCCGGCAAGC |
AS-Qrr1-XbaI-L1 | TCTAGAGGTCACTATACATATAGCAGAG |